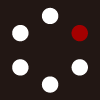
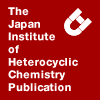
HETEROCYCLES
An International Journal for Reviews and Communications in Heterocyclic ChemistryWeb Edition ISSN: 1881-0942
Published online by The Japan Institute of Heterocyclic Chemistry
e-Journal
Full Text HTML
Received, 25th March, 2009, Accepted, 14th April, 2009, Published online, 17th April, 2009.
DOI: 10.3987/COM-09-11716
■ Synthesis of Aryltetralin Type 2-Azalignans Using Schöllkopf ’s Bislactim-Ether Methodology
Yoshihide Usami,* Masao Arimoto,* Kiyomi Kobayashi, Mikiko Honjou, Masako Yamanaka, Misaki Miyao, Hayato Ichikawa, Kenneth F. Bastow, and Kuo-Hsiung Lee
Osaka University of Pharmaceutical Sciences, 4-20-1 Nasahara, Takatsuki, Osaka 569-1094, Japan
Abstract
Synthesis of (1S,3R)-2-aza-4-deoxypodophyllotoxin has been accomplished in 6 steps using Schöllkopf’s bislactim-ether methodology in 12% total yield. Bislactim-ether, which was originally prepared from commercially available L-valine and glycine, was used as the starting material. Our synthetic route was allied to easy access to aryltetralin type 2-azalignan analogues. Synthesized 2-azalignans were tested for in vitro anticancer activity using a panel of human cancer cell lines. Both of two (1S)-diastereomers (9a and 9b) of 2-aza-4-deoxypodophyllotoxin showed significant activity against human cancer cell lines: A-549 (lung), HTC-8 (ileocecal), and MCF-7 (breast cancer).INTRODUCTION
Lignans are a large family of naturally occurring compounds constructed by oxidative dimerization of phenylpropane (C6-C3) units.1 Podophyllotoxin (1), one of the most well known toxic lignans, was originally isolated from Podophyllum sp. that are widely distributed in nature. Because of its extremely strong tubulin-protein binding activity, which is responsible for its ability to inhibit the formation of microtubules, 1 could not be used as a clinical drug.2,3 Nevertheless, it was further developed to yield such popular anticancer drugs as etoposide (3) and teniposide (4) in the 80s. The mechanism of the anticancer activity is not the inhibition of microtubule formation as observed in 1 but the inhibition of topoisomerase II.4,5 Another candidate for anti-cancer drug currently on phase II clinical trials is GL-331 (5) developed by Lee and co-workers.6 Structures of them were shown in Figure 1.
In our continuing studies of the isolation or the synthetic aspects of podophyllotoxin-related lignans including 4-dexoypodophyllotoxin (2), focusing on the Okinawan higher plant Hernandia ovigera L. that is distributed in tropical regions in Japan,7-9 we were also interested in the asymmetric synthesis of artificial aza-lignanes,10 which have gained significant attention from the end of the 1980s and are thought to be promising candidates for anticancer drug.11-25 In 1989 the synthesis of 2-azapodophyllotoxin was reported by Pearce at the first time.18 Then Vandewalle and co-workers reported the synthesis of 2-aza-4-deoxypodophyllotoxin (9a) using the Bischler-Napieralski method.19,20 Expensive L- or D-DOPA was used as chiral starting material in the literature. Another successful asymmetric synthesis of (1R,3S)-9a was reported by Koga and co-workers.21,22 A series of studies of Koga group elucidated that 9a exhibited potent citotoxicity against KB cells by inhibiting microtubule assembly but the corresponding ortho-quinone had potent DNA topoisomerase II inhibiting activity.23,24 However, their optically active amino acid starting material must be prepared by diastereomeric salt resolution between synthesized racemic amino acid derivative and natural cinchonine or by enzymatic kinetic resolution using takadiastase to the same racemic amino acid derivative26 in their elegant synthetic route. Another recent study of synthesis of azapodophyllotoxin analogues also started from L-DOPA.27 Herein we report the modified synthesis of (1S,3R)-2-aza-4-deoxypodophyllotoxin 9a from chiral starting material prepared from inexpensive L-valine and glycine via Schöllkopf’s bislactim-ether methodology. 28 2-Aza-lignans 10 and 11 as analogues of naturally occurring brusehernin29-34 (6) and polygamains35-37 (7, 8) were also synthesized. Synthesized 2-azalignans in this study were applied to biological assay against human cancer cell lines. Bioactivity of (1S,3R)-9a has not been satisfactory evaluated since (1S)-stereoisomers showed less cytotoxicity than (1R)-stereoisomers in earlier bioassay against KB cell.22
RESULTS AND DISCUSSION
Schöllkopf’s dimethyl-bislactim ether 12 was prepared from L-valine and glycine along a known method.28 The anion prepared from the bislactim-ether by treatment with n-BuLi in dry THF at −78 oC was reacted with piperonylbromide to give product 13 in 61% yield. The diastereomeric excess of this reaction was estimated to be 95% from 1H-NMR analysis. The relative configuration of major diastereoisomer 13 was supported by the observation of the shift of the signal of H-3 at δ 3.37 ppm to the higher field in the 1H-NMR spectrum, which was caused by the anisotropic effect of the cis-rearranged aromatic ring. Purified major diastereomer 13 was hydrolyzed into the methyl ester of chiral amino acid 14,38-40 which was so unstable that was transformed into the corresponding dimer 1541 when it was left at rt, as shown in Figure 2, during storage at room temperature. Thus, hydrolyzed crude 14 was treated immediately with CbzCl to form corresponding N-Cbz protected methyl ester 16 in 78% yield in 2 steps.
Protected 16 was reduced with LiAlH(OtBu)3 into alcohol 17 in 68% yield, which was then converted into oxazolidone 18 by treatment with NaH in 82% yield. This synthesis was completed by a Pictet-Spengler condensation between 18 and 3,4,5-trimethoxybenzaldehyde using the same condition as the literature22 affording (1S,3R)-2-aza-4-deoxypodophyllotoxin 9 in 46% yield with its diastereomer (2%) in our hand. Total yield of this synthesis was 12% in 6 steps from 12.
The relative stereochemistry of purified major diasteromer 9a was confirmed by NOESY analysis where cross peaks were observed between H-2/aromatic protons in the 3,4,5-trimethoxyphenyl group, H-1/H-8, and H-4a,b/H-5. The absolute configuration of the synthesized compound was confirmed as illustrated in Scheme 1 on the basis of the fact that its specific rotation had an opposite sign compared with that of known (1R,3S)-2-aza-4-deoxypodophyllotoxin. Another condition16 was examined in order to reduce minor product resulting lower yield of 9a (entry 2 in Table 1). Furthermore this synthetic route was applied to some 2-azalignane analogues of natural lignans 6−8 as described in entry 3−5 in Table 1.
Synthesized compounds in this study were applied to cytotoxicity test against three kinds of human cancer cell lines, which are A-549 (lung), HTC-8 (ileocecal), and MCF-7 (breast cancer). Results were summarized in Table 2.
2-Azalignans 9a and 9b showed strong cytotoxicity without selectivity. Others 10a, 10c, and 11b also showed week cytotocicity whereas 10b and 11a were not cytotoxic. This result suggested that what effected to cytotoxicity is not relative chemistry of 2-azalignans but substituents on the phenyl group at C-1.
In conclusion, we have developed a facile synthetic route of (1S,3R)-9a via chiral artificial amino acid derivative 13 prepared using Schöllkopf’s bislactim-ether methodology. The total yield of this synthesis from bislactim-ether 12 was 12% in 6 steps. Some applications of synthesizing 2-azalignans were also demonstrated to show our method provide easy and low-cost synthetic access. Among the synthesized compounds, 9a showed significant cytotoxicity and 9b showed more potent activity against human cancer cell lines: A549, HCT-8, and MCF-7.
EXPERIMENTAL
General. IR spectra were obtained with a Perkin Elmer FT-IR spectrometer 1720X or a JEOL FT/IR-680 Plus spectrometer. HRMS were determined using a Hitachi 4000H mass spectrometer and a JEOL JMS-700 (2) mass spectrometer. NMR spectra were recorded at 27 oC on Varian UNITY INOVA-500 and Mercury-3000 spectrometers in CDCl3 with tetramethylsilane (TMS) as internal reference. Melting points were determined on a Yanagimoto micromelting point apparatus and are uncorrected. Specific rotations were measured using a JASCO model DIP1000 digital polarimeter. Liquid column chromatography was conducted over silica gel (SILICYCLE, Silia Flash F60, mesh 230−400). Analytical TLC was performed on precoated Merck glass (Silica gel 60), and compounds were viewed by dipping the plates in an ethanol solution of phosphomolybdic acid, followed by heating. Dry THF was distilled over sodium-benzophenone ketyl under argon atmosphere.
(3S,6S)-3,6-Dihydro-3-isopropyl-2,5-dimethoxy-6-(3,4-methylenedioxy)benzylpyrazine 13
To a solution of 12 (3.06 g, 16.6 mmol) in dry THF (38 mL) was added n-BuLi (1.5 M, 12.2 mL, 18.3 mmol) at −78 oC with stirring under nitrogen atmosphere. After 15 min, a solution of piperonylbromide (4.25 g, 20.0 mmol) in THF (38 mL) was added to the reaction flask and the reaction mixture was stirred for 24 h to allow reaction to proceed at rt. The reaction mixture was treated with aq. NH4Cl, evaporated to reduce organic solvent, and extracted with Et2O. The organic layer was dried over MgSO4, filtered, and evaporated under reduced pressure to afford the crude product, which was purified by silica gel column chromatography (hexane:EtOAc = 8:1) to give 13 in 71% yield (3.78 g). The product ratio was determined to be 95% d.e. from the 1H-NMR spectrum of the crude product. 13: Colorless crystals: mp 64−69 oC; [α]D25 −84.9 (c 0.05, EtOH); IR (KBr) νmax 1700 (C=N), 1510 (C=C), 1500 (C=C) cm<συπ>−</συπ>1; 1H-NMR (CDCl3) δ 0.55 (3H, d, J = 6.8 Hz, iPr-CH3), 0.90 (3H, d, J = 6.8 Hz, iPr-CH3), 2.10 (1H, sept d, J = 6.8, 3.8 Hz, 3-CH(CH3)2), 2.94 (2H, m, -CH2Ph), 3.37 (1H, t, J = 3.3 Hz, H-3), 3.62 (3H, s, OMe), 3.64 (3H, s, OMe), 4.20 (1H, m, H-6), 5.82 (2H, m, -OCH2O-), 6.48 (1H, br d, J = 7.9 Hz, aromatic H), 6.53 (1H, br s, aromatic H), 6.59 (1H, d, J = 7.9 Hz, aromatic H); 13C-NMR (CDCl3) δ 16.9, 19.4, 31.6, 39.9, 52.3, 52.6, 56.7, 60.4, 100.5, 107.5, 110.1, 122.6, 130.6, 145.5, 146.5, 161.7, 163.2; MS m/z 319 (M+); Anal. Calcd for C17H22N2O4: C, 64.13; H, 6.97; N, 8.80. Found: C, 64.20; H, 6.97; N, 8.83.
(2R)-Methyl N-Cbz-2-amino-2-(3,4-methylenedioxy)benzylethanoate 16
To a solution of 13 (3.78 g, 11.9 mmol) in MeOH (78.7 mL) was added 0.25 N HCl aq. (104.4 mL, 26.1 mmol) at rt with stirring. After 2 days, MeOH was removed under reduced pressure to afford an aq. solution that was extracted with CHCl3. After adjusting pH to 8-10 by the addition of aq. NH3, the water layer was extracted with CH2Cl2 three times. The organic layers were combined and evaporated to afford crude residue 14, which was used in the next reaction without purification. To a reaction mixture of 14 and CbzCl (3.73 mL, 26.1 mmol) in H2O (37.2 mL) was added gradually K2CO3 (8.20 g, 59.3 mmol) at rt. After stirring for 24 h, the reaction mixture was acidified with conc. HCl and extracted with CHCl3. The organic layer was washed with brine, dried over MgSO4, filtered, and evaporated to give a crude residue, which was purified by silica gel column chromatography (EtOAc: hexane = 3:1) to give 16 in 84% yield (3.57 g). (2R)-Methyl 2-amino-2-(3,4-methylenedioxy) benzylethanoate 15: Colorless oil: [α]D25 −26.3 (c 0.02, CHCl3); IR (liquid film) νmax 3308 (N-H), 1737 (C=O), 1502 (C=C), 1490 (C=C) cm<συπ>−</συπ>1; 1H-NMR (CDCl3) δ 2.06 (2H, br s, NH2), 2.75 (1H, dd, J = 13.7, 7.7 Hz, ArCHaHb-), 2.96 (1H, dd, J = 13.7, 5.1 Hz, ArCHaHb-), 3.66 (3H, s, COOCH3 ), 3.66 (1H, overlapped, -CH(COOMe) NH2), 6.59 (1H, dd, J = 7.8, 1.5 Hz, Ar-H), 6.67 (1H, d, J = 1.5 Hz, Ar-H), 6.67 (1H, d, J = 7.8 Hz, Ar-H); 13C-NMR (CDCl3) δ 40.5, 52.2, 58.9, 100.7, 108.1, 109.2, 122.0, 130.0, 146.0, 147.2, 174.2; HREIMS m/z calcd for C11H13NO4 (M)+ 223.0845, found, 223.0840; Anal. Calcd for C19H19NO6・1/2H2O: C, 61.55; H, 4.95; N, 7.15. Found: C, 61.84; H, 4.74; N, 7.32. 16: Colorless crystals: mp 93-95 oC; [α]D25 +11.9 (c 0.02, EtOH); IR (KBr) νmax 1690 (C=O), 1643 (C=C), 1470 (C=C) cm<συπ>−</συπ>1; 1H-NMR (CDCl3) δ 2.94 (1H, dd, J = 14.0, 5.7 Hz, piperonyl-CHaHb-), 2.97 (1H, dd, J = 14.0, 5.3 Hz, piperonyl-CHaHb-), 3.66 (3H, s, COOCH3), 4.20 (1H, m, H-6), 4.55 (1H, ddd, J = 8.0, 5.7, 5.3 Hz, NHCHCOOMe(piperonyl)), 5.02 (1H, d, J = 12.3 Hz, Ph-CHaHb-O), 5.03 (1H, d, J = 12.3 Hz, Ph-CHaHb-O), 5.85 (2H, m, -OCH2O-), 6.46(1H, dd, J = 7.9, 1.8 Hz, aromatic H), 6.51 (1H, d, J = 1.8 Hz, aromatic H), 6.63 (1H, d, J = 7.9 Hz, aromatic H), 7.20-7.40 (5H, m, Ph H); 13C-NMR (CDCl3) δ 38.1, 52.5, 55.0, 67.1, 100.8, 108.1, 109.2, 122.0, 127.7, 127.8, 128.1, 128.8, 135.7, 146.2, 147.2, 155.0, 171.2; MS m/z 358 (M+); Anal. Calcd for C19H19NO6: C, 63.86; H, 5.36; N, 3.92. Found: C, 63.43; H, 5.40; N, 3.93.
(2R)-N-Cbz-2-Amino-2-(3,4-methylenedioxy)benzylethanol 17
To a suspension of LiAlH(OtBu)3 in Et2O (200 mL), which was prepared by reacting LiAlH4 (0.95 g, 25 mmol) with tBuOH (7.17 mL, 75 mmol), was added a solution of 16 in Et2O (20 mL) dropwise under nitrogen atmosphere. After stirring overnight at rt, the reaction mixture was treated with 10% H2SO4 aq. and extracted with Et2O. The organic layer was washed with brine, dried over MgSO4, filtered, and evaporated to give a crude residue, which was purified by silica gel column chromatography (EtOAc: CHCl3 = 1:9) to give 17 (2.25g, 68%). 17: Colorless crystals: mp 86−87 oC; [α]D25 +44.6 (c 0.02, EtOH); IR (KBr) νmax 3621 (OH), 3433 (NH), 1699 (C=O), 1610 (C=C), 1515 (C=C) cm<συπ>−</συπ>1; 1H-NMR (CDCl3) δ 2.70 (2H, d, J = 7 Hz, piperonyl-CH2), 3.49 (1H, dd, J = 10.6, 4.7 Hz, -CHaHb-OH), 3.59 (1H, dd, J = 10.6, 3.1 Hz, -CHaHb-OH), 3.79 (1H, m, NHCHCH2OH(piperonyl)), 4.20 (1H, m, H-6), 4.79 (1H, br s, NH), 5.00 (2H, s, -OCH2Ph), 5.85 (2H, s, -OCH2O-), 5.85 (2H, m, -OCH2O-), 6.56 (1H, br d, J = 7.9 Hz, aromatic H), 6.63 (1H, br s, aromatic H), 6.65 (1H, d, J = 7.9 Hz, aromatic H), 7.20-7.40 (5H, m, Ph-H); 13C-NMR (CDCl3) δ 37.3, 54.4, 63.9, 69.9, 100.7, 108.1, 109.3, 121.9, 127.7, 127.8, 128.1, 130.8, 135.8, 145.7, 147.2, 155.9; MS m/z 330 (M+); Anal. Calcd for C18H19NO5: C, 65.64; H, 5.82; N, 4.25. Found: C, 65.61; H, 5.79; N, 4.25.
(4R)-4-Piperonyl-1,3-oxazolidin-2-one 18
To a suspension of NaH (0.32 g, 7.98 mmol) in dry THF (66.5 mL) was added 17 (2.19 g, 6.65 mmol) in THF (5 mL) at 0 oC. After stirring for 4 h, the reaction mixture was quenched with ice water, then THF was removed under reduced pressure to afford a crude residue. After extraction with CHCl3, the organic layer was washed with brine, dried over MgSO4, filtered, and evaporated to give a crude residue, which was purified by silica gel column chromatography (EtOAc: CHCl3 = 1:1) to give 18 in 92% yield (1.36 g). 18: Colorless crystals: mp 96−98 oC; [α]D25 +55.0 (c 1.29, CHCl3); IR (KBr) νmax 3450 (NH), 1761 (C=O), 1618 (C=C), 1510 (C=C), 1500 (C=C) cm<συπ>−</συπ>1; 1H-NMR (CDCl3) δ 2.75 (1H, dd, J = 13.7, 6.4 Hz, 4-Hb), 2.80 (1H, dd, J = 13.7, 7.0 Hz, 4-Ha), 4.01 (1H, dddd, J = 8.6, 7.2, 6.4, 5.5 Hz, H-3), 4.12 (1H, dd, J = 8.6, 5.5 Hz, -CHCHaHbCOO-), 4.41 (1H, t, J = 8.6 Hz, -CHCHaHbCOO-), 5.85 (1H, s, H-1), 5.93 (2H, s, -OCH2O-), 5.99 (1H, s, NH), 6.61 (1H, dd, J = 7.7, 1.6 Hz, Ar-H), 6.64 (1H, d, J = 1.6 Hz, Ar-H), 6.75 (1H, d, J = 7.7 Hz, Ar-H); 13C-NMR (CDCl3) δ 41.3, 54.0, 69.5, 100.9, 108.4, 108.9, 121.7, 129.1, 146.2, 147.5, 158.9; MS m/z 220 (M+); Anal. Calcd for C11H11NO4: C, 59.72; H, 5.01; N, 6,33. Found: C, 59.76; H, 5.02; N, 6.32.
Synthesis of 2-Aza-lignans (Scheme 1, Table 1)
Typical procedure: To a solution of 18 (33.8 mg, 0.15 mmol) and piperonal (25.2 mg, 0.15 mmol) in CH2Cl2 (2 mL) was added trifluoromethanesulfonic acid (25.6 μL, 0.29 mmol) at 5 oC. After stirring for 24 h, the reaction mixture was quenched with aq. NaHCO3, and extracted with CH2Cl2. The organic layer was washed with brine, dried over MgSO4, filtered, and evaporated to give a crude residue, which was purified by preparative TLC (EtOAc: CH2Cl2 = 1:3) to give 11a (25.6 mg, 52% yield) and 11b (0.8 mg, 2% yield) with recovery of 18 (8.3 mg, 24%). *Diastereomers 10a and 10b were finally separated by preparative TLC using solvent system of 5% MeOH in CH2Cl2.
(1S,3R)-2-Aza-4-deoxypodophyllotoxin 9a: Colorless needles: mp 200−201 oC; [α]D25 +160.0 (c 0.05, CH3Cl); IR (KBr) νmax 1734 (C=O), 1589 (C=C) cm<συπ>−</συπ>1; 1H-NMR (CDCl3) δ 2.88 (1H, dd, J = 15.6, 10.5 Hz, 4-Hb), 2.93 (1H, dd, J = 15.6, 5.0 Hz, 4-Ha), 3.80 (6H, s, Ar-OCH3 ), 3.84 (3H, s, Ar-OCH3 ), 4.04 (1H, dddd, J = 10.5, 8.4, 5.0, 4.3 Hz, H-3), 4.11 (1H, dd, J = 8.4, 4.3 Hz, -CHCHaHbCOO-), 4.48 (1H, t, J = 8.4 Hz, -CHCHaHbCOO-), 5.85 (1H, s, H-1), 5.93 (1H, d, J = 1.5 Hz, -OCHaHbO-), 5.96 (1H, d, J = 1.5 Hz, -OCHaHbO-), 6.46 (1H, s, H-8), 6.47 (2H, s, 1-Ar-H), 6.64 (1H, s, H-5); 13C-NMR (CDCl3) δ 34.7, 48.3, 56.4, 56.6, 60.9, 68.4, 101.0, 105.6, 108.0, 108.2, 125.2, 126.3, 137.1, 137.3, 146.2, 146.6, 152.7, 155.9; HREIMS m/z calcd for C21H21N1O2 (M) + 399.1318, found, 399.1312.
(1R,3R)-2-Aza-4-deoxypodophyllotoxin 9b: Colorless crystals: mp 239−243 oC; [α]D22 +49.5 (c 0.02, CH3Cl); IR (KBr) νmax 1761 (C=O), 1593 (C=C) , 1486 (C=C) cm<συπ>−</συπ>1; 1H-NMR (CDCl3) δ 2.96 (1H, dd, J = 14.9, 4.1 Hz, 4-Hb), 3.01 (1H, dd, J = 14.9, 10.2 Hz, 4-Ha), 3.80 (3H, s, Ar-OCH3 ), 3.82 (6H, s, Ar-OCH3 ), 4.05 (1H, dd, J = 11.0, 7.8 Hz, -CHCHaHbCOO-), 4.10 (1H, m, H-3), 4.56 (1H, dd, J = 7.8, 6.5 Hz, -CHCHaHbCOO-), 5.49 (1H, s, H-1), 5.90 (1H, d, J = 1.4 Hz, -OCHaHbO-), 5.94 (1H, d, J = 1.4 Hz, -OCHaHbO-), 6.50 (2H, s, Ar-H-2’), 6.53 (1H, s, H-8), 6.62 (1H, s, H-5); 13C-NMR (CDCl3) δ 34.2 (t), 54.6 (d), 56.1 (q), 59.7 (d), 60.8(q), 68.3 (d), 101.3 (t), 104.7 (d), 108.0 (d), 108.6 (d), 124.2 (s), 130.0 (s), 137.5 (s), 138.0 (s), 146.8 (s), 147.2 (s), 153.2 (s), 156.8 (s); HRMS m/z calcd for C21H21N1O7 (M)+ 369.1218, found 399.1317.
(1S,3R)-2-Azabursehernin 10a: Colorless crystals: mp 225−228 oC; [α]D25 +71.8 (c 1.00, CH3Cl); IR (KBr) νmax 1729 (C=O), 1503, 1488 (C=C) cm<συπ>−</συπ>1; 1H-NMR (CDCl3) δ 2.88 (1H, dd, J = 15.5, 10.3 Hz, 4-Hb), 2.92 (1H, dd, J = 15.5, 5.0 Hz, 4-Ha), 3.85 (3H, s, Ar-OCH3 ), 3.86 (3H, s, Ar-OCH3 ), 4.02 (1H, dddd, J = 10.3, 8.2, 5.0, 4.3 Hz, H-3), 4.10 (1H, dd, J = 8.4, 4.3 Hz, -CHCHaHbCOO-), 4.45 (1H, dd, J = 8.4, 8.2 Hz, -CHCHaHbCOO-), 5.88 (1H, s, H-1), 5.93 (1H, d, J = 1.4 Hz, -OCHaHbO-), 5.95 (1H, d, J = 1.4 Hz, -OCHaHbO-), 6.45 (1H, s, H-8), 6.63 (1H, s, H-5), 6.60 (1H, dd, J = 8.2, 2.1 Hz, H-6’), 6.77 (1H, d, J = 8.2 Hz, H-5’), 6.93 (1H, d, J = 2.1 Hz, H-2’); 13C-NMR (CDCl3) δ 34.7 (t), 47.9 (d), 55.9 (q), 56.0 (q), 56.03 (d), 68.4 (t), 101.1 (t), 108.26 (d), 108.35 (d), 110.8 (d), 112.0 (d), 120.6 (d), 125.6 (s), 127.0 (s), 134.6 (s), 146.7 (s), 147.0 (s), 148.8 (s), 149.1 (s), 156.5 (s); HRMS m/z calcd for C20H19N1O6 (M)+ 369.1212, found 369.1214.
(1R,3R)-2-Azabursehernin 10b: Oil; [α]D22 +21.9 (c 0.03, CH3Cl); IR (liquid film) νmax 1757 (C=O), 1478 (C=C) cm<συπ>−</συπ>1; 1H-NMR (CDCl3) δ 2.95 (1H, dd, J = 14.6, 3.4 Hz, 4-Hb), 3.01 (1H, dd, J = 14.6, 10.3 Hz, 4-Ha), 3.83 (3H, s, Ar-OCH3 ), 3.84 (3H, s, Ar-OCH3 ), 4.03 (1H, ddd, J = 10.8, 7.9, Hz, -CHCHaHbCOO-), 4.11 (1H, m, H-3), 4.54 (1H, dd, J = 7.9, 6.9 Hz, -CHCHaHbCOO-), 5.30 (1H, s, H-1), 5.88 (1H, d, J = 1.4 Hz, -OCHaHbO-), 5.92 (1H, d, J = 1.4 Hz, -OCHaHbO-), 6.50 (1H, s, H-8), 6.62 (1H, s, H-5), 6.67 (1H, d, J = 8.2 Hz, H-5’), 6.80 (1H, d, J = 2.0 Hz, H-2’), 6.84 (1H, dd, J = 8.2, 2.0 Hz, H-6’); HRMS m/z calcd for C20H19N1O6 (M)+ 369.1212, found 369.1205. *Satisfied signals could not be obtained in the 13C-NMR spectrum since of shortage of material.
(1S,3R)-10c: Colorless crystals: mp 203−206 oC; [α]D22 +181.9 (c 0.18, CH3Cl); IR (KBr) νmax 1762 (C=O), 1502 (C=C), 1487 (C=C) cm<συπ>−</συπ>1; 1H-NMR (CDCl3) δ 2.85 (1H, dd, J = 15.5, 5.2 Hz, H-4A), 2.89 (1H, br dd, J = 15.5, 10.3 Hz, H-4B), 3.73 (3H, s, Ar-OCH3 ), 3.75 (3H, s, Ar-OCH3 ), 4.11 (1H, dd, J = 8.5, 3.0 Hz, -CHCHaHbCOO-), 4.18 (1H, m, H-3), 4.44 (1H, dd, J = 8.5, 7.8 Hz, -CHCHaHbCOO-), 5.90 (2H, s, -OCH2O-), 6.14 (1H, s, H-1), 6.38 (1H, s, H-8), 6.60 (1H, s, H-5), 6.63 (1H, d, J = 3.0 Hz, H-2’), 6.80 (1H, dd, J = 8.7, 3.0 Hz, H-6’), 6.86 (1H, d, J = 8.7 Hz, H-5’); 13C-NMR (CDCl3) δ 34.2 (t), 49.3 (d), 52.2 (d), 55.7 (q), 56.5 (q), 68.0 (t), 101.0 (t), 107.5 (d), 108.4 (d), 112.7 (d x 2), 116.8 (d), 125.3 (s), 128.1 (s), 131.8 (s), 146.6 (s), 146.7 (s), 151.5 (s), 153.4 (s), 156.7 (s); HRMS m/z calcd for C20H19N1O6 (M)+ 369.1212, found 369.1209.
(1S,3R)-2-Azapolygamain 11a: Colorless needles: mp 165−167 oC; [α]D25 +150.6 (c 1.28, CH3Cl); IR (KBr) νmax 1742 (C=O), 1590, 1517, 1501, 1483 (C=C) cm<συπ>−</συπ>1; 1H-NMR (CDCl3) δ 2.86 (1H, dd, J = 15.5, 10.5 Hz, 4-Hb), 2.91 (1H, dd, J = 15.5, 5.0 Hz, 4-Ha), 4.03 (1H, dddd, J = 10.5, 8.3, 5.0, 4.3 Hz, H-3), 4.09 (1H, dd, J = 8.7, 4.3 Hz, -CHCHaHbCOO-), 4.45 (1H, dd, J = 8.7, 8.3 Hz, -CHCHaHbCOO-), 5.83 (1H, s, H-1), 5.92 (1H, d, J = 1.4 Hz, -OCHHO-), 5.93 (2H, d, J = 1.4 Hz, -OCHHO-), 5.94 (1H, d, J = 1.4 Hz, -OCHHO-), 6.42 (1H, s, H-8), 6.61 (1H, s, H-5), 6.71 (1H, d, J = 1.8 Hz, H-2’), 6.75 (1H, d, J = 8.0 Hz, H-5’), 6.78 (1H, dd, J = 8.0, 1.8 Hz, H-6’); 13C-NMR (CDCl3) δ 34.3 (t), 47.9 (d), 56.0 (d), 68.4 (t), 101.13 (t), 101.15 (t), 108.1 (d), 108.2 (d), 108.3 (d), 108.8 (d), 122.2 (d), 125.5 (s), 127.0 (s), 135.9 (s), 146.8 (s), 147.1 (s), 147.3 (s), 147.8 (s), 156.4 (s); HRMS m/z calcd for C19H15N1O6 (M)+ 353.0899, found 353.0894.
(1R,3R)-2-Azapolygamain 11b: Oil; [α]D25 +66.1 (c 0.03, CH3Cl); IR (liquid film) νmax 1757 (C=O), 1511, 1487 (C=C) cm<συπ>−</συπ>1; 1H-NMR (CDCl3) δ 2.95 (1H, dd, J = 14.8, 3.4 Hz, 4-Hb), 3.01 (1H, dd, J = 14.8, 10.4 Hz, 4-Ha), 4.03 (1H, dd, J = 10.8, 7.6 Hz, -CHCHaHbCOO-), 4.10 (1H, dddd, J = 10.8, 10.4, 6.7, 3.4 Hz, H-3), 4.54 (1H, dd, J = 7.6, 6.7 Hz, -CHCHaHbCOO-), 5.48 (1H, s, H-1), 5.87 (1H, d, J = 1.4 Hz, -OCHHO-), 5.90 (1H, d, J = 1.6 Hz, -OCHHO-), 5.91 (1H, d, J = 1.6 Hz, -OCHHO-), 5.92 (1H, d, J = 1.4 Hz, -OCHHO-), 6.48 (1H, s, H-8), 6.60 (1H, s, H-5), 6.66 (1H, d, J = 1.6 Hz, H-2’), 6.74 (1H, d, J = 7.6 Hz, H-5’), 6.86 (1H, dd, J = 7.6, 1.6 Hz, H-6’); 13C-NMR (CDCl3) δ 34.2 (t), 54.4 (d), 59.3 (d), 68.2 (t), 101.1 (t), 101.3 (t), 108.5 (d), 108.0 (d), 108.1 (d), 108.5 (d), 121.5 (d), 124.1 (s), 130.0 (s), 136.4 (s), 146.8 (s), 147.0 (s), 147.2 (s), 147.8 (s), 156.8 (s); HRMS m/z calcd for C19H15N1O6 (M)+ 353.0899, found 353.0903.
Cytotoxic Activity Assay42
All stock cultures were grown in T-25 flask. Freshly trypsinized cell suspensions were seeded in 96-well microtiter plates at densities of 1500-7500 cells per well with compounds added from DMSO-diluted stock. After 3 days in culture, attached cells were fixed with cold trichloroacetic acid and then stained with 0.4% sulforhodamine B. The absorbency at 562 nm was measured using a microplate reader after solubilizing the bound dye. The mean ED50 is the concentration of agent that reduces cell grows by 50% under the experimental conditions and is the average of two independent determinations that were reproducible and statistically significant. The following human tumor cell lines were used in the assay: A549 (human lung carcinoma), HCT-8 (colon adenocarcinoma), and MCF-7 (breast cancer). All cell lines were obtained from the Lineberger Cancer Center (UNC-CH) or ATCC (Rockville, MD) and were cultured in RPMI-1640 medium supplemented with 25 mM HEPES, 0.25% sodium bicarbonate, 10% fetal bovine serum, and 100μg/mL kanamycin.
ACKNOWLEDGEMENTS
We are grateful to Dr. K. Minoura, Ms. M. Fujitake and Ms. S. Seki of this University for NMR, MS measurements, and elemental analysis respectively. This work was supported in part by a Grant-in-Aid for “High-Tech Research Center” Project for Private Universities: matching fund subsidy from MEXT Ministry of Education, Culture, Sports, Science, and Technology; 2002−2006, Japan (M. Arimoto) and in part by NIH grant CA 17625 (K. H. Lee).
This paper is dedicated to the memory of late Honorary Professor Hideo Yamaguchi of Osaka University of Pharmaceutical Sciences, who passed away on 22nd January 2009.
References
1. M. Saleem, H. J. Kim, M. S. Ali, and Y. S. Lee, Nat. Prod. Rep., 2005, 22, 696. CrossRef
2. C. Canel, R. M. Moraes, F. E. Dayan, and D. Ferreira, Phytochemistry, 2000, 54, 115. CrossRef
3. J. C. Paulson and W. O. McClure, Annals of the New York Academy of Sciences, 1975, 253 (Biol. Cytoplasmic Microtubules, Pap. Conf., 1974), 517.
4. E. L. Baldwin and N. Osheroff, Current Medicinal Chemistry: Anti-Cancer Agents, 2005, 5, 363. CrossRef
5. V. Srivastava, A. S. Negi, J. K. Kumar, M. M. Gupta, and S. P. S. Khanuja, Bioorg. Med. Chem., 2005, 13, 5892. CrossRef
6. Z. Xiao, J. R. Vance, K. F. Bastow, A. Brossi, H. K. Wang, and K. H. Lee, Bioorg. Med. Chem., 2004, 12, 3363. CrossRef
7. M. Arimoto, H. Yamaguchi, and S. Nishibe, Studies in Natural Products Chemistry, 1996, 18 (Stereoselective Synthesis (Part K)), 551.
8. M. Arimoto, S. Matsuura, C. Muro, H. Tsujibo, E. Matsumura, H. Yamaguchi, and Y. Inamori, Biosci. Biotech. Biochem., 1994, 58, 189. CrossRef
9. M. Tanoguchi, E. Hosono, M. Kitaoka, M. Arimoto, and H. Yamaguchi, Chem. Pharm. Bull., 1991, 39, 1873. CrossRef
10. M. Arimoto, A. Miyamoto, H. Nakayama, T. Okano, H. Miyoshi, A. Iwai, M. Yamanaka, F. Hirano, A. Ohguro, H. Ichikawa, and Y. Usami, Bull. Osaka Univ. Pharm. Sci., 2009, 3, 39.
11. S. Tu, Y. Zhang, B. Jiang, R. Jia, J. Zhang, J. Zhang, and S. Ji, Synthesis, 2006, 22, 3874. CrossRef
12. Y. Hitotsuyanagi, M. Fukuyo, K. Tsuda, M. Kobayashi, A. Ozeki, H. Itokawa, and K. Takeya, Bioorg. Med. Chem. Lett., 2000, 10, 315. CrossRef
13. A. Legrand, B. Rigo, P. Gautret, J.-P. Henichart, and D. Couturier, J. Heterocycl. Chem., 1999, 36, 1263. CrossRef
14. R. Katritzky, J. Cobo-Domingo, B. Yang, and P. J. Steel, Tetrahedron: Asymmetry, 1999, 10, 255. CrossRef
15. Y. Hitotsuyanagi, M. Kobayashi, K. Takeya, and H. Itokawa, J. Chem. Soc., Perkin Trans. 1, 1995, 11, 1387. CrossRef
16. Y. Hitotsuyanagi, Y. Ichihara, K. Takeya, and H. Itokawa, Tetrahedron Lett., 1994, 35, 9401. CrossRef
17. P. Lienard, J. C. Quirion, and H. P. Husson, Tetrahedron, 1993, 49, 3995. CrossRef
18. H. L. Pearce, N. J. Bach, and T. L. Cramer, Tetrahedron Lett., 1989, 30, 907. CrossRef
19. J. Van der Eycken, J. P. Bosmans, D. Van Haver, M. Vandewalle, A. Hulkenberg, W. Veerman, and R. Nieuwenhuizen, Tetrahedron Lett., 1989, 30, 3873. CrossRef
20. J. P. Bosmans, J. Van der Eycken, M. Vandewalle, A. Hulkenberg, R. Van Hes, and W. Veerman, Tetrahedron Lett., 1989, 30, 3877. CrossRef
21. K. Tomioka, Y. Kubota, and K. Koga, Tetrahedron Lett., 1989, 30, 2953. CrossRef
22. K. Tomioka, Y. Kubota, and K. Koga, Tetrahedron, 1993, 49, 1891. CrossRef
23. A, Iida, M. Kano, Y. Kubota, K. Koga, and K. Tomioka, Bioorg. Med. Chem. Lett., 1997, 7, 2565. CrossRef
24. A. Iida, M. Kano, Y. Kubota, K. Koga, and K. Tomioka, Chem. Pharm. Bull., 2000, 48, 486. CrossRef
25. H. Itokawa, Y. Hitotsuyanagi, and K. Takeya, Heterocycles, 1992, 33, 537. CrossRef
26. S. Yamada, T. Fujii, and T. Shioiri, Chem. Pharm. Bull., 1962, 10, 680. CrossRef
27. E. Marnantoni, M. Petrini, and R. Profeta, Tetrahedron Lett., 2004, 45, 2133. CrossRef
28. U. Schöllkopf, U. Groth, and C. Deng, Angew. Chem., Int. Ed. Engl., 1981, 20, 798. CrossRef
29. P. B. McDoniel and J. R. Cole, J. Pharm. Sci., 1972, 61, 1992. CrossRef
30. H. Yamaguchi, M. Arimoto, K. Yamamoto, and A. Numata, Yakugaku Zasshi, 1979, 99, 674.
31. H. Yamaguchi, M. Arimoto, M. Tanoguchi, T. Ishida, and M. Inoue, Chem. Pharm. Bull., 1982, 30, 3212. CrossRef
32. P. Richomme, J. Bruneton, P. Cabalion, and M. M. Debray, J. Nat. Prod., 1984, 47, 879. CrossRef
33. M. C. Chalandre, C. Pareyre, and J. Bruneton, Annal. Pharm. Francaises, 1984, 42, 317.
34. A. Koulman, M. E. Kubbinga, S. Batterman, H. J. Woerdenbag, N. Pras, J. G. Woolley, and W. J. Quax, Planta Med., 2003, 69, 733. CrossRef
35. G. C. Hokanson, J. Nat. Prod., 1979, 40, 378. CrossRef
36. G. J. Provan and P. G. Waterman, Planta Med., 1985, 271. CrossRef
37. B. Gözler, T. Gözler, G. Saglam, and M. Hesse, Phytochemistry, 1996, 42, 689. CrossRef
38. L. Ollelo, L. Castero, and D. Dominguez, Tetrahedron, 1999, 55, 4445. CrossRef
39. G. Guillena and C. C. Nájera, J. Org. Chem., 2000, 65, 7310. CrossRef
40. Y. N. Belokon, N. B. Bespalova, T. D. Churkina, I. Císařová, M. G. Ezernitskaya, S. R. Harutyunyan, R. Hrdina, H. B. Kagan, P. Kočovský, K. A. Kochetkov, O. V. Larionov, K. A. Lyssenko, M. North, M. Polášek, A. S. Peregudov, V. V. Prisyazhnyuk, and Š. Vyskočil, J. Am. Chem. Soc., 2003, 125, 12680. CrossRef
41. V. Deulofeu and J. Mendive, Z. Physiol. Chem., 1932, 211, 1. CrossRef
42. Y. Nakanishi, F.-R. Chang, C.-C. Liaw, Y.-C. Wu, K. F. Bastow, and K.-H. Lee, J. Med. Chem., 2003, 46, 3185 CrossRef