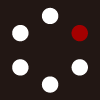
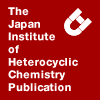
HETEROCYCLES
An International Journal for Reviews and Communications in Heterocyclic ChemistryWeb Edition ISSN: 1881-0942
Published online by The Japan Institute of Heterocyclic Chemistry
e-Journal
Full Text HTML
Received, 27th January, 2009, Accepted, 5th March, 2009, Published online, 6th March, 2009.
DOI: 10.3987/COM-09-11666
■ Synthesis and Properties of 9,9’-Spirobifluorene-Based Heterocycles
Jong Myoung Chea and Yurngdong Jahng*
College of Pharmacy, Yeungnam University, Kyongsan 712-749, Korea
Abstract
A series of 9,9’-spirobifluorene-derived N-heterocycles were prepared by Frieländer reaction of 2-acetyl-9,9’-spirobifluorene with a series of ortho-aminoaldehydes in 74-88% yields. Spectral properties and abilities to form Ru(II) complexes were examined.The 9,9’-spirobifluorene (1) has been attractive due to the characteristic structure since its discovery.1 After the first examples employed as a motif in the area of molecular recognition were reported by the groups of Prelog,2 Diederich,3 and others,4 9,9’-spirobifluorene and related compounds especially oligomers or polymers have been used in a wide range of applications in molecular electronics,5 macromolecular chemistry,6 light emitting devices,7 and others.8
Studies of monomeric 9,9’-spirobifluorenes especially 9,9’-spirobifluorene-derived N-heterocycles are very limited. Although a few poly(pyridine-derived-heteroaryl)-9,9’-spirobifluorenes such as 2,2’-di(1,10-phenanthrol-2-yl)-9,9’-spirobifluorene,9 2,2’-di(benzo[h]quinol-2-yl)-9,9’-spirobifluorene,10 and 2,2’,7,7’-tetra(benzo[h]quinol-2-yl)-9,9’-spirobifluorene,10 have been reported, 2-(4-phenylquinol- 2-yl)-9.9’-spirobifluorene11 is, to the best of our knowledge, the only mono-substituted pyridine-derived heteroaryl-9,9’-spirobifluorene reported so far. In addition, systematic approaches either for the preparation or for the examination of their properties of the systems have not been pursued as yet.
Our interest12 in the preparation and properties of polydentates as well as their metal complexes spurred to prepare a series of 9,9’-spirobifluorene-derived N-heterocycles and to study their properties.
The prerequisite 9,9’-spirobifluorene (1) was readily prepared in three steps from relatively cheap 2-bromobiphenyl by a modification of previously reported method.1,13 The Grignard reaction of 2-bromobiphenyl with commercially available 9-fluorenone gave 9-(2’-biphenyl)-9-fluorenol, which was subjected to acid-catalyzed cyclization to afford 1 in an overall yield of 52%. The Friedel-Craft acylation of 1 with an equimolar of acetyl chloride afforded 2-acetyl-9,9’-spirobifluorene (2)14 in 11% yield along with 2,2’-diacetyl-9,9’-spirobifluorene as a major product (51%), which were readily separable by column chromatography and fully characterized.
Synthesis of the 9,9’-spirobifluorene-derived heterocycles was straight-forwards as shown in Scheme 1. The Friedländer condensation of 2-acetyl-9,9’-spirobifluorene (2) with a series of o-aminoaldehydes (3)15 afforded the corresponding 9,9’-spirobifluorene-derived heteroaromatics (4) in 74-88% yields.
Some proton resonances of the products were characteristic enough to afford probes for the structure suggested. The protons adjacent to nitrogen such as H7” of 4b and H9” of 4d are the ones of characteristic chemical shifts (δ 9.02 and 9.15, respectively) with relatively small 3J coupling constant (4.3 Hz).16 In addition, the resonances of H1 of 9,9’-spirobifluorene moiety generally appeared in the region of δ 7.47-7.80 with a characteristic meta-coupling constant (4J = 1.4-1.8 Hz). The protons H8, H1’ and H8’ of the 9,9’-spirobifluorene moiety are the most upfield-shifted ones due to the anisotropic effect of the neighboring two phenyl groups, thus are resonanced in the range of δ 6.80-6.71. Additionally, the protons at the peri-position of 4a and 4e (H8” of 4a, H11” of 4e) were resonanced at δ 8.67 and 8.61, respectively, and H10” of benzo[h]quinoline moiety of 4c was resonanced at δ 9.34 as a doublet of doublet (3J = 8.8 Hz and 4J = 1.8 Hz), which experienced anisotropic effect of neighboring pyridine as well as electronic effect of lone pair electrons on N1” most seriously.
UV absorption spectra of 9,9’-spirobifluorene (1) and its derivatives 4 were taken from 95% EtOH (1.6 x 10-5 M) and are summarized in Table 1. Three major absorption maxima originated from the π-π* transition were observed in the regions of 221-226, 253-274, 296-315, and 330-370 nm. The absorptions in the range of 330-370 nm were not observed in the parent compound 9,9’-spirobifluorene, thus such absorptions were from π-π* transition of the heterocyclic rings of the system. The absorption maximum is highly dependent on the nature of the heterocycles attached to 9,9’-spirobifluorene. UV spectrum of 4e showed two characteristic features. Annulation of an additional benzene ring on 4d not only led bathochromic shift of the absorptions at 233 and 264 nm to 254 and 275 nm, respectively, and increased corresponding intensity, but also increased the absorption intensity for the absorption at 332 nm.
To examine the ability to form metal complexes, a reaction of ligand 4d with RuCl3·3H2O in refluxing 95% EtOH was pursued to afford Ru(4d)2Cl2 in 83% yield as dark brown solid. The steric congestion around the Ru(II) core induced by the two ligands chelated would not allow approaching of the third ligand for forming tris-complexes.
Although two diastereomers (5a and 5b) were possible in Ru(4d)2Cl2, 1H NMR of the complex showed only one set of spectrum in DMSO-d6. The resonance at δ 8.27 with characteristic 3J coupling constant (3J = 4.8 and 4J = 1.0 Hz)16 covered H9”, a sensitive probe for the structure of the complex, which was upfield-shifted by 0.88 ppm compared to that of the parent ligand (4d). In complex 5a, H9” experiences the shielding effect of neighboring ligand on the Ru core by lying towards the shielding region to lead 0.88 ppm upfield-shift while H9” in 5b lies towards deshielding region of Cl.12b,17 It should be noted that reaction of 4e with RuCl3·3H2O afforded a messy mixture of complexes which were not able either to separate nor to characterize. Steric hindrance induced by H11” of the ligand and/or free rotation through 2,2’-bond would prevent forming not only bis- but also tris-complexes as have been reported for 4’-(pyri-4’”-yl)-2,2’;6’,2”-terpyridine.18
In conclusion, a series of 9,9’-spirobifluorene-derived N-heterocycles were prepared by Frieländer reaction of 2-acetyl-9,9’-spirobifluorene with a series of ortho-aminoaldehydes. Studies on photophysical properties of the ligands and their metal complexes are in progress, which will be due in the future.
EXPERIMENTAL
Melting points were determined using a Fischer-Jones melting points apparatus and are not corrected. IR spectra were obtained using a Perkin-Elmer 1330 spectrophotometer. NMR spectra were obtained using a Bruker-250 spectrometer 250 MHz for 1H NMR and 62.5 MHz for 13C NMR and are reported as parts per million (ppm) from tetramethylsilane. The starting 9,9’-spirobifluorene (1)1,13 and o-aminoaldehydes (3)15 were prepared by previously reported methods. Electrospray ionization (ESI) mass spectrometry (MS) experiments were performed on a LCQ advantage-trap mass spectrometer (Thermo Finnigan, San Jose, CA, USA). Elemental analyses were taken on a Hewlett-Packard Model 185B elemental analyzer.
2-Acetyl-9,9’-spirobifluorene (2)
A solution of 9,9-spirobifluorene (5.0 g, 15.8 mmol) in dry CS2 (30 mL) was slowly added powdered AlCl3 (9.0 g) in portions (~10 min). To a stirring mixture resulted was added 1.24 g (15.8 mmol) of acetyl chloride in CS2 (20 mL). After 1 h reflux, the reaction mixture was poured to a mixture of ice (100 g) and 2N HCl (50 mL). The solid formed was collected and chromatographed on silica gel eluting with benzene:EtOAc (10:1). The early fractions [Rf = 0.26 (CH2Cl2:hexane = 4:9)] afforded 0.60 g (11%) of 2-acetyl-9,9’-spirobifluorene (4) (CHCl3:benzene): mp 225 oC (lit.,14 mp 225 oC). 1H NMR (CDCl3) δ 8.00 (dd, J = 8.0, 1.6 Hz, 1H, H3), 7.89 (d, J = 8.0 Hz, 1H, H4), 7.87(d, J = 8.0 Hz, 1H, H5), 7.83 (d, J = 8.0 Hz, 2H, H4’, H5’), 7.39 (td, J = 7.5, 1.2 Hz, 1H, H6), 7.35 (td, J = 7.5, 1.2 Hz, 2H, H3’, H6’), 7.30 (d, J = 1.8 Hz, 1H, H1), 7.15 (td, J = 7.5, 1.2 Hz, 1H, H7), 7.08 (td, J = 7.5, 1.2 Hz, 2H, H2’, H7’), 6.73 (d, J = 7.8 Hz, 1H, H8), 6.67 (d, J = 7.5 Hz, 2H, H1’, H8’), 2.43 (s, 3H). The latter fractions [Rf = 0.07 (CH2Cl2:hexane = 4:9)] afforded 1.60 g (51%) of 2,2’-diacetyl-9,9’-spirobifluorene.
2-(1”,8”-Naphthrid-2-yl)-9,9’-spirobifluorene (4b)
Yield (88%): mp 142 oC. 1H NMR (CDCl3) δ 9.02 (dd, J = 4.3, 2.0 Hz, 1H, H7”), 8.36 (dd, J = 8.3, 1.5 Hz, 1H, H5”), 8.09 (d, J = 8.5 Hz, 1H, H4”), 8.08 (dd, J = 8.0, 1.2 Hz, 1H, H3), 7.99 (d, J = 8.0 Hz, 1H, H4), 7.91-7.83 (m, 4H), 7.76 (d, J = 1.0 Hz, 1H, H1), 7.40-7.33 (m, 4H), 7.11 (td, J = 7.8, 1.2 Hz, 1H, H6), 7.08 (td, J = 7.8, 1.2 Hz, 2H, H3’ and H6’), 6.75 (d, J = 7.8 Hz, 2H, H4’ and H5’), 6.72 (d, J = 7.8 Hz, 1H, H5). 13C NMR (CDCl3) δ 160.00, 155.88, 153.68, 149.91, 148.30, 144.02, 141.95, 140.84, 138.04, 137.41, 136.65, 128.41, 127.93, 127.83, 127.77, 124.08, 124.04, 123.67, 121.58, 121.54, 120.46, 120.27, 120.17, 119.85, 66.05. MS (ESI) calcd for C33H21N2+ [M+H+]: 573. Found: 573. Anal. Calcd for C33H20N2·H2O C, 85.69; H, 4.79; N, 6.06. Found: C, 85.73, H, 4.74 N, 6.09.
2-(Benzo[h]quinol-2”-yl)-9,9’-spirobifluorene (4c)
Yield (79%): mp 132 oC. 1H NMR (CDCl3) δ 9.34 (dd, J = 8.8, 1.8 Hz, 1H, H10”), 8.57 (dd, J = 8.0, 1.5 Hz, 1H), 8.04 (dd, J = 8.0 Hz, 2H), 7.94-7.85 (m, 4H), 7.71-7.68 (m, 4H), 7.60 (d, J = 8.5 Hz, 1H), 7.48 (d, J = 1.3 Hz, H1), 7.40 (td, J = 7.5, 1.0 Hz, 1H, H6), 7.39 (td, J = 7.5, 1.0 Hz, 2H, H3’ and H6’), 7.13 (td, J = 7.5, 1.2 Hz, 1H, H7), 7.12 (td, J = 7.5, 1.0 Hz, 2H, H2’ and H7’), 6.81 (d, J = 8.0 Hz, 2H, H1’ and H8’), 6.72 (d, J = 8.0 Hz, 1H, H8). 13C NMR (CDCl3) δ 155.37, 149.57, 149.20, 148.59, 146.09, 142.99, 141.85, 141.21, 139.57, 136.24, 133.80, 131.66, 128.13, 128.06, 127.91, 127.84, 127.79 (two C’s), 127.30, 126.81, 125.00, 124.63, 124.21, 124.03, 122.78, 120.50, 120.34, 120.05, 119.10, 66.09. MS (ESI) calcd for C38H24N+ [M+H+]: 494. Found: 494. Anal. Calcd for C38H23N: C, 92.47; H, 4.70; N, 2.84. Found: C, 92.51; H, 4.68; N, 2.87.
2-(1”,10”-phenanthrol-2”-yl)-9,9’-spirobifluorene (4d)
Yield (76%): mp 351 oC. 1H NMR (CDCl3) δ 9.15 (dd, J = 4.3, 1.8 Hz, 1H, H9”), 8.55 (dd, J = 8.0, 1.8 Hz, 1H, H7”), 8.20 (dd, J = 8.3, 1.8 Hz, 1H, H3), 8.12 (d, J = 8.5 Hz, 1H, H4”), 8.03 (d, J = 8.0 Hz, 1H, H4), 7.91 (d, J = 7.5 Hz, 1H, H5), 7.86 (d, J = 7.5 Hz, 2H, H4’ and H5’), 7.81 (d, J = 8.5 Hz, 1H, H3”), 7.70 (AB quartet, 2H, H5” and H6”), 7.59 (dd, J = 8.0, 4.5 Hz, 1H, H8’), 7.43 (d, J = 1.3 Hz, 1H, H1), 7.38 (td, J = 7.5, 1.0 Hz, 1H, H6), 7.36 (td, J = 7.5, 1. Hz, 2H, H3’ and H6’), 7.10 (td, J = 7.5, 1.0 Hz, 3H, H7, H2’ and H7’), 6.78 (d, J = 7.5 Hz, 2H, H1’ and H8’), 6.72 (d, J = 7.5 Hz, 1H, H8). 13C NMR (CDCl3) δ 157.66, 150.26, 149.63, 148.91, 148.59 (two C’s), 146.24, 145.92, 143.08, 141.85, 141.16, 139.72, 136.51, 136.02, 128.95, 128.79, 128.08, 127.90, 127.73 (two C’s), 127.34, 126.28, 126.07, 124.24, 123.93, 123.14, 122.80, 121.08, 120.57, 120.39, 120.00, 66.07. MS (ESI) calcd for C37H23N2 [M+H+]: 495. Found: 495. Anal. Calcd for C37H22N2: C, 89.85; H, 4.48; N, 5.66. Found: C, 89.82; H, 4.51; N, 5.63.
2-(Benzo[b]-1,10-phenathrolin-2-yl)-9.9’-spirobifluorene (4e)
Yield (74 %): mp 332 °C. 1H NMR (CDCl3) δ 8.71 (s, 1H, H7”), 8.61 (dd, J = 8.0, 1.5 Hz, 1H, H11”), 8.55 (d, J = 8.3 Hz, 1H, H4”), 8.11 (d, J = 8.5 Hz, 1H, H3”), 8.07 (d, J = 8.0 Hz, 1H, H5), 8.03 (d, J = 8.5 Hz, 1H, H3), 7.94-7.78 (m, 6H), 7.63 (td, J = 8.0, 1.0 Hz, 1H, H10”), 7.62 (d, J = 8.5 Hz, 1H), 7.48 (d, J = 1.0 Hz, 1H, H1), 7.40 (td, J = 7.5, 1.0 Hz, 1H, H6), 7.37 (td, J = 8.0, 1.0 Hz, 2H, H3’ and H6’), 7.10 (td, J = 7.5, 1.0 Hz, 3H, H7, H2’ and H7’), 6.79 (d, J = 7.5 Hz, 2H, H1’ and H8’), 6.72 (d, J = 7.5 Hz, 1H, H8). ). 13C NMR (CDCl3) δ 157.36, 149.67, 149.09, 148.64 (two C’s), 148.43, 147.09, 146.63, 143.11, 141.91, 141.25, 139.76, 136.53, 131.16, 129.93, 128.72, 128.02, 127.93, 127.75 (two C’s), 127.56, 127.36, 127.08, 126.76, 125.76, 124.28, 123.99, 123.24, 121.43, 120.58, 120.42, 120.03, 66.13. MS (ESI) calcd for C41H25N2 [M+H+]: 545. Found: 545. Anal. Calcd for C41H24N2: C, 90.42; H, 4.44; N, 5.14. Found: C, 90.46; H, 4.42; N, 5.12.
Ru(4d)2Cl2
A mixture of 4d (494 mg, 1.0 mmol) and RuCl3·3H2O (80 mg, 0.31 mmol) in 95% EtOH was refluxed for 8 h. Resulting precipitate was filtered, washed with water and EtOH to give dark red solid: mp > 300 oC. 1H NMR (DMSO-d6) δ 8.90 (overlapped d, 4H, J = 7.5 Hz), 8.57 (t, 2H, J = 7.8 Hz), 8.27 (dd, 2H, J = 4.8, 1.0 Hz, H9”), 8.15-7.93 (m, 12H), 7.53 (d, 4H, J = 7.3 Hz), 7.42-7.33 (m, 6H), 7.21-7.04 (m, 6H), 6.95-6.91 (d, 4H, J = 9.3 Hz), 6.70 (d, 2H, J = 7.8 Hz), 6.32 (d, 2H, J = 7.3 Hz). Not soluble enough to get 13C NMR spectrum.
ACKNOWLEDGEMENTS
Support form Korean Research Foundation Grant (KRF-2008-521-E00189) is gratefully acknowledged. JMC is a recipient of BK-21 scholarship (2008).
References
1. R. G. Clarkson and M. Gomberg, J. Am. Chem. Soc., 1930, 52, 2881. CrossRef
2. (a) G. Haas and V. Prelog, Helv. Chim. Acta, 1969, 52, 1202; CrossRef (b) V. Prelog and D. Kovačević, Helv. Chim. Acta, 1979, 62, 2285; CrossRef (c) K. Neupert-Laves, and M. Dobler, Helv. Chim. Acta, 1981, 64, 1653; CrossRef (d) W. Bussmann and W. Simon, Helv. Chim. Acta, 1981, 64, 2101; CrossRef (e) V. Prelog and S. Mutak, Helv. Chim. Acta, 1983, 66, 2274; CrossRef (f) M. Dobler, M. Dumíc, M. Egli, and V. Prelog, Angew. Chem., Int. Ed. Engl., 1985, 24, 792; CrossRef (g) M. Egli and M. Dobler, Helv. Chim. Acta, 1986, 69, 626; CrossRef (h) V. Prelog, M. Kovačević, and M. Egli, Angew. Chem., Int. Ed. Engl., 1989, 28, 1147. CrossRef
3. (a) V. Alcázar Montero, L. Tomlinson, K. N. Houk, and F. Diederich, Tetrahedron Lett., 1991, 32, 5309; CrossRef (b) V. Alcázar and F. Diedrich, Angew. Chem., Int. Ed. Engl., 1992, 31, 1521; CrossRef (c) P. Lustenberger, E. Martinborough, T. Mordasini Denti, and F. Diederich, J. Chem. Soc., Perkin Trans. 2, 1998, 747; CrossRef (d) D. K. Smith and F. Diederich, Chem. Commun., 1998, 2501; CrossRef (f) F. Diederich and B. Felber, Proc. Natl. Acad. Sci. USA, 2002, 99, 4778. CrossRef
4. (a) M. Czugler, J. J. Stezowski, and E. Weber, J. Chem. Soc., Chem. Commun., 1983, 154; CrossRef (b) E. Weber, J. Ahrendt, M. Czugler, and I. Csöregh, Angew. Chem., Int. Ed. Engl., 1986, 25, 746; CrossRef (c) G. Das and A. D. Hamilton, Tetrahedron Lett., 1997, 38, 3675; CrossRef (d) A. Tejeda, A. Oliva, L. Simón, M. Grande, C. Caballero, and J. R. Morán, Tetrahedron Lett., 2000, 41, 4563. CrossRef
5. (a) J. M. Tour, R. Wu, and J. S. Schumm, J. Am. Chem. Soc., 1990, 112, 5662; CrossRef (b) J. M. Tour, R. Wu, and J. S. Schumm, J. Am. Chem. Soc., 1991, 113, 7064; CrossRef (c) J. Guay, A. Diaz, R. Wu, and J. M. Tour, J. Am. Chem. Soc., 1993, 115, 1869; CrossRef (d) U. Bach, K. De Cloedt, H. Spreitzer, and M. Grätzel, Adv. Mater., 2000, 12, 1060; CrossRef (e) J. Pei, J. Ni, X.-H. Zhou, X.-Y. Cao, and Y.-H. Lai, J. Org. Chem., 2002, 67, 8104; CrossRef (f) C.-c. Wu, T.-L. Liu, W.-Y. Hung, Y.-T. Lin, K.-T. Wong, R.-T. Chen, Y.-M. Chen, and Y.-Y. Chien, J. Am. Chem. Soc., 2003, 125, 3710. CrossRef
6. (a) D. S. Reddy, C.-F. Shu, and F.-I. Wu, J. Polym. Sci. Part A: Polym. Chem., 2002, 40, 262; CrossRef (b) C.-H. Chen, and C.-F. Shu, J. Polym. Sci. Part A: Polym. Chem., 2004, 42, 3314. CrossRef
7. (a) N. Johansson, D. A. dos Santos, S. Guo, J. Cornil, M. Fahlman, J. Salbeck, H. Schenk, H. Arwin, J. L. Brédas, and W. R. Salanek, J. Chem. Phys., 1997, 107, 2542; CrossRef (b) R. E. Martin and F. Diederich, Angew. Chem. Int. Ed., 1999, 38, 1350; CrossRef (c) C. D. Müller, A. Falcou, N. Reckefuss, M. Rojahn, V. Wiederhirn, P. Rudati, H. Frohne, O. Nuyken, H. Becker, and K. Meerholz, Nature, 2003, 421, 829; CrossRef (d) F. Laquai and D. Hertel, Appl. Phys. Lett., 2007, 90, 142109; CrossRef (d) C. Poriel, J.-J. Liang, J. Rault-Berthelot, Barriere, N. Cocherel, A. M. Z. Slawin, D. Horhant, M. Virboul, G. Alcaraz, N. Audebrand, L. Vignau, N. Huby, G. Wantz, and L. Hirsch., Chem. Eur. J., 2007, 13, 10055. CrossRef
8. (a) S. Tang, M. Liu, C. Gu, Y. Zhao, P. Lu, D. Lu, L. Liu, F. Shen, B. Yang, and Y. Ma, J. Org. Chem., 2008, 73, 4212; CrossRef (b) F. Thiemann, T. Piehler, D. Haase, W. Saak, and A. Lützen, Eur. J. Org. Chem., 2005, 1991. CrossRef
9. (a) F. Wu, E. C. Riesgo, R. P. Thummel, A. Juris, M. Hissler, A. Elghayoury, and R. Ziessel, Tetrahedron Lett., 1999, 40, 7311; CrossRef (b) A. Juris, L. Prodi, A. Harriman, R. Ziessel, M. Hissler, A. El-ghayoury, F. Wu, E. C. Riesgo, and R. P. Thummel, Inorg. Chem., 2000, 39, 3590. CrossRef
10. (a) S. Murase, T. Tominaga, and D. Kitazawa, D. Jpn. Kokai Tokkyo Koho, 2005, JP 2005093425; (b) T. Tominaga, D. Kitazawa, A. Makiyama, and A. Kohama, PCT Int. Appl., 2002, WO 2002043449, in which physical and spectral data were not given.
11. Y.-w. Qu, Ke-h. Shi, Ke-h. and Q.-c. Liu, Chin. J. Synth. Chem., 2008, 16, 15.
12. (a) M. A. F. M. Rahman and Y. Jahng, Heterocycles, 2007, 71, 2003; CrossRef (b) A. F. M. M. Rahman and Y. Jahng, Heteroatom Chem., 2007, 18, 650; CrossRef (c) A. F. M. M. Rahman and Y. Jahng, Heterocycles, 2008, 75, 871; CrossRef (d) M. A. F. M. Rahman and Y. Jahng, Heterocycles, 2008, 75, 2507. CrossRef
13. (a) J. H. Weisburger, E. K. Weisburger, and F. R. Ray, J. Am. Chem. Soc., 1950, 72, 4250; CrossRef (b) V. Prelog and D. Bedeković, Helv. Chim. Acta, 1979, 62, 2285; CrossRef (c) J. Pei, J. Ni, X.-H. Zhou, X.-Y. Cao and Y.-H. Lai, J. Org. Chem., 2002, 67, 4924; CrossRef (d) R. Wu, J. S. Schumm, D. L. Pearson, and J. M. Tour, J. Org. Chem., 1996, 61, 6906. CrossRef
14. G. Haas and V. Prelog, Helv. Chim. Acta, 1969, 52, 1202. CrossRef
15. (a) J. W. Opie and L. I. Smith, Org. Syn. Col. Vol. III, 1955, 56; (b) G. Majewicz and O. A. Caluwe, J. Org. Chem., 1974, 39, 720; CrossRef (c) C.-Y. Hung, T.-L. Wang, Z. Shi, and R. P. Thummel, Tetrahedron, 1994, 50, 10685; CrossRef (d) E. C. Riesgo, X. Jin, and R. P. Thummel, J. Org. Chem., 1996, 61, 3017; CrossRef (e) J. K. Son, J. K. Son, and Y. Jahng, Heterocycles, 2002, 57, 1109. CrossRef
16. E. Pretsch, J. Seibl, W. Simon, and T. Clerc, Tables of Spectral Data for Structure Determination of Organic Compounds. 13C-NMR, 1H NMR, IR, MS, and UV/VIS –Chemical Laboratory Practice. 2nd Ed., Springer-Verlag, Berlin Heidelberg 1989.
17. Y. Jahng, R. P. Thummel, and S. G. Bott, Inorg. Chem., 1997, 36, 3133. CrossRef
18. (a) V. Hedge, Y. Jahng, and R. P. Thummel, Tetrahedron Lett., 1987, 28, 4203; CrossRef (b) Unpublished results, in which similar results were observed in the reaction of 2-(prid-2’-yl)benzo[b]-1,10-phenthroline with various d6-metals.