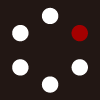
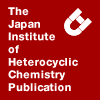
HETEROCYCLES
An International Journal for Reviews and Communications in Heterocyclic ChemistryWeb Edition ISSN: 1881-0942
Published online by The Japan Institute of Heterocyclic Chemistry
e-Journal
Full Text HTML
Received, 13th June, 2009, Accepted, 16th July, 2009, Published online, 17th July, 2009.
DOI: 10.3987/COM-09-S(S)34
■ Asymmetric Hydrogenation of Aromatic Heterocyclic Ketones Catalyzed by the MsDPEN–Cp*Ir(III) Complex
Noriyuki Utsumi, Kunihiko Tsutsumi, Masahiko Watanabe, Kunihiko Murata, Noriyoshi Arai, Nobuhito Kurono, and Takeshi Ohkuma*
Graduate School of Engineering, Hokkaido University, Kita 13 Nishi 8, Kita-ku, Sapporo, Hokkaido 060-0828, , Japan
Abstract
Asymmetric hydrogenation of aromatic heterocyclic ketones catalyzed by Cp*Ir(OTf)(Msdpen) (MsDPEN = N-(methanesulfonyl)-1,2-diphenylethylenediamine) affords the heterocyclic alcohols in 93% to >99% ee. The reaction is conducted in a methanolic solution with a substrate-to-catalyst molar ratio of 200–5000 under 15 atm of H2. The heterocyclic rings of substrates are left intact.Asymmetric hydrogenation of ketones is a key technology to synthesize optically active secondary alcohols.1 Selection of appropriate chiral catalysts according to the structure and chemical features of the ketonic substrates is crucial to achieve high reactivity and enantioselectivity. We have previously exploited RuXY(diphosphine)(1,2-diamine)2,3 and RuXY(diphosphine)(α-picolylamine)4 (X = Y = Cl or X = H, Y = η1-BH4), which act as excellent catalysts for asymmetric hydrogenation of simple ketones in basic or slightly basic alcoholic solutions. However, these catalysts cannot be used for the reaction of seriously base-labile 3(2H)-benzofuranones, 4-chromanones, and their derivatives. We have recently reported that Ru(OTf)(Tsdpen)(η6-p-cymene) (TfO– = trifluoromethanesulfonate, TsDPEN = N-(p-toluenesulfonyl)-1,2-diphenylethylenediamine) without any basic compounds catalyzes hydrogenation of 4-chromanone to give the chiral alcohol in 97% ee quantitatively.5,6 The neutral to slightly acidic reaction conditions prevent decomposition of the substrate. The isoelectronic Ir(III) complex, Cp*Ir(OTf)(Msdpen) (3) (Cp* = pentamethylcyclopentadienyl, MsDPEN = N-(methanesulfonyl)-1,2-diphenylethylenediamine), has been revealed to be an excellent catalyst for asymmetric hydrogenation of α-hydroxy ketones, which are another class of base-sensitive ketonic substrates.7 We herein describe enantioselective hydrogenation of aromatic heterocyclic ketones catalyzed by the Ir(III) complex 3. The reaction of 4-chromanone resulted in an optical yield of 99%, even higher than that by Ru(OTf)(Tsdpen)(η6-p-cymene). A series of heterocyclic alcohols was obtained with high enantioselectivity.
Asymmetric hydrogenation of 3(2H)-benzofuranone (1a) with the Ir complex, (S,S)-3, at a substrate-to-catalyst molar ratio (S/C) of 500 in CH3OH at 60 °C under 15 atm of H2 was completed in 24 h to afford the desired alcohol, (R)-2a, in 94% ee (Table 1, entry 1).8 The heterocyclic ring was left intact. To our knowledge, this is the first example of asymmetric hydrogenation of 3(2H)-benzofuranones. The substrate with a CH3O group at the C7 position, 1b, was also converted to (R)-2b in high enantioselectivity (entry 2).9 When 1-indanone (1c) was hydrogenated at 30 °C (S/C = 200, 15 atm H2, 24 h), (S)-1-indanol [(S)-2c] was obtained in 98% ee and 95% yield with some unidentified byproducts, possible due to instability of the substrate (entry 3).10–12 The reaction conducted at 60 °C was stopped at about 50% conversion. The Ir complex (S,S)-3 exhibited excellent performance in hydrogenation of 4-chromanone (1d). Thus, the reaction with an S/C of 5000 (15 atm H2, 60 °C) for 24 h quantitatively gave (S)-2d in 99% ee (entry 4).5,11,13 The high substrate concentration of 3.5 M is suitable for practical use. The catalytic activity of 3 for hydrogenation of 1d is comparable to that of an isoelectronic Ru(OTf)(Tsdpen)(η6-p-cymene), but the enantioselective ability is higher than that of the Ru complex (99% vs. 97%).5 4-Chromanones with a CH3 or Cl substituent at the C6 position, 1e and 1f, were also smoothly hydrogenated with 3 in >98% optical yield (entries 5 and 6).14,15 The electronic and steric properties of these substituents little affected the reactivity and enantioselectivity. The Ir-catalyzed hydrogenation was applicable to 4-thiochromanone (1g) without loss of thiocyclic skeleton (entry 7).11 The desired alcohol (S)-2g was obtained in >99% ee quantitatively.16 A cyclic keto sulfone 1h, which is hardly soluble in CH3OH, was hydrogenated in a 3:2 mixture of CH3OH and DMF ([1h] = 2.0 M) to give (S)-2h in 98% ee (entry 8).17 Hydrogenation of 1-tert-butoxycarbonyl-1,2,3,4-tetrahydro-4-quinolinone (1i) afforded 2i in 96% ee and 90% yield (entry 9).18 It is noteworthy that hydrogenation of 1-tetralone (1j), a carbocyclic aromatic ketone, gave the alcohol 2j in only 93% ee and 88% yield (entry 10: S/C = 500, 15 atm H2, 60 °C, 24 h),11,12,19,20 suggesting that a heteroatom, especially oxygen, in the aliphatic ring of the substrate plays crucial roles in achieving high reactivity and enantioselectivity (see for example entry 4).
In summary, we have disclosed enantioselective hydrogenation of aromatic heterocyclic ketones catalyzed by the MsDPEN–Cp*Ir(III) complex 3. The reaction is conducted with an S/C of 200–5000 under 15 atm of H2 to give the heterocyclic alcohols in >99% ee in the best cases. The heterocyclic structures are left intact. This is the first example of asymmetric hydrogenation of 3(2H)-benzofuranones, 4-thiochromanone 1,1-dioxides, and 1,2,3,4-tetrahydro-4-quinolinones. Thus, this method provides an efficient strategy to synthesize chiral heterocyclic alcohols.
ACKNOWLEDGEMENTS
This work was supported by a Grant-in-Aid from the Japan Society for the Promotion of Science (JSPS) (Grant No. 21350048).
References
1. Reviews on asymmetric hydrogenation of ketones: T. Ohkuma and R. Noyori, ‘Comprehensive Asymmetric Catalysis,’ Vol. 1, ed. by E. N. Jacobsen, A. Pfaltz, and H. Yamamoto, Springer, Berlin–Heidelberg, 1999, pp. 199–246; T. Ohkuma, M. Kitamura, and R. Noyori, ‘Catalytic Asymmetric Synthesis, 2nd ed.,’ ed. by I. Ojima, Wiley-VCH, New York, 2000, pp. 1–110; CrossRef T. Ohkuma and R. Noyori, ‘Transition Metals for Organic Synthesis, 2nd ed.,’ Vol. 2, ed. by M. Beller and C. Bolm, Wiley-VCH, Weinheim, 2004, pp. 29–113; T. Ohkuma and R. Noyori, ‘The Handbook of Homogeneous Hydrogenation,’ Vol. 3, ed. by J. G. de Vries and C. J. Elsevier, Wiley-VCH, Weinheim, 2007, pp. 1105–1163.
2. See for example: H. Doucet, T. Ohkuma, K. Murata, T. Yokozawa, M. Kozawa, E. Katayama, A. F. England, T. Ikariya, and R. Noyori, Angew. Chem. Int. Ed., 1998, 37, 1703; CrossRef T. Ohkuma, M. Koizumi, H. Doucet, T. Pham, M. Kozawa, K. Murata, E. Katayama, T. Yokozawa, T. Ikariya, and R. Noyori, J. Am. Chem. Soc., 1998, 120, 13529; CrossRef T. Ohkuma, M. Koizumi, K. Muñiz, G. Hilt, C, Kabuto, and R. Noyori, J. Am. Chem. Soc., 2002, 124, 6508; CrossRef N. Arai, H. Ooka, K. Azuma, T. Yabuuchi, N. Kurono, T. Inoue, and T. Ohkuma, Org. Lett., 2007, 9, 939; CrossRef N. Arai, K. Azuma, N. Nii, and T. Ohkuma, Angew. Chem. Int. Ed., 2008, 47, 7457; CrossRef H. Ooka, N. Arai, K. Azuma, N. Kurono, and T. Ohkuma, J. Org. Chem., 2008, 73, 9084. CrossRef
3. Reviews: T. Ohkuma, J. Synth. Org. Chem. Jpn., 2007, 65, 1070; R. Noyori and T. Ohkuma, Angew. Chem. Int. Ed., 2001, 40, 40. CrossRef
4. T. Ohkuma, C. A. Sandoval, R. Srinivasan, Q. Lin, Y. Wei, K. Muñiz, and R. Noyori, J. Am. Chem. Soc., 2005, 127, 8288; CrossRef N. Arai, K. Suzuki, S. Sugizaki, H. Sorimachi, and T. Ohkuma, Angew. Chem. Int. Ed., 2008, 47, 1770; CrossRef K. Tsutsumi, T. Katayama, N. Utsumi, K. Murata, N. Arai, N. Kurono, and T. Ohkuma, Org. Proc. Res. Dev., 2009, 13, 625. CrossRef
5. T. Ohkuma, N. Utsumi, K. Tsutsumi, K. Murata, C. A. Sandoval, and R. Noyori, J. Am. Chem. Soc., 2006, 128, 8724; CrossRef C. A. Sandoval, T. Ohkuma, N. Utsumi, K. Tsutsumi, K. Murata, and R. Noyori, Chem. Asian J., 2006, 1–2, 102. CrossRef
6. For asymmetric hydrogenation of α-chloro ketones catalyzed by η6-arene/TsDPEN–Ru(II) complexes, see: T. Ohkuma, K. Tsutsumi, N. Utsumi, N. Arai, R. Noyori, and K. Murata, Org. Lett., 2007, 9, 255. CrossRef
7. T. Ohkuma, N. Utsumi, M. Watanabe, K. Tsutsumi, N. Arai, and K. Murata, Org. Lett., 2007, 9, 2565. CrossRef
8. (R)-2,3-Dihydro-3-benzofuranol (R)-2a: HPLC analytical conditions: column, CHIRALCEL OJ-H (4.6 mm i.d. x 250 mm); eluent, n-hexane/2-propanol = 99/1; flow rate, 1.5 mL/min; temp, 35 °C; detection, UV 220 nm; retention time (tR) of (R)-2a, 34.4 min; tR of (S)-2a, 31.9 min. [α]D25 –61.6 (c 1.68, CHCl3) [lit., [α]D25 +47 (c 1.70, CHCl3), 73% ee, (S)-isomer, D. R. Boyd, N. D. Sharma, R. Boyle, J. F. Malone, J. Chima, and H. Dalton, Tetrahedron: Asymmery, 1993, 4, 1307]. 1H NMR (400 MHz, CDCl3) δ 1.88 (d, 1H, J = 7.3 Hz), 4.45 (dd, 1H, J = 2.7, 11.0 Hz), 4.54 (dd, 1H, J = 6.4, 11.0 Hz), 5.36 (m, 1H), 6.88–6.97 (m, 2H), 7.28 (m, 1H), 7.43 (m, 1H). 13C NMR (100 MHz, CDCl3) δ 72.2, 79.1, 110.6, 121.0, 125.4, 128.2, 130.8, 160.2. CrossRef
9. (R)-7-Methoxy-2,3-dihydro-3-benzofuranol (R)-2b: HPLC analytical conditions: column, CHIRALCEL OJ-H; eluent, n-hexane/2-propanol = 9/1; flow rate, 1.0 mL/min; temp, 35 °C; detection, UV 220 nm; tR of (R)-2b, 19.9 min; tR of (S)-2b, 24.2 min. [α]D25 –43.2 (c 1.00, CHCl3). 1H NMR (400 MHz, CDCl3) δ 1.97 (br d, 1H, J = 7.3 Hz), 3.89 (s, 3H), 4.51 (dd, 1H, J = 2.3, 10.5 Hz), 4.59 (dd, 1H, J = 6.4, 10.5 Hz), 5.38 (m, 1H), 6.86 (m, 1H), 6.91 (t, 1H, J = 7.8 Hz), 7.04 (m, 1H). 13C NMR (100 MHz, CDCl3) δ 56.0, 72.8, 79.7, 113.1, 117.2, 121.8, 129.3, 145.1, 148.9. The absolute configuration was estimated by 1H-NMR analysis after conversion to the (R)- and (S)-MTPA esters as described in the literature, see: I. Ohtani, T. Kusumi, Y. Kashman, and H. Kakisawa, J. Am. Chem. Soc., 1991, 113, 4092. CrossRef
10. (S)-1-Indanol (S)-2c: HPLC analytical conditions: column, CHIRALCEL OB-H (4.6 mm i.d. x 250 mm); eluent, n-hexane/2-propanol = 9/1; flow rate, 0.5 mL/min; temp, 30 °C; detection, UV 220 nm; tR of (R)-2c, 11.1 min; tR of (S)-2c, 16.2 min. [α]D25 +31.2 (c 1.03, CHCl3) [lit., [α]D25 +29.8 (c 2.0, CHCl3), 98% ee, (S)-isomer, J. S. Yadav, S. Nanda, P. T. Reddy, and A. B. Rao, J. Org. Chem., 2002, 67, 3900]. 1H NMR (400 MHz, CDCl3) δ 1.71 (br s, 1H), 1.95 (m, 1H), 2.50 (m, 1H), 2.83 (m, 1H), 3.06 (ddd, 1H, J = 4.6, 8.2, 15.6 Hz), 5.25 (dd, 1H, J = 6.0, 6.4 Hz), 7.21–7.34 (m, 3H), 7.42 (d, 1H, J = 5.5 Hz). 13C NMR (100 MHz, CDCl3) δ 29.8, 36.0, 76.5, 124.2, 124.9, 126.7, 128.3, 143.3, 145.0. CrossRef
11. For asymmetric hydrogenation of cycloalkanones catalyzed by the BINAP–Ir(I)–aminophosphine system, see: X. Zhang, T. Taketomi, T. Yoshizumi, H. Kumobayashi, S. Akutagawa, K. Mashima, and H. Takaya, J. Am. Chem. Soc., 1993, 115, 3318. CrossRef
12. For asymmetric hydrogenation of aromatic ketones catalyzed by Ru(II) complexes with some amine-based chiral ligands, see: H. Huang, T. Okuno, K. Tsuda, M. Yoshimura, and M. Kitamura, J. Am. Chem. Soc., 2006, 128, 8716; CrossRef M. Ito, Y. Endo, and T. Ikariya, Organometallics, 2008, 27, 6053. CrossRef
13. Procedure for asymmetric hydrogenation of 4-chromanone (1d) with (S,S)-3: 4-Chromanone (1d) (2.97 g, 20.0 mmol) and (S,S)-3 (3.10 mg, 4.0 µmol) were placed in a 100-mL glass autoclave. The atmosphere was replaced with Ar gas, and MeOH (3.3 mL) was added to this mixture. Hydrogen was initially introduced into the autoclave at a pressure of 10 atm, before being reduced to 1 atm. This procedure was repeated five times. Then the autoclave was pressurized with H2 gas (15 atm), and the solution was stirred vigorously at 60 °C for 24 h. The 1H-NMR and chiral HPLC analysis indicate that (S)-4-chromanol [(S)-2d] was obtained in 99% ee and >99% yield. Silica gel chromatography (silica gel 60N (Kanto Chemical), eluent: Et2O) of the crude product gave pure (S)-4-chromanol [(S)-2d] in 99% ee and 99% isolated yield (2.98 g, colorless crystals). The HPLC analytical conditions were the same as those described in note 8; tR of (R)-2d, 30.3 min; tR of (S)-2d, 25.5 min. [α]D25 –71.3 (c 0.50, EtOH) [lit.,5 [α]D25 –77 (c 0.5, EtOH), 95% ee, (S)-isomer]. 1H NMR (400 MHz, CDCl3) δ 1.86 (br s, 1H), 2.00–2.17 (m, 2H), 4.26–4.31 (m, 2H), 4.79 (dd, 1H, J = 3.7, 4.1 Hz), 6.84–6.94 (m, 2H), 7.19–7.32 (m, 2H). 13C NMR (100 MHz, CDCl3) δ 30.8, 61.9, 63.2, 117.1, 120.6, 124.3, 129.6, 129.7, 154.6.
14. (S)-6-Chloro-4-chromanol (S)-2f: HPLC analytical conditions were the same as those described in note 8; tR of (R)-2f, 33.1 min; tR of (S)-2f, 34.8 min. [α]D25 –26.3 (c 1.00, CHCl3) [lit.,5 [α]D25 –24 (c 1.0, CHCl3), 98% ee, (S)-isomer]. 1H NMR (400 MHz, CDCl3) δ 1.89 (br s, 1H), 1.99–2.16 (m, 2H), 4.23–4.29 (m, 2H), 4.76 (dd, 1H, J = 4.1, 4.6 Hz), 6.78 (d, 1H, J = 9.2 Hz), 7.15 (dd, 1H, J = 2.7, 9.2 Hz), 7.30 (d, 1H, J = 2.7 Hz). 13C NMR (100 MHz, CDCl3) δ 30.6, 62.2, 63.1, 118.5, 125.2, 125.6, 129.1, 129.6, 153.2.
15. (S)-6-Chloro-4-chromanol (S)-2f: HPLC analytical conditions were the same as those described in note 8; tR of (R)-2f, 33.1 min; tR of (S)-2f, 34.8 min. [α]D25 –26.3 (c 1.00, CHCl3) [lit.,5 [α]D25 –24 (c 1.0, CHCl3), 98% ee, (S)-isomer]. 1H NMR (400 MHz, CDCl3) δ 1.89 (br s, 1H), 1.99–2.16 (m, 2H), 4.23–4.29 (m, 2H), 4.76 (dd, 1H, J = 4.1, 4.6 Hz), 6.78 (d, 1H, J = 9.2 Hz), 7.15 (dd, 1H, J = 2.7, 9.2 Hz), 7.30 (d, 1H, J = 2.7 Hz). 13C NMR (100 MHz, CDCl3) δ 30.6, 62.2, 63.1, 118.5, 125.2, 125.6, 129.1, 129.6, 153.2.
16. (S)-4-Thiochromanol (S)-2g: HPLC analytical conditions: column, CHIRALCEL OD (4.6 mm i.d. x 250 mm); eluent, n-hexane/2-propanol = 98/2; flow rate, 1.0 mL/min; temp, 35 °C; detection, UV 220 nm; tR of (R)-2g, 27.4 min; tR of (S)-2g, 21.0 min. [α]D25 –136 (c 1.00, CHCl3) [lit., [α]D22 –136 (c 1.00, CHCl3), 97% ee, (S)-isomer, C. V. Ursini, G. H. M. Dias, and J. A. R. Rodrigues, J. Organomet. Chem., 2005, 690, 3176]. 1H NMR (400 MHz, CDCl3) δ 1.84 (br s, 1H), 2.05 (m, 1H), 2.35 (m, 1H), 2.86 (ddd, 1H, J = 3.7, 5.5, 12.4 Hz), 3.32 (dt, 1H, J = 12.4, 2.8 Hz), 4.81 (m, 1H), 7.05–7.18 (m, 3H), 7.31 (d, 1H, J = 6.9 Hz). 13C NMR (100 MHz, CDCl3) δ 21.5, 30.0, 66.5, 124.3, 126.7, 128.4, 130.3, 133.2, 134.6. CrossRef
17. (S)-4-Thiochromanol 1,1-dioxide (S)-2h: HPLC analytical conditions were the same as those described in note 9; tR of (R)-2h, 34.3 min; tR of (S)-2h, 37.8 min. [α]D25 +5.1 (c 1.00, CHCl3). 1H NMR (400 MHz, CDCl3) δ 2.30 (d, 1H, J = 5.5 Hz), 2.55 (m, 1H), 2.75 (m, 1H), 3.30 (ddd, 1H, J = 3.2, 7.8, 14.2 Hz), 3.72 (ddd, 1H, J = 3.2, 11.0, 14.2 Hz), 4.93 (dd, 1H, J = 5.5, 9.2 Hz), 7.51–7.61 (m, 3H), 7.90 (d, 1H, J = 8.2 Hz). 13C NMR (100 MHz, CDCl3) δ 29.4, 46.5, 65.7, 123.6, 129.1, 129.7, 133.0, 137.7, 137.8. The absolute configuration was estimated by 1H-NMR analysis after conversion to the (R)- and (S)-MTPA esters as described in the literature.9 .
18. (S)-1-tert-Butoxycarbonyl-1,2,3,4-tetrahydro-4-quinolinol (S)-2i: HPLC analytical conditions were the same as those described in note 8; tR of (R)-2i, 21.6 min; tR of (S)-2i, 18.6 min. [α]D25 –27.7 (c 1.04, CHCl3). 1H NMR (400 MHz, CDCl3) δ 1.53 (s, 9H), 1.85 (br s, 1H), 1.98–2.11 (m, 2H), 3.59 (ddd, 1H, J = 3.7, 9.6, 13.3 Hz), 4.05 (ddd, 1H, J = 4.6, 6.0, 13.3 Hz), 4.77 (t, 1H, J = 4.6 Hz), 7.07 (m, 1H), 7.25 (m, 1H), 7.38 (m, 1H), 7.78 (m, 1H), 13C NMR (100 MHz, CDCl3) δ 28.3, 31.9, 40.4, 65.9, 81.2, 123.4, 123.6, 127.9, 128.2, 130.5, 137.8, 153.6. The absolute configuration was estimated by 1H-NMR analysis after conversion to the (R)- and (S)-MTPA esters as described in the literature.9 .
19. For asymmetric hydrogenation of 1-tetralones catalyzed by BINAP/chiral 1,4-diamine–Ru(II) complexes, see: T. Ohkuma, T. Hattori, H. Ooka, T. Inoue, and R. Noyori, Org. Lett., 2004, 6, 2681. CrossRef
20. (S)-1-Tetralol (S)-2j: HPLC analytical conditions were the same as those described in note 8; tR of (R)-2j, 14.6 min; tR of (S)-2j, 13.3 min. [α]D25 +31.1 (c 1.05, CHCl3) [lit.,19 [α]D23 –33.5 (c 1.01, CHCl3), 99% ee, (R)-isomer]. 1H NMR (400 MHz, CDCl3) δ 1.65 (br s, 1H), 1.76–2.02 (m, 4H), 2.71–2.86 (m, 2H), 4.79 (dd, 1H, J = 3.7, 5.5 Hz), 7.11 (m, 1H), 7.18–7.22 (m, 2H), 7.43 (m, 1H), 13C NMR (100 MHz, CDCl3) δ 18.7, 29.2, 32.2, 68.1, 126.1, 127.5, 128.6, 129.0, 137.1, 138.7.