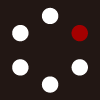
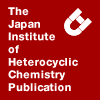
HETEROCYCLES
An International Journal for Reviews and Communications in Heterocyclic ChemistryWeb Edition ISSN: 1881-0942
Published online by The Japan Institute of Heterocyclic Chemistry
e-Journal
Full Text HTML
Received, 18th December, 2008, Accepted, 22nd January, 2009, Published online, 26th January, 2009.
DOI: 10.3987/COM-08-11632
■ Studies on the Synthesis and Crystal Structure of N-(1-Naphthyl)succinimide
Ying Wang, Hailong Yang, Hexian Li, and Guochang Wang*
Institute of Polymer Chemistry, Nankai University, 94 Weijin Road, Tianjin, 300071, China
Abstract
A modified two-step procedure for synthesis of N-(1-naphthyl)succinimide (NaS) was developed, and its single crystal was prepared for the first time. The molecular and crystal structures were characterized by XRD, FT-IR, 1H NMR, DSC, etc. It has been found that the melting point (159-160.5 °C) of our product is ca 10 °C higher than the documented values, while the characteristic infrared absorption band of carbonyl group splits into two peaks (1705cm-1/1779cm-1) rather than one as reported in the references. These discrepancies were further examined by X-ray crystallographic analysis. Meanwhile, photophysical spectroscopy was found to be powerful to study the molecular structure and crystal morphology of organic compound.N-(1-Naphthyl)succinimide is one of the important N-substituted succinimide derivatives and has been widely used as model compound for some physiological processes1 and the fluorescence probe.2 However, there has been only a few of papers reporting the synthesis of NaS, in which two main procedures were adopted, i.e., two-step and one-step methods. In the two-step method, 1-naphthylamine was first reacted with succinimide to yield N-(1-naphthyl)succinamic acid, and it was then dehydrated by acetic anhydride to get the final product. Zhen-chu Chen et al.3 obtained NaS through a one-step reaction that the mixture of succinimide and 1-naphthylamine was heated with the promotion of ionic liquid [Bmim][PF6]. The products from both of the above mentioned methods was reported to have a melting point in the range of 147-149 °C, and a unique vibration peak at about 1700 cm-1 for the carbonyl stretching. Recently, we synthesized NaS compound by employing a modified two-step method. After careful purification and characterization, we found that the structural identification data of our product were quite discrepant from those of the reported products. Our product showed a melting point of 159-160.5 °C and a double-splitting band of 1705/1779 cm-1 for the carbonyl vibration in the infrared spectrum, and both of our results were well reproducible within the experimental error. For further structural identification, we grew the single crystal of our product, which clearly confirmed that the molecular chemical structure of our product completely coincided with the theoretical formula of NaS. As another important finding of this study, the photophysical spectroscopy performed extremely high sensitivity and resolution in characterization of molecular electronic structure and crystal morphology.
RESULTS AND DISCUSSION
In following the two-step synthetic procedure, we found that the final product was very difficult to get highly purified, and it often exhibited some uncertainty in physical properties. For this, we made two modifications on the procedure: (1) In the second step of dehydrolysis, we used pure acetic anhydride instead of acetic anhydride solution used in the reference to increase the concentration of dehydrating agent and improve the conversion of N-(1-naphyl)succinamic acid to N-(1-naphthyl)succinimide; (2) An additional washing process with K2CO3 solution was applied at the end of the second step for thoroughly removing the acidic impurity. By using this modified two-step procedure, we were able to make the synthesis well reproducible and the final product achieved with high purity. The results of HPLC show
that our product is clearly distinguished from 1-naphthylamine and N-(1-naphthyl)succinamic acid in retention time and the purity is estimated to be nearly 100%. However, the melting point measurement showed quite a large distinction between our product (159-160.5 °C) and the documented product (147-149 °C). For confirming the exact structure of our product, we further studied the molecular chemical structure and the single crystal morphology.
Figure 1a is the infrared spectrum of our product. As can be seen, the most striking feature is that the absorption band for carbonyl-stretching vibration splits into two peaks (1705 cm-1/ 1779 cm-1). The succinimide group has a formal plane of symmetry, and two frequencies would be expected corresponding to symmetry and antisymmetry vibrations of the two carbonyl groups in phase and out of phase (Figure 1b),4 in which the antisymmetry vibration leads to more stable resonance structure of the succinimide group.5 This splitting feature of the carbonyl vibration is typical of succinimide group. However, the infrared data reported in the references all show a single peak at frequency of about 1700 cm-1, which provide further evidence for the discrepancy between the documented and our products.
In Figure 2, the UV-Vis absorption and fluorescence emission spectra of naphthalene, 1-naphthylamine and NaS in solution are compared. It can be seen clearly that the absorption and emission spectra of NaS are quite different from those of 1-naphthylamine, but very close to those of the unsubstituted naphthalene ring except for a slight red shift and a little loss of resolution. In 1-naphthylamine, the induction and conjugation effects of the amino group break down the symmetry of electron cloud distribution and further enlarge the π-electron delocalization, which underlie the large red shift and the complete loss of the fine structure of the UV-Vis adsorption and fluorescence spectra. In NaS molecule, however, the electronic interaction between the succinimide and naphthyl rings are sufficiently reduced due to the carbonyl induction and steric restriction on coplanar conformation of the two rings, so that the naphthyl ring in NaS molecule has a electronic structure very similar to that of the pure naphthalene ring. Here we see that the photophysical spectroscopy provides a very sensitive method in study of molecular electronic structure.
The X-ray crystallographic analysis was performed based on a single crystal sample grown from a solution of NaS in tetrahydronfuran. The ORTEP drawing (Figure 3) provides the exact molecular structure of NaS, in which a five-membered and a naphthyl ring lie on two planes with a dihedral angle of 107.9 °, which contributes to the stability of the molecule by reducing the steric hindrance. The bond angles of C(1)-N(1)-C(11) [123.35(15)], C(1)-N(1)-C(14) [123.27(16)] are nearly equal but different from that of C(11)-N(1)-C(14) [113.04(16)], indicating the effect of the cyclic tensility on the sp2 hybridization of the nitrogen atom. The shortest intramolecular distance between O atoms and H atoms on naphthyl ring is 2.7832 Å[O(2)…H(9)]. It oversteps the effective range to form a hydrogen bond. The morphology of NaS single crystal is found to be of orthorhombic system with a space group of P212121 (Figure 4). In the cell packing pattern of the single crystal, each unit cell is seen to contain four inequivalent NaS molecules and two types of hydrogen bond formed via intermolecular interaction as labeled with broken lines. When viewed along the a-axis, each molecule in the crystal lattice interacts with another symmetry related molecule with the aid of these intermolecular hydrogen bonds. The hydrogen bonds form a zigzag chain, which runs down along the translation axis b. The shortest distance between two neighboring naphthyl rings is greater than 6.0Å, which is beyond the effective distance for π-π interaction.
For further exploring the origin of the discrepancy, it is necessary to compare the structures and properties of the single crystal with microcrystal. Figure 5a shows the standard DSC melting scan of the single crystal sample. A single melting peak with a maximum of 160.5 °C is observed with melting enthalpy of 79J/g. These data are almost identical with those for the microcrystal sample (158.9 °C/80J·g-1, Figure 5b). This result manifests that both microcrystal and single crystal are of the same morphology except for a slight difference in the DSC data, which characterizes more surface defects with the microcrystal sample. Moreover, the peak values of DSC are found to be very close to those determined by a melting point apparatus.
Figure 6b shows the typical transmitted XRD signal of microcrystal, which is quite consistent with the result calculated from single crystal reflections (Figure 6a). Similarly to DSC measurement, the slight broadening peaks and intensity ratio variation of the peaks in the XRD pattern of the microcrystal are due to its surface defects, for in theory the half-width of the peak is inversely proportional to the size of crystal grains. Obviously, both the results of DSC and XRD suggest that the microcrystal and single crystal belong to the same morphology. However, the fluorescence measurements reveal more detailed feature of the surface structure of the microcrystal.
The fluorescence emission spectrum of NaS single crystal basically retains the spectral feature of its dilute solution (Figure 7a). However, the absorption maximum in the excitation spectrum shifts from 280 nm to 315nm (Figure 7b), which reflects the influence of crystal environmental anisotropy, hydrogen bonding and dipole-dipole interactions. Furthermore, as indicated by the results of single crystal X-ray diffraction, there are four translationally inequivalent molecules per unit cell, and which will correspondingly give a rise to quadruple Davydov Splitting for each excited electronic state of the molecule,6 and cause further spectral shift. Basically, the microcrystal behaves similarly to the single crystal in monomeric absorption and emission properties, which suggests that the NaS molecules experience similar microenviroments in single and microcrystals, i.e., both the crystals are of the same morphology. Nevertheless, some distinctive feature still can be seen with the microcrystal spectra, particularly an extraneous weak absorption band peaked at 344 nm appears in the excitation spectrum with λem=420 nm, and correspondingly a weak redshift emission band centered at ca 400nm is observed with λex=344 nm. This result suggests some new fluorescence species, which we assign to ground-state association of the NaS molecules constrained in the surface defects rather than excimers, for the new and distinctive absorption band appears relatively to the monomer absorption of the NaS molecules.
In conclusion, first, we have developed a modified two-step method for NaS synthesis, which yields product with the exact theoretically formulated structure; second, we have, for the first time, carried out the preparation and the X-ray crystallographic analysis of NaS single crystal, which is confirmed to be of the same orthorhombic crystal system as the microcrystal, except that the latter features molecular ground-state association in surface defects; finally in a NaS molecule, the carbonyl vibration is doubly splitted, and the naphthyl ring much resembles an unsubstituted naphthalene ring in electronic structure. What is worth emphasizing here is the powerful sensitivity of photophysical spectroscopy in getting insight into more detailed structure of both molecules and crystals.
EXPERIMENTAL
Synthesis of Nas: Succinic anhydride (0.02 mol) was added to a stirring solution of 1-naphthylamine (0.02 mol) dissolved in toluene (100 mL), and the mixture was then stirred at 80 °C for ca 1 h until white precipitate was formed. After filtration and washing with toluene, the precipitate was dried in an oven at 60 °C to give crude N-(1-naphthyl)succinamic acid (I) in 94% yield. Compound I (0.018 mol) was then added to a 100 mL solution of acetic anhydride containing 0.46 g sodium acetate (0.006 mol). The resultant suspension was heated at 100 °C for 1 h under stirring. The reaction mixture was cooled to rt in a cold water bath and then poured into 1 L ice water. The raw product of N-(1-naphthyl)succinimide (II) was separated by suction filtration, washed with 300 mL 10% aqueous potassium carbonate solution, and finally recrystallized from absolute EtOH for 3 times. After drying in an oven at 60 °C, the white microcrystal was gained. Yield 38%; Anal. Calcd. (%) for C14H11NO2: C 74.65’, H 4.92’, N 6.22’. Found (%): C 74.60, H 5.01, N 6.15. (sample dried in vacuum for 12 h); IR: 1705/1779 (ν (CO))cm-1; 1H NMR (CDCl3, 400MHz, 298K) δ=3.01-3.12 (m, 4H; CH2CH2), 7.27-7.98 (m, 7H; aryl-H); mp 159-160.5 °C
Crystal data for NaS: C14H11NO2; orthorhombic; space group P212121; a=8.735(4), b=9.807(4), c=13.729(5) Å, V=1176.1(8) Å3; Z=4; T=293K; M=225.24; μ(Moκα)=0.086mm-1, 6652 reflections measured, 2369 independent reflections. Final Rl=0.0390, ωR2 (all data)=0.0957. CCDC-267586 (NaS) contains the supplementary crystallographic data for this paper. These data can be obtained free of charge from The Cambridge Crystallographic Data Centre via www.ccdc.cam.ac.uk/data_request/cif.
References
1. J. L. Hubbard, J. M. Carl III, and G. D. Anderson, J. Heterocycl. Chem., 1992, 29, 719; CrossRef A. Gossauer, W. Hirsch, and R. Kutschan, Angew. Chem., Int. Ed. Engl., 1976, 15, 626; CrossRef H. D. King and M. Gene, Tetrahedron Lett., 2002, 43, 1987; CrossRef B. N. Manjula and A. Tsai, Bioconjugate Chem., 2003, 14, 464. CrossRef
2. J. Duhamel, A. Yekta, and M. A. Winnik, J. Phys. Chem., 1993, 97, 13708. CrossRef
3. Z.-G. Le, Z.-C. Chen, Y. Hu, and Q.-G. Zheng, Synthesis, 2004, 995. CrossRef
4. X.-J. Zheng, J.-Y. Zhuang, and L.-P. Jin, J. Mol. Struct., 2001, 595, 201. CrossRef
5. P. T. Mckitterick and J. E. Katon, Appl. Spectrosc., 1900, 44, 812. CrossRef
6. J. B. Birks, 'Photophysics of Aromatic Molecules', Wiley- Interscience Press, Inc., London, 1970.