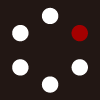
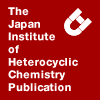
HETEROCYCLES
An International Journal for Reviews and Communications in Heterocyclic ChemistryWeb Edition ISSN: 1881-0942
Published online by The Japan Institute of Heterocyclic Chemistry
e-Journal
Full Text HTML
Received, 20th August, 2009, Accepted, 26th October, 2009, Published online, 28th October, 2009.
DOI: 10.3987/REV-09-SR(S)6
■ Recent Development for Formation of Aromatic Compounds via Metallacyclopentadienes as Metal-Containing Heterocycles
Lishan Zhou, Shi Li, Ken-ichiro Kanno, and Tamotsu Takahashi*
Catalysis Research Center and Graduate School of Pharmaceutical Science, Hokkaido University, Sapporo 001-0021, Japan
Abstract
Metallacyclopentadiene, which is recognized as a metal-containing heterocycle, is an important intermediate for the formation of aromatic derivatives. In this short review, we summarized the recent advance in selective aromatic compounds formation catalyzed or mediated by transition metal complexes via metallacyclopentadienes which are formed by intermolecular coupling of various alkynes including unsymmetrical alkynes, two different alkynes and three different alkynes. Some reaction mechanisms were also reviewed.1. INTRODUCTION
Reactions of two alkynes with low valent transition metals generally provide metallacyclopentadienes 1 as intermediates for the formation of benzene derivatives. The metallacyclopentadiene 1 is a cyclic compound with a metal. Therefore, it can be recognized as a metal-containing heterocycle (Scheme 1). Stepwise reaction of the metallacyclopentadienes with the third alkyne affords benzene derivatives 2 and 3.
Many reviews on benzene derivatives formation from alkynes using transition metals have been published.1 This review focuses on the recent development of selective formation of benzene derivatives from alkynes using transition metals as stoichiometric reactions or catalytic reactions.
Generally speaking, these type reactions include two different modes: intramolecular coupling reaction and intermolecular coupling reaction (Scheme 1). The intramolecular cyclotrimerization of triynes to prepare benzene derivatives 2 via metallacyclopentadienes 1 have been established as powerful synthetic methods. However, intermolecular and partially intermolecular versions generally have encountered difficulties in the control of chemo- and regioselectivity. When unsymmetrical alkynes or different alkynes were employed for the cyclotrimerization, in most cases, isomeric aromatic derivatives 3 were generally formed.2 This severely limited the utility of this method. Therefore, the search for a highly chemo- and regioselective intermolecular [2 + 2 + 2] cycloaddition reaction of alkynes is greatly challenging. Recently, several groups have devoted substantial effort in this area. Various transition metal complexes have been used to overcome this problem. This review introduces some advance in intermolecular cyclotrimerization of unsymmetrical alkynes or different alkynes catalyzed or mediated by transition metal complexes.
2. CATALYTIC HOMO-CYCLOTRIMERIZATION OF UNSYMMETRICAL ALKYNES
There are two possible isomeric products of trisubstituted benzenes in a catalytic homo-cyclotrimerization of an unsymmetrical alkyne. As shown in Scheme 2, the reaction of a terminal alkyne can afford the corresponding 1,3,5- and 1,2,4- trisubstituted benzenes 4 and 5, respectively.
According to generally accepted metallacyclopentadiene mechanism shown in Scheme 3, 1,2,4-isomer 5 is formed exclusively via symmetrical metallacyclopentadienes 6 or 8, and to some degree via unsymmetrical 7. On the other hand, the possibility for the formation of 1,3,5-isomer 4 is smaller than that for 1,2,4-isomer 5, since 4 can be formed via metalacycle 7 only in competition with formation of 5. This consideration would conclude that the key points to achieve high selectivity for the formation of one of the isomers are (1) selective formation of the intermediary metallacyclopentadiene, and (2) in the case of metalacycle 7, regioselective addition of the third alkyne. Especially, for selective formation of 1,3,5-isomer 4, the second key point is very crucial.
Tables 1 and 2 summarized the results of catalytic homo-cyclotrimerization of phenylacetylene to afford the 1,3,5- or 1,2,4-triphenylbenzenes 9 or 10 as a major isomer, respectively (Scheme 4). A comparison of the degree and type of selectivity depending on the employed catalysts is discussed here.
When camphorimine palladium complex 11 was employed for cyclotrimerization of phenylacetylene, 1,3,5-triphenylbenzene 9 was obtained as an exclusive benzene product (Table 1, entry 1).3 Although the product yield was low, the unique regioselectivity is worth noting. The same catalyst showed much higher efficiency for unsymmetrical internal alkyne without loss of regioselectivity. The cyclotrimerization of 1-phenylpropyne gave 1,3,5-trimethyl-2,4,6-triphenylbenzene in 74% yield as a sole product.
The predominant formation of the 1,3,5-isomer 9 was also observed in the reactions with ruthenium dinuclear complex 12 (entry 2),4 half-sandwiched cobalt 13 (entry 3),5 cycloheptatrienyliron 14 (entry 4),6 and dinuclear niobium 15 (entry 5).7 Among them, niobium catalyst 15 showed excellent selectivity and excellent product yield compared with the others.
According to the metalacycle mechanism mentioned above, 2,4-disubstituted metallacyclopentadiene 7 must be formed as a major intermediate in these cases. In the niobium-catalyzed reaction, a possible mechanism was proposed as shown in Scheme 5.7 Dinuclear complex 15 reacts with two molecules of phenylacetylene to afford chlorine-bridged dimer of metallacyclopropene 16, which was observed spectroscopically. Further insertion of the second and third alkynes can afford the corresponding dimer of metallacyclopentadiene 17, and that of metallacycloheptatriene 18 followed by reductive elimination to produce the benzene 9 along with regeneration of the catalyst 15. It is worth noting that both of the alkyne insertion steps proceed with high regioselectivity. Since the related mononuclear niobium complex did not show any catalytic activity, such superior efficiency can be ascribed to the dinuclear structure.
Palladium catalyst supported on gold nanoparticle 19 gave the 1,2,4-isomer 10 as a major product (Table 2, entry 1).8 Using a cobalt carbonyl cluster Co3(CO)9(μ3-CH) 20 as catalyst, 1,2,4-isomeric benzene 10 was obtained with excellent regioselectivity (entry 2).9 The trinuclear complex is stable and easily prepared from dicobalt octacarbonyl compound and trihalomethane. The simple cobalt catalyst system 21 was also found for the efficient and regioselective cyclotrimerization of alkynes (entry 3).10 In the case of phenylacetylene, 1,2,4-triphenylbenzene was obtained in 99% yield in 95:5 regioselectivity. The cobalt catalyst system 21 is applicable for cyclotrimerization of unsymmetrical internal alkynes to furnish the corresponding 1,2,4-substituted benzenes with good regioselectivity in high yields. In the cobalt catalyst system, when sterically demanding iminomethylpyridine was used as a ligand instead of bipyridine, the reaction system 22 became less sensitive to moisture without loss of efficiency (entry 4).11
Rhodium catalysts also showed outstanding regioselectivity for the 1,2,4-isomer 10. Catalytic activity of cationic rhodium(I) segphos complex 23 is exceedingly high and the reaction can be carried out under mild conditions (entry 5).12 Although the reaction conditions are more drastic, just simple combination of rhodium(III) chloride and amine 24 produced efficient catalytic system (entry 6).13
Except for the late transition metal catalysts mentioned above, a titanium complex 25 having 1,2-alternate bridged p-tert-butylcalix[4]arene can also act as a highly regioselective catalyst (entry 7).14 The complex is pre-activated with sodium or magnesium metal. The reaction of activated 25 in the presence of an excess of trimethylsilylacetylene produced the corresponding titanium complex with η6-coordinated 1,2,4-tris(trimethylsilyl)benzene, which is also an efficient catalyst for cyclotrimerization of alkynes at room temperature.
Cross-cyclotrimerization of trimethylsilylacetylene with 4-chlorostyrene with titanium complex 25 gave the corresponding cyclohexadiene 27 as a major product (scheme 6). The result suggests the formation of α,β'-disubstituted metalacycle 26 predominantly. This means that although the α,α’'- or β,β'’-disubstituted metalacycles can afford the corresponding 1,2,4-trisubstituted benzene exclusively in the homo-cyclotrimerization, their contribution is minor. Moreover, insertion of the third alkyne into metalacycle 26 should occur in highly regioselective manner. Probably, the major reason of such high regioselectivity can be ascribed to sterically demanding nature of the calix[4]arene ligand.
3. CATALYTIC CROSS-CYCLOTRIMERIZATION OF TWO DIFFERENT ALKYNES
In trimerization reactions using two different alkynes, late transition metals, especially group 8-10 metals, were mainly used as catalysts. Mitsudo et al. developed a ruthenium-catalyzed synthesis of o-phthalates by intermolecular cycloaddition of two different alkynes.15 Excess amount of terminal alkynes reacted with DMAD in the presence of Cp*RuCl(cod) catalyst to give an isomeric mixture of dialkylated o-phthalates in high yield. As shown in Scheme 7, ruthenacycle 28 which consists of two different alkynes was proposed as a plausible intermediate.
An efficient cobalt-catalyzed cyclotrimerization for synthesis of benzolactones 29 was reported (Scheme 8).16 In this reaction, alkynyl alcohols reacted with 2 equiv of acetylenic esters to form substituted benzolactones 29 in a highly chemo and regioselective fashion with excellent yields. Interestingly, when the catalyst was changed, the regioselectivity was reversed at the metalacycle formation step (Scheme 9). In the case of the cobalt catalyst, the corresponding metallacyclopentadiene 30 having two ester groups at α,α’'-positions, whereas the β,β'-isomer 31 formed in that of the nickel catalyst and no transesterification occurred to afford benzyl alcohol 32 in a good yield.
Adoption of iridium-phosphine complex in alkyne cyclotrimerization was also reported.17 As shown in Scheme 10, chemoselectivity of the iridium catalyst can be controlled by changing the employed phosphine ligands. When dppe ligand was used for the catalysis, 2:1 coupling products benzene tetraester 34 was obtained in excellent yield and selectivity. On the other hand, when polyfluorinated diphosphine, 1,2-bis[bis(pentafluorophenyl)phosphino]ethane is used, 1:2 coupling product o-phthalate 36 was obtained with high selectivity. These results suggest the complete difference of the intermediary metalacycles. In the case of dppe, benzene tetraester 34 was formed via iridacycle 33, whereas o-phthalate 36 was obtained in the reaction with polyfluorinated dppe via iridacycle 35 as a predominant intermediate. The electron-deficient nature of the fluorinated ligand would make coordination of electron-rich alkyne B toward the iridium center much more favored than that of electron-deficient alkyne A.
Highly chemo- and regioselective intermolecular cyclotrimerization of two different alkyne moieties catalyzed by rhodium (I) complex was also reported (Scheme 11).12,18 In the presence of a cationic rhodium(I)/H8-BINAP complex, excellent regioselectivity was observed. Two molecules of a wide variety of terminal alkynes cleanly reacted with one molecule of a dialkyl acetylenedicarboxylate to give 3,6-disubstituted dialkyl phthalates 37 in high yields and with high regioselectivity.
Based on the numerous experimental results, the origin of the high selectivity was explained as shown in Scheme 12. First, two different alkynes react with cationic rhodium(I) in 1:1 ratio to afford the corresponding metallacyclopentadiene 38. The origin of this selectivity is due to the steric reason. Coordination of another terminal alkyne to 38 affords complex 39. In this step, the electronic nature of the α-carbon of the metalacycle and the coordinated alkyne determines the regioselectivity to afford 1,2,3,4-tetrasubstituted benzene.
4. CROSS-CYCLOTRIMERIZATION OF THREE DOFFERENT ALKYNES
One of the major problems of selective benzene formation from three different alkynes is the control of combination of alkynes. It has been difficult to prepare cleanly the unsymmetrical metallacyclopentadienes using transition metals by the intermolecular coupling of two different alkynes. To solve this problem,19 Takahashi and co-workers studied the formation of unsymmetrical zirconacyclopentadienes. Symmetrical zirconacyclopentadienes were conveniently prepared from alkynes and Cp2ZrBu2 (Negishi reagent).20 They found the use of ethylene gas was a prerequisite to prepare very clean unsymmetrical zirconacyclopentadienes.21 The first alkyne was treated with Negishi reagent under ethylene gas to give zirconacyclopentenes 40. Subsequent addition of a second alkyne to the species at 50 °C gave unsymmetrical zirconacyclopentadienes 41 selectively in high yields. Homo-coupling products of either the first alkyne or the second alkyne were negligible (Scheme 13).
The use of unsymmetrical zirconacyclopentadienes resulted in the formation of unsymmetrical benzene derivatives 42.22 Thus prepared benzene rings have alkyl, aryl, trimethylsilyl and ester groups. This was the first and practical example of the selective coupling of three different alkynes in high yields in one-pot.
They found zirconacyclopentadienes 41 reacted with alkynes with electron-donating groups as well as alkynes with electron-withdrawing groups to give benzene derivatives 42 in the presence of NiX2(PPh3)2 (X = Cl or Br) in good yields.23 Using Zr/Cu and Zr/Ni-mediated synthetic methods, not only aromatic carbocycles but also aromatic heterocycles could be prepared.24
Sato and co-workers reported a metalative Reppe type of acetylene cyclotrimerization.25 In this reaction, three different unsymmetrical acetylenes and one molecule of titanium species were combined together in a highly controlled manner to give aryltitanium compounds as a single isomer. As shown in Scheme 14, a dialkoxytitanacyclopentadienes 43 were prepared from two different acetylenes and a divalent titanium alkoxide reagent (η2-propene)Ti(O-i-Pr)2. Addition of ethynyl para-tolyl sulfone as the third alkyne afforded aryl titanium intermediates 44, which reacted with various electrophiles to produce a series of highly substituted benzenes 45.
Compared with the stoichiometric reactions mentioned above, the catalytic version of the selective three-component cycloaddition is highly crucial task. CpRuCl(cod)-catalyzed system was applied to chemoselective [2+2+2] cycloaddition of three different alkynes.26 An internal alkyne, a terminal alkyne and dimethyl acetylenedicarboxylate was efficiently catalyzed by Cp*RuCl(cod) to give substituted o-phthalates 46 and 48 (Scheme 15). It is critical to control the molar ratio of the three substrates to achieve high chemoselectivity.
Using semi-intramolecular strategy, selective catalytic cyclotrimerization of three unsymmetrical alkynes was realized (Scheme 16). 27 Alkynylboronates reacted with propargyl alcohol to form unsymmetrical diynes with a temporal C-B-O linkage. In the presence of Ru catalyst, cycloaddition of diynes with the third terminal alkynes afford arylboronates 50 via ruthenacycles 49 with excellent chemo- and regioselectivity. The crude arylboronates 50 underwent Suzuki–Miyaura cross-coupling with various aryl iodides in the presence of Pd-catalyst to afford a series of substituted biaryls 51 as single regioisomers. They also developed the Pd (II)-catalyzed carbonylation of the boraphthalides to establish the novel four-component coupling synthesis of substituted phthalides in good yields.28
The similar strategy was also reported with a cobalt complex.29 Use of temporary silylated tethers 52 as starting materials allowed the transformation of intermolecular reaction to intramolecular ones. Silicon-tethered triynes 52 led to the corresponding cycloadducts 53 in good yields in the presence of 5 mol% of [CpCo(CO)2]. The opening of the silylated ether was easily achieved either with n-tetrabutylammonium fluoride or with methyllithium to deliver quantitatively the benzene derivatives 54 after the reaction (Scheme 17).
5. SUMMARY
A remarkable effect of transition metals on the trimerization of alkynes was discovered in 1940's by Reppe. After that, this strategy has been developed enormously as the most powerful tool for construction benzene and the other aromatic ring skeletons. At the beginning of the history, two possible mechanisms were proposed for the [2+2+2] cyclotrimerization. One is a cyclobutadiene mechanism in which a cyclobutadiene-transition metal complex derived from two alkynes by [2+2] cycloaddition is an intermediate. The other is a metallacyclopentadiene mechanism, which is now commonly accepted from a large number of experimental results.
Recent developments of the aromatic ring construction methods by the [2+2+2] cycloaddition can be regarded as the consequence of the intermediacy of the metallacyclopentadiene. That provides an important opportunity for regioselective construction of substituted benzene rings from substituted alkynes. In fact, the controlled formation of the metallacyclopentadiene intermediate is one of the most important key points for selective construction of aromatic rings as we described above. Further development of this chemistry has still been desired because of growing importance of the aromatic compound in various areas in human society.
References
1. K. P. C. Vollhardt, Acc. Chem. Res., 1977, 10, 1; CrossRef K. P. C. Vollhardt, Angew. Chem., Int. Ed. Engl., 1984, 23, 539; CrossRef N. E. Schore, Chem. Rev., 1988, 88, 1081; CrossRef I. Ojima, M. Tzamarioudaki, Z. Li, and R. J. Donovan, Chem. Rev., 1996, 96, 635; CrossRef H.-W. Fruhauf, Chem. Rev., 1997, 97, 523; CrossRef S. Saito and Y. Yamamoto, Chem. Rev., 2000, 100, 2901; CrossRef S. Kotha, E. Brahmachary, and K. Lahiri, Eur. J. Org. Chem., 2005, 4741; CrossRef V. Gandon, C. Aubert, and M. Malacria, Chem. Commun., 2006, 2209; CrossRef P. R. Chopadea and J. Louie, Adv. Synth. Catal., 2006, 348, 2307; CrossRef B. R. Galan and T. Rovis, Angew. Chem. Int. Ed. 2009, 11, 2830; CrossRef N. Agenet, O. Buisine, F. Slowinski, V. Gandon, C. Auvert, and M. Malacria, Org. React., 2007, 68, 1; Y. Yamamoto, Cur. Org. Chem., 2005, 9, 503. CrossRef
2. G. P. Colman, G. W. Kang, W. F. Little, and M. F. Sullivan, Inorg. Chem., 1968, 7, 1298; CrossRef R. A. Ferreri and A. P. Wolf, J. Phys. Chem., 1984, 88, 2256; CrossRef M. Lautens, W. Klute, and W. Tam, Chem. Rev., 1996, 96, 49. CrossRef
3. M. F. N. N. Carvalho, F. M. T. Almeida, A. M. Galvão, and A. J. L. Pombeiro, J. Organomet. Chem., 2003, 679, 143. CrossRef
4. V. Cadierno, S. E. García-Garrido, and J. Gimeno, J. Am. Chem. Soc., 2006, 128, 15094. CrossRef
5. L. Yong and H. Butenschön, Chem. Commun., 2002, 2852. CrossRef
6. C. Breschi, L. Piparo, P. Pertici, A. M. Caporusso, and G. Vitulli, J. Organomet. Chem., 2000, 607, 57. CrossRef
7. M. Kakeya, T. Fujihara, T. Kasaya, and A. Nagasawa, Organometallics, 2006, 25, 4131. CrossRef
8. Y.-Y. Lin, S.-C. Tsai, and S. J. Yu, J. Org. Chem., 2008, 73, 4920. CrossRef
9. T. Sugihara, A. Wakabayashi, Y. Nagai, H. Takao, H. Imagawa, and M. Nishizawa, Chem. Commun., 2002, 576. CrossRef
10. G. Hilt, T. Vogler, W. Hess, and F. Galbiati, Chem. Commun., 2005, 1474; CrossRef G. Hilt, W. Hess, T. Vogler, and C. Hengst, J. Organomet. Chem., 2005, 690, 5170. CrossRef
11. N. Saino, F. Amemiya, E. Tanabe, K. Kase, and S. Okamoto, Org. Lett., 2006, 8, 1439; CrossRef A. Goswami, T. Ito, and S. Okamoto, Adv. Synth. Catal., 2007, 349, 2368. CrossRef
12. K. Tanaka, K. Toyoda, A. Wada, K. Shirasaka, and M. Hirano, Chem. Eur. J., 2005, 11, 1145. CrossRef
13. K. Yoshida, I. Morimoto, K. Mitsudo, and H. Tanaka, Chem. Lett., 2007, 36, 998. CrossRef
14. O. V. Ozerov, F. T. Ladipo, and B. O. Patrick, J. Am. Chem. Soc., 1999, 121, 7941; CrossRef O. V. Ozerov, C. P. Brock, S. D. Carr, and F. T. Ladipo, Organometallics, 2000, 19, 5016; CrossRef O. V. Ozerov, B. O. Patrick, and F. T. Ladipo, J. Am. Chem. Soc., 2000, 122, 6423; CrossRef F. T. Ladipo, V. Sarveswaran, J. V. Kingston, R. A. Huyck, S. Y. Bylikin, S. D. Carr, R. Watts, and S. Parkin, J. Organomet. Chem., 2004, 689, 502. CrossRef
15. Y. Ura, Y. Sato, M. Shiotsuki, T. Kondo, and T. Mitsudo, J. Mol. Catal. A: Chem., 2004, 209, 35. CrossRef
16. H.-T. Chang, M. Jeganmohan, and C.-H. Cheng, Chem. Commun., 2005, 4955. CrossRef
17. R. Takeuchi and Y. Nakaya, Org. Lett., 2003, 5, 3659. CrossRef
18. K. Tanaka and K. Shirasaka, Org. Lett., 2003, 5, 4697. CrossRef
19. A pioneering work in this subject, see: Y. Wakatsuki, T. Kuramitsu, and H. Yamazaki, Tetrahedron Lett. 1974, 15, 4549. CrossRef
20. E. Negishi, D. R. Swanson, F. E. Cederbaum, and T. Takahashi, Tetrahedron Lett., 1986, 27, 2829; CrossRef E. Negishi, S. J. Holmes, J. Tour, J. A. Miller, F. E. Cederbaum, D. R. Swanson, and T. Takahashi, J. Am. Chem. Soc., 1989, 111, 3336. CrossRef
21. Z. Xi, R. Hara, and T. Takahashi, J. Org. Chem., 1995, 60, 4444; CrossRef T. Takahashi, M. Kageyama, V. Denisov, R. Hara, and E. Negishi, Tetrahedron Lett., 1993, 34, 687; CrossRef T. Takahashi, Z. Xi, C. J. Rousset, and N. Suzuki, Chem. Lett., 1993, 1001. CrossRef
22. T. Takahashi, M. Kotora, and Z. Xi, J. Chem. Soc., Chem. Commun., 1995, 361; CrossRef T. Takahashi, Z. Xi, A. Yamazaki, Y. Liu, K. Nakajima, and M. Kotora, J. Am. Chem. Soc., 1998, 120, 1672; CrossRef T. Takahashi, F-Y. Tsai, and Y. Li, Chem. Lett., 1999, 1173; CrossRef Z. Xi and T. Takahashi, Huaxue Xuebao, 2000, 58, 1177; T. Takahashi, Pure Appl. Chem., 2001, 73, 271. CrossRef
23. T. Takahashi, F-Y. Tsai, Y. Li, K. Nakajima, and M. Kotora, J. Am. Chem. Soc., 1999, 121, 11093; CrossRef H. Wang, F-Y. Tsai, and T. Takahashi, Chem. Lett., 2000, 1410. CrossRef
24. L. Zhou, M. Yamanaka, K. Kanno, and T. Takahashi, Heterocycles, 2008, 76, 923. And references cited therein. CrossRef
25. D. Suzuki, H. Urabe, and F. Sato, J. Am. Chem. Soc., 2001, 123, 7925; CrossRef R. Tanaka, Y. Nakano, D. Suzuki, H. Urabe, and F. Sato, J. Am. Chem. Soc., 2002, 124, 9682. CrossRef
26. Y. Ura, Y. Sato, H. Tsujita, T. Kondo, M. Imachi, and T. Mitsudo, J. Mol. Catal. A: Chem., 2005, 239, 166. CrossRef
27. Y. Yamamoto, J. Ishii, H. Nishiyama, and K. Itoh, J. Am. Chem. Soc., 2004, 126, 3712; CrossRef Y. Yamamoto, J. Ishii, H. Nishiyama, and K. Itoh, Tetrahedron, 2005, 61, 11501. CrossRef
28. Y. Yamamoto, J. Ishii, H. Nishiyama, and K. Itoh, J. Am. Chem. Soc., 2005, 127, 9625. CrossRef
29. G. Chouraqui, M. Petit, C. Aubert, and M. Malacria, Org. Lett., 2004, 6, 1519. CrossRef