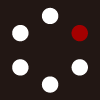
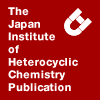
HETEROCYCLES
An International Journal for Reviews and Communications in Heterocyclic ChemistryWeb Edition ISSN: 1881-0942
Published online by The Japan Institute of Heterocyclic Chemistry
e-Journal
Full Text HTML
Received, 1st October, 2009, Accepted, 20th November, 2009, Published online, 20th November, 2009.
DOI: 10.3987/COM-09-S(S)133
■ The Mukaiyama Aldol and Mukaiyama–Michael Reactions Promoted by Commercially Available Molecular Sieves
Masahiro Anada, Takuya Washio, Yudai Watanabe, and Shunichi Hashimoto*
Laboratory of Synthetic and Industrial Chemistry, Graduate School of Life Science, Division of Life Science, Faculty of Pharmaceutical Sciences, Hokkaido University, Kita 12, Nishi 6, Kita-ku, Sapporo, Hokkaido 060-0812, Japan
Abstract
The Mukaiyama aldol reaction of silyl ketene acetals with aldehydes has been effected by using commercially available 4Å molecular sieves (4Å MS) as a promoter. Various silyl ketene acetals and silyl enol ethers have been shown to be effective nucleophiles for this reaction. For the first time, it has been found that 4Å MS can promote the Mukaiyama–Michael addition reactions of silyl ketene acetals to α,β-enones.The addition of silyl enol ethers and silyl ketene acetals to carbonyl compounds, commonly referred to as the Mukaiyama aldol reaction,1 is well recognized as one of the most powerful tools for carbon–carbon bond formation. The Mukaiyama aldol reaction can be catalyzed either by Lewis acids through the activation of carbonyl compounds or by Lewis bases through the activation of silyl enol ethers and silyl ketene acetals.2,3 It has also been shown that the Mukaiyama aldol reaction could proceed without added catalysts in some highly polar solvents such as DMF, DMSO, ionic liquids, water, and 1,8-diazabicyclo[5.4.0]undec-7-ene.4
Recently, we reported a catalytic enantioselective Mukaiyama aldol reaction of aldehydes (2) and methyl acetate-derived trimethylsilyl ketene acetal (3a) with the use of Rh2(S-BPTPI)4 (1), a dirhodium(II) carboxamidate complex that incorporates (S)-3-benzene-fused phthalimido-2-piperidinonate as chiral bridging ligands, as a chiral Lewis acid in the presence of 4Å molecular sieves (4Å MS) [eqn. (1)].5 Although high yields and enantioselectivities of up to 94% ee were observed with specific aldehydes such as benzyloxyacetaldehyde and electron-poor aromatic aldehydes, the use of most aldehydes such as benzaldehyde resulted in the production of racemic aldol adducts. Surprisingly, further studies to improve the scope and generality of this reaction revealed that 4Å MS could accelerate the Mukaiyama aldol reaction of silyl ketene acetal and benzaldehyde even more effectively than could dirhodium(II) catalyst (1) at room temperature. Although a number of examples of the Mukaiyama aldol reaction accelerated by zeolite-type mesoporous silica have been reported,6-8 there is only one example of the Mukaiyama aldol reaction promoted by commercially available molecular sieves. Scettri and co-workers reported that the solvent-free Mukaiyama aldol reaction of O-silyl dienolates derived from 2,2-dimethyl-1,3-dioxin-4-ones with aromatic aldehydes in the presence of 3Å MS (pellets, purchased from Aldrich) gave the corresponding aldol adducts, albeit in moderate yields.9 In this paper, we report that the Mukaiyama aldol and Mukaiyama–Michael reactions can proceed smoothly in the presence of commercially available molecular sieves to afford the corresponding products in good to high yields.
At the outset of this work, the aldol reaction of benzaldehyde (2a) and ethyl acetate-derived trimethylsilyl ketene acetal (3b) in the presence of commercially available pulverized 4Å MS (Junsei, 250 mg/mmol of 2a) was explored. The reaction in toluene proceeded smoothly at 23 °C to complete in less than 10 min, providing a silylated aldol adduct (5ab) in 97% yield (Table 1, Entry 1).10,11 We next evaluated the
performance of other commercially available molecular sieves. While 5Å MS (Junsei) and AW 300 MS (Aldrich) were as effective as 4Å MS (Entries 2 and 3), 3Å MS (Junsei) and 13X MS (Aldrich) were less effective than 4Å MS (Entries 4 and 5). Surprisingly, no reaction took place when powdered 4Å MS (purchased from Junsei, Aldrich, Merck, and Nacalai) was used (Entry 6). A solvent survey revealed that benzene, dichloromethane, and 1,2-dichloroethane were also suitable solvents for the present aldol reaction (Entries 7–9). The use of ether required a longer reaction time than that with toluene (Entry 10). Interestingly, the aldol reaction proceeded smoothly without a solvent, providing 5ab in high yield (Entry 11).
Using pulverized 4Å MS as a promoter and toluene as a solvent, the scope of the reaction with regard to silyl ketene acetals and silyl enol ethers was explored. Trimethylsilyl ketene acetal (3a) derived from methyl acetate and tert-butyldimethylsilyl ketene acetal (3c) derived from ethyl acetate each reacted with benzaldehyde smoothly at 23 °C to give the corresponding aldol adducts (5aa,ac) in high yields (Table 2, Entries 2 and 3). The use of pulverized 4Å MS was effective even for the sterically demanding silyl ketene acetal (3d) derived from methyl isobutyrate to afford O-silylated aldol product (5ad) in 87% yield (Entry 4). Trimethylsilyl ketene acetals (3e,f) derived from thioester and silyl enol ether (3g) could also be employed, though these reactions required heating at reflux for smooth conversion (Entries 5–7). The reaction with 1-trimethylsiloxycyclohexene (3h) proceeded at reflux to provide O-silylated aldol adduct (5ah) in good yield with no syn/anti selectivity (Entry 8).
The reaction of O-silyl dienolates (3i) derived from 2,2-dimethyl-1,3-dioxin-4-one with benzaldehyde proceeded at 23 °C to give the corresponding adduct (5ai) in 90% yield [eqn. (2)]. It is noteworthy that the yield of the reaction is very good, compared with a previous description of this type of reaction (reaction of 3i with p-anisaldehyde or p-tolualdehyde in the presence of 3Å MS) resulting in 45–50% yield.9
Using silyl ketene acetal 3b, the reaction scope with regard to the electrophilic component was next investigated (Table 3). p-Anisaldehyde (2b) led to the desired aldol adduct (5bb) in 94% yield (Entry 2). Aromatic aldehydes (2c,d) bearing electron-withdrawing groups at the para position on the benzene ring provided the aldol adducts (5cb,db) in high yields, but reaction times were significantly longer than those with 2a or 2b (Entries 3 and 4), though the mechanistic profile is unclear at this time. The present method was applicable to α,β-unsaturated aldehydes (2e,f), providing the corresponding aldol adducts (5eb,fb) in high yields (Entries 5 and 6). No evidence of the conjugate addition, commonly known as the Mukaiyama–Michael reaction, was observed.12 The reaction with aliphatic aldehydes (2g,h) proceeded uneventfully to give aldol adducts (5gb,hb) in high yields (Entries 7 and 8). Aside from aldehydes, acetophenone (2i) reacted with 3b in toluene under reflux to give the corresponding aldol adduct (5ib) in high yield (Entry 9).
As might be expected, the reaction of benzaldehyde dimethyl acetal (6) and 3b under the standard conditions proceeded cleanly to provide β-methoxy ester (7) in 97% yield [eqn. (3)].
Armed with these positive results, we then explored the 4Å MS-promoted Mukaiyama–Michael addition reaction.12,13 The addition of silyl ketene acetal (3b) to α,β-enones (8a,b) in the presence of 4Å MS in toluene proceeded at reflux to provide the corresponding silyl enol ethers (9ab,bb) in high yields [eqn. (4)]. Somewhat surprisingly, when 2-phenyl-2,3-dihydro-4H-pyran-4-one (8c) of 95% ee, prepared by the catalytic enantioselective hetero-Diels-Alder reaction employing Rh2(S-BPTPI)4 (1),5b was used as a Michael acceptor, the reaction with 3b proceeded smoothly at 23 °C to completion in less than 10 min and, after treatment with trifluoroacetic acid, gave 2,6-trans-disubstituted tetrahydropyran-4-one (10) as the sole product in 88% yield [eqn. (5)].14 This protocol represents the first example of the Mukaiyama–Michael reaction promoted by commercially available molecular sieves.
In summary, we have demonstrated that the Mukaiyama aldol reactions of aldehydes with silyl ketene acetals or silyl enol ethers can proceed smoothly in the presence of pulverized commercially available 4Å MS. This method is applicable to a wide range of aldehydes, benzaldehyde dimethyl acetal, and acetophenone, providing the corresponding aldol adducts in good to high yields. It has also been found that 4Å MS can promote the Mukaiyama–Michael addition reactions of silyl ketene acetals to α,β-enones. The present protocol based on commercially available 4Å MS offers several advantages including mild reaction conditions as well as operational simplicity and practical value. Further studies on the scope and utility of 4Å MS-promoted reactions are currently in progress.
EXPERIMENTAL
General. IR spectra were recorded on a JASCO FT/IR-4100 spectrometer and absorbance bands are reported in wavenumber (cm–1). 1H NMR spectra were recorded on a JEOL JNM-EX 270 (270 MHz) or JEOL JNM-AL 400 (400 MHz) spectrometer. Chemical shifts are reported relative to internal standard (tetramethylsilane; δH 0.00, CDCl3; δH 7.26 or C6D6; δH 7.20). Data are presented as follows: chemical shift (δ, ppm), multiplicity (s = singlet, d = doublet, t = triplet, q = quartet, m = multiplet), coupling constant and integration. 13C NMR spectra were recorded on a JEOL JNM-AL 400 (100 MHz) spectrometer. The following internal references were used (CDCl3; δ 77.0). Optical rotations were measured on a JASCO P-1030 digital polarimeter at the sodium D line (589 nm). EI-MS spetra were obtained on a JEOL JMS-T100GC spectrometer, operating with ionization energy of 70 eV. ESI-MS spectra were obtained on a Thermo Scientific Exactive spectrometer. Column chromatography was carried out on Kanto silica gel 60 N (63–210 mesh) or Wakogel® C-200 (75–150 mesh). Analytical thin layer chromatography (TLC) was carried out on Merck Kieselgel 60 F254 plates with visualization by UV light, anisaldehyde stain solution or phosphomolybdic acid stain solution.
All non-aqueous reactions were carried out in a flame-dried glassware under argon atmosphere unless otherwise noted. Reagents and solvents were purified by standard means. Dehydrated stabilizer-free CH2Cl2, toluene and THF were purchased from Kanto Chemical Co., Inc. Diisopropylamine and N,N-dimethylformamide (DMF) were distilled from calcium hydride prior to use. O-Methyl-O-trimethylsilyl ketene acetal (3a) was prepared from methyl acetate according to the procedure of Shibasaki.15 Silyl ketene acetals (3b-d) were prepared from corresponding esters according to the literature procedure.16 Trimethylsilyl ketene acetals derived from thioester (3f,g) were prepared according to the procedure of Evans.17 Silyl enol ethers (3g,h) were prepared from corresponding ketones according to the literature procedure.18 2,2-Dimethyl-6-methylene-4-trimethylsiloxy-6H-1,3-dioxine (3i) was prepared from 2,2,6-trimethyl-1,3-dioxin-4-one according to the procedure of Grunwell.19 (S)-2-Phenyl-2,3-dihydro-4H-pyran-4-one (8c) (95% ee) was prepared according to literature procedure.5b All molecular sieves were used after finely ground in mortar and activated by heating in vacuo at 220 °C for 12 h.
General procedure for the Mukaiyama aldol reaction (Table 1, Entry 1): Ethyl 3-phenyl-3-trimethylsiloxypropanoate (5ab).20 To an oven-dried flask equipped with a Teflon-coated magnetic stirring bar were added pulverized 4Å MS (50 mg, 250 mg/mmol of 2a), and a solution of benzaldehyde (2a) (21.2 mg, 0.20 mmol) in toluene (0.2 mL). A solution of 3b (48.0 mg, 0.30 mmol, 1.5 equiv.) in toluene (0.2 mL) was added to the mixture at 23 °C. After 2 h of stirring at this temperature, the reaction mixture was then filtered through a plug of Celite and the residue was washed with EtOAc (10 mL). Concentration of the combined filtrates in vacuo followed by column chromatography (silica gel, 19:1 hexane/EtOAc) provided 5ab (52.2 mg, 98%) as a colorless oil; Rf = 0.29 (19:1 hexane/EtOAc); 1H NMR (400 MHz, CDCl3) δ 0.02 (s, 9H, SiCH3), 1.25 (t, J = 7.3 Hz, 3H, CO2CH2CH3), 2.57 (dd, J = 4.0, 14.5 Hz, 1H, C2-H), 2.72 (dd, J = 9.2, 14.5 Hz, 1H, C2-H), 4.12 (q, J = 7.3 Hz, 2H, CO2CH2CH3), 5.16 (dd, J = 4.0, 9.2 Hz, 1H, C3-H), 7.22-7.36 (m, 5H, Ar).
Methyl 3-phenyl-3-trimethylsiloxypropanoate (5aa).21 According to the general procedure for the Mukaiyama aldol reaction, 5aa was prepared from benzaldehyde (2a) (21.2 mg, 0.20 mmol), 3a (43.9 mg, 0.30 mmol, 1.5 equiv.), and pulverized 4Å MS (50 mg, 250 mg/mmol of 2a). The crude product was purified by column chromatography (silica gel, 19:1 hexane/EtOAc) to provide 5aa (49.3 mg, 95%) as a colorless oil; Rf = 0.27 (19:1 hexane/EtOAc); 1H NMR (270 MHz, CDCl3) δ 0.02 (s, 9H, SiCH3), 2.59 (dd, J = 4.0, 15.1 Hz, 1H, C2-H), 2.73 (dd, J = 9.2, 15.1 Hz, 1H, C2-H), 3.69 (s, 3H, CO2CH3), 5.16 (dd, J = 4.0, 9.2 Hz, 1H, C3-H), 7.31-7.34 (m, 5H, Ar).
Ethyl 3-tert-butyldimethylsiloxy-3-phenylpropanoate (5ac).22 According to the general procedure for the Mukaiyama aldol reaction, 5ac was prepared from benzaldehyde (2a) (21.2 mg, 0.20 mmol), 3c (60.7 mg, 0.30 mmol, 1.5 equiv.), and pulverized 4Å MS (50 mg, 250 mg/mmol of 2a). The crude product was purified by column chromatography (silica gel, 19:1 hexane/EtOAc) to provide 5ac (58.6 mg, 95%) as a colorless oil; Rf = 0.31 (19:1 hexane/EtOAc); 1H NMR (400 MHz, CDCl3) δ –0.18 (s, 3H, SiCH3), 0.02 (s, 3H, SiCH3), 0.84 (s, 9H, SiC(CH3)3), 1.25 (t, J = 6.8 Hz, 3H, CO2CH2CH3), 2.53 (dd, J = 3.6, 14.5 Hz, 1H, C2-H), 2.72 (dd, J = 9.1, 14.5 Hz, 1H, C2-H), 4.13 (q, J = 6.8 Hz, 2H, CO2CH2CH3), 5.14 (dd, J = 3.6, 9.1 Hz, 1H, C3-H), 7.23-7.35 (m, 5H, Ar).
Methyl 2,2-dimethyl-3-phenyl-3-trimethylsiloxypropanoate (5ad).23 According to the general procedure for the Mukaiyama aldol reaction, 5ad was prepared from benzaldehyde (2a) (21.2 mg, 0.20 mmol), 3d (52.3 mg, 0.30 mmol, 1.5 equiv.), and pulverized 4Å MS (50 mg, 250 mg/mmol of 2a). The crude product was purified by column chromatography (silica gel, 19:1 hexane/EtOAc) to provide 5ad (48.8 mg, 87%) as a colorless oil; Rf = 0.29 (19:1 hexane/EtOAc); 1H NMR (270 MHz, CDCl3) δ –0.03 (s, 9H, SiCH3), 0.99 (s, 3H, C2-CH3), 1.12 (s, 3H, C2-CH3), 3.67 (s, 3H, CO2CH3), 4.97 (s, 1H, C3-H), 7.21-7.29 (m, 5H, Ar).
S-Ethyl 3-phenyl-3-trimethylsiloxypropanethioate (5ae).24 According to the general procedure for the Mukaiyama aldol reaction, 5ae was prepared from benzaldehyde (2a) (21.2 mg, 0.20 mmol), 3e (52.9 mg, 0.30 mmol, 1.5 equiv.), and pulverized 4Å MS (50 mg, 250 mg/mmol of 2a). The crude product was purified by column chromatography (silica gel, 19:1 hexane/EtOAc) to provide 5ae (54.2 mg, 96%) as a colorless oil; Rf = 0.34 (19:1 hexane/EtOAc); 1H NMR (270 MHz, CDCl3) δ 0.02 (s, 9H, SiCH3), 1.25 (t, J = 7.3 Hz, 3H, SCH2CH3), 2.74 (dd, J = 4.0, 14.5 Hz, 1H, C2-H), 2.89 (q, J = 7.3 Hz, 2H, SCH2CH3), 2.97 (dd, J = 9.2, 14.5 Hz, 1H, C2-H), 5.19 (dd, J = 4.0, 9.2 Hz, 1H, C3-H), 7.24-7.33 (m, 5H, Ar).
S-tert-Butyl 3-phenyl-3-trimethylsiloxypropanethioate (5af).24 According to the general procedure for the Mukaiyama aldol reaction, 5af was prepared from benzaldehyde (2a) (21.2 mg, 0.20 mmol), 3f (61.3 mg, 0.30 mmol, 1.5 equiv.), and pulverized 4Å MS (50 mg, 250 mg/mmol of 2a). The crude product was purified by column chromatography (silica gel, 19:1 hexane/EtOAc) to provide 5af (58.4 mg, 94%) as a colorless oil; Rf = 0.43 (19:1 hexane/EtOAc); 1H NMR (270 MHz, CDCl3) δ 0.03 (s, 6H, SiCH3), 1.46 (s, 9H, SC(CH3)3), 2.63 (dd, J = 4.0, 14.5 Hz, 1H, C2-H), 2.87 (dd, J = 9.2, 14.5 Hz, 1H, C2-H), 5.17 (dd, J = 4.0, 9.2 Hz, 1H, C3-H), 7.20-7.32 (m, 5H, Ar).
3-Trimethylsiloxy-1,3-diphenylpropan-1-one (5ag).25 According to the general procedure for the Mukaiyama aldol reaction, 5ag was prepared from benzaldehyde (2a) (21.2 mg, 0.20 mmol), 3g (57.7 mg, 0.30 mmol, 1.5 equiv.), and pulverized 4Å MS (50 mg, 250 mg/mmol of 2a). The crude product was purified by column chromatography (silica gel, 19:1 hexane/EtOAc) to provide 5ag (52.2 mg, 93%) as a colorless oil; Rf = 0.36 (19:1 hexane/EtOAc); 1H NMR (270 MHz, CDCl3) δ –0.05 (s, 9H, SiCH3), 3.02 (dd, J = 4.0, 15.8 Hz, 1H, C2-H), 3.57 (dd, J = 8.6, 15.8 Hz, 1H, C2-H), 5.37 (dd, J = 4.0, 8.6 Hz, 1H, C3-H), 7.22-7.58 (m, 8H, Ar), 7.94-7.97 (m, 2H, Ar).
2-[Phenyl(trimethylsiloxy)methyl]cyclohexanone (5ah).1b According to the general procedure for the Mukaiyama aldol reaction, 5ah was prepared from benzaldehyde (2a) (21.2 mg, 0.20 mmol), 3h (51.1 mg, 0.30 mmol, 1.5 equiv.), and pulverized 4Å MS (50 mg, 250 mg/mmol of 2a). The crude product was purified by column chromatography (silica gel, 1:2 hexane/toluene) to provide syn-5ah (16.1 mg, 29%) as a colorless oil and anti-5ah (22.6 mg, 41%) as a colorless oil; syn-5ah: Rf = 0.32 (1:3 hexane/toluene); 1H NMR (400 MHz, CDCl3) δ 0.02 (s, 9H, SiCH3), 1.48–1.96 (m, 6H, C3-H, C4-H and C5-H), 2.25 (m, 1H, C6-H), 2.37-2.46 (m, 2H, C2-H and C6-H), 5.31 (d, J = 4.1 Hz, 1H, C1'-H), 7.24-7.30 (m, 5H, Ar); anti-5ah: Rf = 0.18 (1:3 hexane/toluene); 1H NMR (400 MHz, CDCl3) δ –0.03 (s, 9H, SiCH3), 1.21 (m, 1H, C3-H), 1.51-1.62 (m, 2H, C3-H and C4-H), 1.68-1.78 (m, 2H, C4-H and C5-H), 1.95 (m, 1H, C5-H), 2.35-2.44 (m, 2H, C6-H), 2.70 (m, 1H, C2-H), 5.04 (d, J = 8.2 Hz, 1H, C1'-H), 7.24-7.40 (m, 5H, Ar).
6-(2-Phenyl-2-trimethylsiloxyethyl)-2,2-dimethyl-4H-1,3-dioxin-4-one (5ai).26 According to the general procedure for the Mukaiyama aldol reaction, 5ai was prepared from benzaldehyde (2a) (21.2 mg, 0.20 mmol), 3i (64.3 mg, 0.30 mmol, 1.5 equiv.), and pulverized 4Å MS (50 mg, 250 mg/mmol of 2a). The crude product was purified by column chromatography (silica gel, 3:1 hexane/EtOAc) to provide 5ai (57.7 mg, 90%) as a colorless oil; Rf = 0.29 (2:1 hexane/EtOAc); 1H NMR (270 MHz, CDCl3) δ 0.02 (s, 9H, SiCH3), 1.64 (s, 3H, CCH3), 1.67 (s, 3H, CCH3), 2.50 (dd, J = 4.6, 13.8 Hz, 1H, C4-H), 2.63 (dd, J = 7.9, 13.8 Hz, 1H, C4-H), 4.92 (q, J = 4.6, 7.9 Hz, 2H, C5-H), 5.23 (s, 1H, C2-H), 7.24-7.36 (m, 5H, Ar).
Ethyl 3-(4-methoxyphenyl)-3-trimethylsiloxypropanoate (5bb).27 According to the general procedure for the Mukaiyama aldol reaction, 5bb was prepared from p-anisaldehyde (2b) (27.2 mg, 0.20 mmol), 3b (48.0 mg, 0.30 mmol, 1.5 equiv.), and pulverized 4Å MS (50 mg, 250 mg/mmol of 2b). The crude product was purified by column chromatography (silica gel, 19:1 hexane/EtOAc) to provide 5bb (55.7 mg, 94%) as a colorless oil; Rf = 0.41 (9:1 hexane/EtOAc); 1H NMR (270 MHz, CDCl3) δ 0.03 (s, 9H, SiCH3), 1.27 (t, J = 7.3 Hz, 3H, CO2CH2CH3), 2.57 (dd, J = 4.6, 14.5 Hz, 1H, C2-H), 2.73 (dd, J = 9.2, 14.5 Hz, 1H, C2-H), 3.81 (s, 3H, ArO-CH3), 4.15 (q, J = 7.3 Hz, 2H, CO2CH2CH3), 5.12 (dd, J = 4.6, 9.2 Hz, 1H, C3-H), 6.83-6.89 (m, 2H, Ar), 7.25-7.29 (m, 2H, Ar).
Ethyl 3-(4-nitrophenyl)-3-trimethylsiloxypropanoate (5cb).5a According to the general procedure for the Mukaiyama aldol reaction, 5cb was prepared from p-nitrobenzaldehyde (2c) (30.2 mg, 0.20 mmol), 3b (48.0 mg, 0.30 mmol, 1.5 equiv.), and pulverized 4Å MS (50 mg, 250 mg/mmol of 2c). The crude product was purified by column chromatography (silica gel, 19:1 hexane/EtOAc) to provide 5cb (50.4 mg, 81%) as a colorless oil; Rf = 0.25 (9:1 hexane/EtOAc); 1H NMR (270 MHz, CDCl3) δ 0.05 (s, 9H, SiCH3), 1.25 (t, J = 7.3 Hz, 3H, CO2CH2CH3), 2.58 (dd, J = 4.6, 14.5 Hz, 1H, C2-H), 2.71 (dd, J = 8.6, 14.5 Hz, 1H, C2-H), 4.15 (q, J = 7.3 Hz, 2H, CO2CH2CH3), 5.25 (dd, J = 4.6, 8.6 Hz, 1H, C3-H), 7.53 (d, J = 8.6 Hz, 2H, Ar), 7.19 (d, J = 8.6 Hz, 2H, Ar).
Ethyl 3-[(4-trifluoromethyl)phenyl]-3-trimethylsiloxypropanoate (5db). According to the general procedure for the Mukaiyama aldol reaction, 5db was prepared from p-trifluoromethylbenzaldehyde (2d) (34.8 mg, 0.20 mmol), 3b (48.0 mg, 0.30 mmol, 1.5 equiv.), and pulverized 4Å MS (50 mg, 250 mg/mmol of 2d). The crude product was purified by column chromatography (silica gel, 19:1 hexane/EtOAc) to provide 5db (58.2 mg, 87%) as a colorless oil; TLC Rf = 0.47 (9:1 hexane/EtOAc); 1H NMR (270 MHz, CDCl3) δ 0.03 (s, 9H, SiCH3), 1.25 (t, J = 7.3 Hz, 3H, CO2CH2CH3), 2.56 (dd, J = 4.0, 14.5 Hz, 1H, C2-H), 2.70 (dd, J = 8.6, 14.5 Hz, 1H, C2-H), 4.12 (q, J = 7.3 Hz, 2H, CO2CH2CH3), 5.21 (dd, J = 4.0, 8.6 Hz, 1H, C3-H), 7.47 (d, J = 7.9 Hz, 2H, Ar), 7.59 (d, J = 7.9 Hz, 2H, Ar); 13C NMR (100 MHz, CDCl3) δ –0.11 (CH3), 14.2 (CH3), 46.0 (CH2), 60.6 (CH2), 71.3 (CH), 124.1 (q, J = 271 Hz, CF3), 125.3 (q, J = 3.8 Hz, CH), 129.6 (C), 130.0 (q, J = 12.5 Hz, C), 148.0 (C), 170.7 (C); EI-HRMS m/z calcd for C15H21F3O2Si (M+) 334.12120, found 334.12083.
Ethyl 5-phenyl-3-trimethylsiloxypent-4-ynoate (5eb). According to the general procedure for the Mukaiyama aldol reaction, 5eb was prepared from phenylpropargylaldehyde (2e) (26.0 mg, 0.20 mmol), 3b (48.0 mg, 0.30 mmol, 1.5 equiv.), and pulverized 4Å MS (50 mg, 250 mg/mmol of 2e). The crude product was purified by column chromatography (silica gel, 19:1 hexane/EtOAc) to provide 5eb (56.9 mg, 98%) as a colorless oil; Rf = 0.47 (9:1 hexane/EtOAc); 1H NMR (270 MHz, CDCl3) δ 0.15 (s, 9H, SiCH3), 1.22 (t, J = 7.3 Hz, 3H, CO2CH2CH3), 2.69 (dd, J = 5.9, 15.2 Hz, 1H, C2-H), 2.78 (dd, J = 8.6, 15.2Hz, 1H, C2-H), 4.15 (q, J = 7.3 Hz, 2H, CO2CH2CH3), 4.99 (dd, J = 4.6, 9.2 Hz, 1H, C3-H), 7.20-7.28 (m, 3H, Ar), 7.31-7.37 (m, 2H, Ar); 13C NMR (100 MHz, CDCl3) δ 0.04 (CH3), 14.2 (CH3), 43.9 (CH2), 59.8 (CH), 60.6 (CH2), 84.7 (C), 89.1 (C), 122.5 (C), 128.2 (CH), 128.3 (CH), 131.5 (CH), 170.1 (C); ESI-HRMS m/z calcd for C16H22O3NaSi (M++Na) 313.12303, found 313.12304.
Ethyl trans-5-phenyl-3-trimethylsiloxypent-4-enoate (5fb).20 According to the general procedure for the Mukaiyama aldol reaction, 5fb was prepared from trans-cinnamaldehyde (2f) (26.4 mg, 0.20 mmol), 3b (48.0 mg, 0.30 mmol, 1.5 equiv.), and pulverized 4Å MS (50 mg, 250 mg/mmol of 2f). The crude product was purified by column chromatography (silica gel, 19:1 hexane/EtOAc) to provide 5fb (55.0 mg, 94%) as a colorless oil; Rf = 0.40 (9:1 hexane/EtOAc); 1H NMR (270 MHz, CDCl3) δ 0.14 (s, 9H, SiCH3), 1.26 (t, J = 7.3 Hz, 3H, CO2CH2CH3), 2.53 (dd, J = 5.3, 14.5 Hz, 1H, C2-H), 2.62 (dd, J = 7.9, 14.5 Hz, 1H, C2-H), 4.15 (q, J = 7.3 Hz, 2H, CO2CH2CH3), 6.19 (dd, J = 6.6, 15.8 Hz, 1H, C4-H), 6.57 (d, J = 15.8 Hz, 1H C5-H), 7.20-7.39 (m, 5H, Ar).
Ethyl 5-phenyl-3-trimethylsiloxypentanoate (5gb).20 According to the general procedure for the Mukaiyama aldol reaction, 5gb was prepared from 3-phenylpropanal (2g) (26.8 mg, 0.20 mmol), 3b (48.0 mg, 0.30 mmol, 1.5 equiv.), and pulverized 4Å MS (50 mg, 250 mg/mmol of 2g). The crude product was purified by column chromatography (silica gel, 19:1 hexane/EtOAc) to provide 5gb (55.4 mg, 94%) as a colorless oil; Rf = 0.27 (19:1 hexane/EtOAc); 1H NMR (270 MHz, CDCl3) δ 0.12 (s, 9H, SiCH3), 1.26 (t, J = 7.3 Hz, 3H, CO2CH2CH3), 1.76-1.85 (m, 2H, C4-H), 2.47-2.77 (m, 4H, C2-H and C5-H), 4.10-4.24 (m, 3H, CO2CH2CH3 and C3-H), 7.16-7.31 (m, 5H, Ar).
Ethyl 4-benzyloxy-3-trimethylsiloxybutanoate (5hb).5a According to the general procedure for the Mukaiyama aldol reaction, 5hb was prepared from benzyloxyacetaldehyde (2h) (30.0 mg, 0.20 mmol), 3b (48.0 mg, 0.30 mmol, 1.5 equiv.), and pulverized 4Å MS (50 mg, 250 mg/mmol of 2h). The crude product was purified by column chromatography (silica gel, 19:1 hexane/EtOAc) to provide 5hb (57.1 mg, 92%) as a colorless oil; Rf = 0.36 (9:1 hexane/EtOAc); 1H NMR (270 MHz, CDCl3) δ 0.08 (s, 9H, SiCH3), 1.25 (t, J = 7.3 Hz, 3H, CO2CH2CH3), 2.44 (dd, J = 8.6, 15.1 Hz, 1H, C2-H), 2.60 (dd, J = 4.6, 15.1 Hz, 1H, C2-H), 3.38 (dd, J = 5.3, 9.3 Hz, 1H, C4-H), 3.45 (dd, J = 5.3, 9.2 Hz, 1H, C4-H), 4.13 (q, J = 7.3 Hz, 2H, CO2CH2CH3), 4.31 (m, 1H, C3-H) 4.53 (s, 2H, PhCH2), 7.26-7.37 (m, 5H, Ar).
Ethyl 3-phenyl-3-trimethylsiloxybutanoate (5ib).28 According to the general procedure for the Mukaiyama aldol reaction, 5ib was prepared from acetophenone (2i) (24.0 mg, 0.20 mmol), 3b (48.0 mg, 0.30 mmol, 1.5 equiv.), and pulverized 4Å MS (50 mg, 250 mg/mmol of 2i). The crude product was purified by column chromatography (silica gel, 19:1 hexane/EtOAc) to provide 5ib (48.8 mg, 87%) as a colorless oil; Rf = 0.42 (19:1 hexane/EtOAc); 1H NMR (270 MHz, CDCl3) δ 0.08 (s, 9H, SiCH3), 1.10 (t, J = 7.3 Hz, 3H, CO2CH2CH3), 1.82 (s, 3H, C4-H), 2.74 (dd, J = 5.9, 7.2 Hz, 1H, C2-H), 3.98 (q, J = 7.3 Hz, 2H, CO2CH2CH3), 7.19-7.33 (m, 3H, Ar), 7.40-7.44 (m, 2H, Ar).
Ethyl 3-methoxy-3-phenylpropanoate (7).29 According to the general procedure for the Mukaiyama aldol reaction, 7 was prepared from benzaldehyde dimethyl acetal (6) (30.4 mg, 0.20 mmol), 3b (48.0 mg, 0.30 mmol, 1.5 equiv.), and pulverized 4Å MS (50 mg, 250 mg/mmol of 6). The crude product was purified by column chromatography (silica gel, 19:1 hexane/EtOAc) to provide 7 (40.4 mg, 81%) as a colorless oil; Rf = 0.39 (9:1 hexane/EtOAc); 1H NMR (270 MHz, CDCl3) δ 1.23 (t, J = 6.6 Hz, 3H, CO2CH2CH3), 2.55 (dd, J = 4.6, 15.1 Hz, 1H, C2-H), 2.80 (dd, J = 9.2, 15.1 Hz, 1H, C2-H), 3.23 (s, 3H, OCH3), 4.12 (q, J = 6.6 Hz, 2H, CO2CH2CH3), 4.63 (dd, J = 4.6, 9.2 Hz, 1 H, C3-H), 7.27-7.39 (m, 5H, Ar).
General procedure for the Mukaiyama-Michael reaction [eqn. (4)]: Ethyl 2-(3-trimethylsiloxycyclopent-2-enyl)acetate (9ab).30 To an oven-dried flask equipped with a Teflon-coated magnetic stirring bar were added pulverized 4Å MS (50 mg, 250 mg/mmol of 8a), 2-cyclopentenone (8a) (16.4 mg, 0.20 mmol), 3b (48.0 mg, 0.30 mmol, 1.5 equiv.) and toluene (0.4 mL). The mixture was refluxed for 3 h and filtered through a plug of Celite, and the residue was washed with EtOAc (10 mL). Concentration of the combined filtrates in vacuo followed by column chromatography (Wakogel C-200, 1% Et3N in hexane) provided 9ab (45.5 mg, 94%) as a colorless oil; TLC Rf = 0.31 (2:1 hexane/EtOAc); 1H NMR (270 MHz, C6D6) δ 0.19 (s, 9H, SiCH3), 1.01 (t, J = 7.2 Hz, 3H, CO2CH2-CH3), 1.44 (m, 1H, C5’-H), 2.04 (m, 1H, C5’-H), 2.24–2.35 (m, 4H, C1-H, C4’-H and C1-H2), 3.19 (m, 1H, C4’-H), 4.03 (q, J = 7.2 Hz, 2H, CO2-CH2), 4.80 (m, 1H, C2’-H).
Ethyl 2-(3-trimethylsiloxycyclohex-2-enyl)acetate (9bb).30 According to the general procedure for the Mukaiyama-Michael reaction, 9bb was prepared from 2-cyclohexenone (8b) (19.2 mg, 0.20 mmol), 3b (48.0 mg, 0.30 mmol, 1.5 equiv.), and pulverized 4Å MS (50 mg, 250 mg/mmol of 8b). The crude product was purified by column chromatography (Wakogel C-200, 1% Et3N in hexane) to provide 9bb (48.7 mg, 95%) as a colorless oil; Rf = 0.33 (2:1 hexane/EtOAc); 1H NMR (270 MHz, C6D6) δ 0.16 (s, 9H, SiCH3), 1.15 (m, 1H, C5-H), 1.24 (t, J = 7.2 Hz, 3H, CO2CH2CH3), 1.59 (m, 1H, C6-H), 1.72-1.77 (m, 2H, C5-H and C6-H), 1.94-2.00 (m, 2H, C1-H and C4-H), 2.22 (m, 2H, EtOCOCH2), 2.64 (m, 1H, C4-H), 4.12 (q, J = 7.2 Hz, 2H, CO2CH2CH3), 4.75 (m, 1H, C2-H).
Ethyl 2-[(2S,6S)-4-oxo-6-phenyltetrahydro-2H-pyran-2-yl]acetate (10). A solution of 3b (24.1 mg, 0.15 mmol) in toluene (0.1 mL) was added to the mixture of (S)-2-phenyl-2,3-dihydro-4H-pyran-4-one (8c) (95% ee, 17.4 mg, 0.10 mmol) and 4Å MS (50 mg, 250 mg/mmol of 8c) in toluene (0.1 mL) at 23 °C. After stirring for 10 min, the reaction mixture was filtered through a plug of Celite and the residue was washed with CH2Cl2 (10 mL). A 10% solution of trifluoroacetic acid in CH2Cl2 (0.1 mL) was added to the combined filtrate, and the mixture was stirred for 10 min and poured into saturated aq. NaHCO3 solution (3 mL). The whole mixture was extracted with EtOAc (2 x 10 mL), and the combined organic layers were washed with water (3 mL) and brine (2 x 3 mL), and dried over anhydrous Na2SO4. Filtration and evaporation in vacuo furnished the crude product, which was purified by column chromatography (silica gel, 4:1 hexane/EtOAc) to provide 10 (46.4 mg, 88%) as a colorless oil; Rf = 0.28 (4:1 hexane/EtOAc); [α]D23 +37.6° (c 1.01, CHCl3); 1H NMR (400 MHz, CDCl3) δ 1.23 (t, J = 7.0 Hz, 3H, CO2CH2CH3), 2.44 (ddd, J = 1.4, 7.0, 15.0 Hz, 1H, C5-H), 2.50 (dd, J = 5.4, 14.9 Hz, 1H, C6-CH), 2.60 (ddd, J = 1.4, 4.5, 15.0 Hz, 1H, C5-H), 2.69 (dd, J = 8.2, 14.9 Hz, 1H, C6-CH), 2.83 (ddd, J = 1.4, 5.9, 14.5 Hz, 1H, C3-H), 2.92 (ddd, J = 1.4, 5.9, 14.5 Hz, 1H, C3-H), 4.15 (q, J = 7.0 Hz, 1H, CO2CH2CH3), 4.38 (m, 1H, C6-H), 5.32 (t, J = 5.9 Hz, 1H, C2-H), 7.31-7.38 (m, 5H, Ar); 13C NMR (100 MHz, CDCl3) δ 14.1 (CH3), 40.2 (CH2), 45.4 (CH2), 46.4 (CH2), 60.8 (CH2), 68.6 (CH), 74.1 (CH), 126.9 (CH), 128.2 (CH), 128.6 (CH), 139.2 (C), 170.0 (C), 206.2 (C); ESI-HRMS m/z calcd for C15H18O4 (M+) 263.12757, found 263.12779.
ACKNOWLEDGEMENTS
This research was supported in part by a Grant-in-Aid for Scientific Research from the Ministry of Education, Culture, Sports, Science and Technology, Japan. We thank S. Oka, M. Kiuchi and T. Hirose of the Center for Instrumental Analysis at Hokkaido University, for technical assistance in MS and elemental analysis.
References
1. (a) T. Mukaiyama, K. Narasaka, and K. Banno, Chem. Lett., 1973, 2, 1011; CrossRef (b) T. Mukaiyama, K. Banno, and K. Narasaka, J. Am. Chem. Soc., 1974, 96, 7503; CrossRef (c) K. Saigo, M. Osaki, and T. Mukaiyama, Chem. Lett., 1975, 4, 989; CrossRef (d) T. Mukaiyama, Org. React., 1982, 28, 203; (e) T. Mukaiyama, Angew. Chem. Int. Ed., 2004, 43, 5590. CrossRef
2. For a book and reviews, see: (a) R. Mahrwald, Chem. Rev., 1999, 99, 1095; CrossRef (b) T. D. Machajewski and C.-H. Wong, Angew. Chem. Int. Ed., 2000, 39, 1352; CrossRef (c) C. Palomo, M. Oiarbide, and J. M. García, Chem. Eur. J., 2002, 8, 36; CrossRef (d) 'Modern Aldol Reactions,' ed. by R. Mahrwald, Wiley-VCH, Weinheim, 2004.
3. For recent reviews on the enantioselective Mukaiyama aldol reactions, see: (a) S. G. Nelson, Tetrahedron: Asymmetry, 1998, 9, 357; CrossRef (b) E. M. Carreira, 'Comprehensive Asymmetric Catalysis,' ed. by E. N. Jacobsen, A. Pfaltz, and H. Yamamoto, Springer, Berlin, 1999, Vol. 3, Chapter 29.1; (c) M. Sawamura and Y. Ito, 'Catalytic Asymmetric Synthesis,' ed. by I. Ojima, Wiley-VCH, New York, 2000, Chapter 8B1; (d) E. M. Carreira, 'Catalytic Asymmetric Synthesis,' ed. by I. Ojima, Wiley-VCH, New York, 2000, Chapter 8B2; (e) S. E. Denmark and R. A. Stavenger, Acc. Chem. Res., 2000, 33, 432; CrossRef (f) J. Gawronski, N. Wascinska, and J. Gajewy, Chem. Rev., 2008, 108, 5227; CrossRef (g) M. Naodovic and H. Yamamoto, Chem. Rev., 2008, 108, 3132. CrossRef
4. (a) Y. Kita, O. Tamura, F. Itoh, H. Yasuda, H. Kishino, Y. Y. Ke, and Y. Tamura, J. Org. Chem., 1988, 53, 554; CrossRef (b) Y. Génisson and L. Gorrichon, Tetrahedron Lett., 2000, 41, 4881; CrossRef (c) T.-P. Loh, L.-C. Feng, and L.-L. Wei, Tetrahedron, 2000, 56, 7309; CrossRef (d) S.-L. Chen, S.-J. Ji, and T.-P. Loh, Tetrahedron Lett., 2004, 45, 375. CrossRef
5. (a) T. Washio, S. Nakamura, M. Anada, and S. Hashimoto, Heterocycles, 2005, 66, 567; CrossRef Rh2(S-BPTPI)4 (1) has been used to catalyze endo- and enantioselective hetero-Diels–Alder reactions of a diverse range of aldehydes with Danishefsky-type dienes as well as with monooxygenated dienes, see: (b) M. Anada, T. Washio, N. Shimada, S. Kitagaki, M. Nakajima, M. Shiro, and S. Hashimoto, Angew. Chem. Int. Ed., 2004, 43, 2665; CrossRef (c) T. Washio, R. Yamaguchi, T. Abe, H. Nambu, M. Anada, and S. Hashimoto, Tetrahedron, 2007, 63, 12037; CrossRef (d) T. Washio, H. Nambu, M. Anada, and S. Hashimoto, Tetrahedron: Asymmetry, 2007, 18, 2606; CrossRef (e) A. Boyer, G. E. Veitch, E. Beckmann, and S. V. Ley, Angew. Chem. Int. Ed., 2009, 48, 1317. CrossRef
6. For reviews on organic transformations using zeolites, see: (a) M. E. Davis, Acc. Chem. Res., 1993, 26, 111; CrossRef (b) I. W. C. E. Arends, R. A. Sheldon, M. Wallau, and U. Schuchardt, Angew. Chem. Int. Ed. Engl., 1997, 36, 1144; CrossRef (c) S. E. Sen, S. M. Smith, and K. A. Sullivan, Tetrahedron, 1999, 55, 12657; CrossRef (d) D. E. De Vos, M. Dams, B. F. Sels, and P. A. Jacobs, Chem. Rev., 2002, 102, 3615; CrossRef (e) A. Corma and H. García, Chem. Rev., 2003, 103, 4307. CrossRef
7. (a) M. Sasidharan, S. V. N. Raju, K. V. Srinivasan, V. Paul, and R. Kumar, Chem. Commun., 1996, 129; CrossRef (b) H. Matsuhashi, M. Tanaka, H. Nakamura, and K. Arata, Appl. Catal. A: General, 2001, 208, 1; CrossRef (c) H. Ishitani and M. Iwamoto, Tetrahedron Lett., 2003, 44, 299; CrossRef (d) M. Sasidharan and R. Kumar, J. Catal., 2003, 220, 326; CrossRef (e) R. Garro, M. T. Navarro, J. Primo, and A. Corma, J. Catal., 2005, 233, 342; CrossRef (f) T. R. Gaydhankar, P. N. Joshi, P. Kalita, and R. Kumar, J. Mol. Catal. A: Chem., 2007, 265, 306; CrossRef (g) R. Srivastava, J. Mol. Catal. A: Chem., 2007, 264, 146; CrossRef (h) U. S. Taralkar, P. Kalita, R. Kumar, and P. N. Joshi, Appl. Catal. A: General, 2009, 358, 88; CrossRef (i) S. Ito, H. Yamaguchi, Y. Kubota, and M. Asami, Chem. Lett., 2009, 38, 700. CrossRef
8. For selected examples of the Mukaiyama aldol reaction catalyzed by inorganic solids, see: (a) M. Onaka, R. Ohno, M. Kawai, and Y. Izumi, Bull. Chem. Soc. Jpn., 1987, 60, 2689; CrossRef (b) M. Kawai, M. Onaka, and Y. Izumi, Bull. Chem. Soc. Jpn., 1988, 61, 1237; CrossRef (c) T.-P. Loh and X.-R. Li, Tetrahedron, 1999, 55, 10789; CrossRef (d) A. Heydari, S. Khaksar, M. Sheykhan, and M. Tajbakhsh, J. Mol. Catal. A: Chem., 2008, 287, 5. CrossRef
9. M. R. Acocella, A. Massa, L. Palombi, R. Villano, and A. Scettri, Tetrahedron Lett., 2005, 46, 6141. CrossRef
10. Pulverized 4Å MS purchased from Wako, Kanto, and Aldrich displayed almost the same activity as that of pulverized 4Å MS purchased from Junsei.
11. It was found that 23 °C is the temperature limit for allowing smooth reaction.
12. (a) K. Narasaka, K. Soai, and T. Mukaiyama, Chem. Lett., 1974, 3, 1223; CrossRef (b) K. Saigo, M. Osaki, and T. Mukaiyama, Chem. Lett., 1976, 5, 163; CrossRef (c) K. Narasaka, K. Soai, Y. Aikawa, and T. Mukaiyama, Bull. Chem. Soc. Jpn., 1976, 49, 779. CrossRef
13. For selected examples of the Mukaiyama–Michael reaction without use of Lewis acids, see: (a) Y. Kita, J. Segawa, J. Haruta, T. Fujii, and Y. Tamura, Tetrahedron Lett., 1980, 21, 3779; CrossRef (b) Y. Kita, J. Segawa, J. Haruta, H. Yasuda, and Y. Tamura, J. Chem. Soc., Perkin Trans. 1, 1982, 1099; CrossRef (c) R. A. Bunce, M. F. Schlecht, W. G. Dauben, and C. H. Heathcock, Tetrahedron Lett., 1983, 24, 4943; CrossRef (d) Y. Yamamoto, K. Maruyama, and K. Matsumoto, Tetrahedron Lett., 1984, 25, 1075; CrossRef (e) B. C. Ranu, M. Saha, and S. Bhar, Tetrahedron Lett., 1993, 34, 1989; CrossRef (f) T. Nakagawa, H. Fujisawa, Y. Nagata, and T. Mukaiyama, Bull. Chem. Soc. Jpn., 2005, 78, 236. See also Ref. 6b. CrossRef
14. For selected examples of the Mukaiyama–Michael reaction between silyl ketene acetals and dihydropyranones, see: (a) Y. Yamashita, S. Saito, H. Ishitani, and S. Kobayashi, J. Am. Chem. Soc., 2003, 125, 3793; CrossRef (b) A. B. Smith, III, T. M. Razler, J. P. Ciavarri, T. Hirose, and T. Ishikawa, Org. Lett., 2005, 7, 4399; CrossRef (c) J. C. Jewett and V. H. Rawal, Angew. Chem. Int. Ed., 2007, 46, 6502. CrossRef
15. K. Oisaki, Y. Suto, M. Kanai, and M. Shibasaki, J. Am. Chem. Soc., 2003, 125, 5644. CrossRef
16. K. Mikami, S. Matsumoto, A. Ishida, S. Takamuku, T. Suenobu, and S. Fukuzumi, J. Am. Chem. Soc., 1995, 117, 11134. CrossRef
17. (a) D. A. Evans, T. Rovis, M. C. Kozlowski, C. W. Downey, and J. S. Tedrow, J Am. Chem. Soc., 2000, 122, 9134; CrossRef see also, (b) G. Simchen and W. West, Synthesis, 1977, 247. CrossRef
18. H. O. House, L. J. Czuba, M. Gall, and H. D. Olmstead, J. Org. Chem., 1969, 34, 2324. CrossRef
19. J. R. Grunwell, A. Karipides, C. T. Wigal, S. W. Heinzman, J. Parlow, J. A. Surso, L. Clayton, F. J. Fleitz, M. Daffner, and J. E. Stevens, J. Org. Chem., 1991, 56, 91. CrossRef
20. E. Nakamura, M. Shimizu, and I. Kuwajima, Tetrahedron Lett., 1976, 1699. CrossRef
21. J. K. MacLeod, K. L. Gaul, and P. B. Oelrichs, Aust. J. Chem., 1990, 43, 1533.
22. K. Saigo, M. Osaki, and T. Mukaiyama, Chem. Lett., 1975, 4, 989. CrossRef
23. M. T. Reetz and A. E. Vougioukas, Tetrahedron Lett., 1987, 28, 793. CrossRef
24. T. Mukaiyama, A. Inubushi, S. Suda, R. Hara, and S. Kobayashi, Chem. Lett., 1990, 19, 1015. CrossRef
25. Y. Naruse, J. Ukai, N. Ikeda, and H. Yamamoto, Chem. Lett., 1985, 14, 1451. CrossRef
26. B. L. Pagenkopf, J. Krüger, A. Stojanovic, and E. M. Carreira, Angew. Chem. Int. Ed., 1998, 37, 3124. CrossRef
27. L. Birkofer, A. Ritter, and H. Wieden, Chem. Ber., 1962, 95, 971. CrossRef
28. S. R. Klei, T. D. Tilley, and R. G. Bergman, Organometallics, 2002, 21, 4648. CrossRef
29. K. Saigo, M. Osaki, and T. Mukaiyama, Chem. Lett., 1976, 5, 769. CrossRef
30. T. V. RajanBabu, J. Org. Chem., 1984, 49, 2083. CrossRef