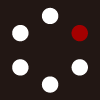
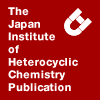
HETEROCYCLES
An International Journal for Reviews and Communications in Heterocyclic ChemistryWeb Edition ISSN: 1881-0942
Published online by The Japan Institute of Heterocyclic Chemistry
e-Journal
Full Text HTML
Received, 26th March, 2009, Accepted, 14th May, 2009, Published online, 15th May, 2009.
DOI: 10.3987/COM-09-11718
■ The Reactions of N3P3Cl4[NH(CH2)3NMe] with Difunctional Fluoro Alcohols
Esra Tanrıverdi, Adem Kılıç, Serpil Harbeck, and Gönül Yenilmez Çiftçi*
Department of Chemistry, Gebze Institute of Technology, Gebze 41400, Kocaeli, Turkey
Abstract
The reactions of N3P3Cl4[NH(CH2)3NMe] (1) in one stoichiometry (1:1) with the sodium derivatives of the fluorinated diols 2,2,3,3-tetrafluorobutane-1,4-diol (2) and 2,2,3,3,4,4-hexafluoropentane-1,5-diol (3) in THF solution at room temperature yielded the following five products, whose structures (4-8) have been characterized by elemental analysis, mass spectrometry, FT-IR, 1H and 31P NMR spectroscopy: the mono-spiro compound, N3P3Cl2[NH(CH2)3NMe](OCH2CF2CF2CH2O) (4), a di-spiro derivative N3P3[NH(CH2)3NMe](OCH2CF2CF2CH2O)2 (6) and the mono-spiro compound, N3P3Cl2[NH(CH2)3NMe](OCH2CF2CF2CF2CH2O) (5), its ansa isomer (8), a di-spiro derivative N3P3[NH(CH2)3NMe](OCH2CF2CF2CF2CH2O)2 (7). Besides, the thermal properties of the compounds (1, 4-8) were investigated by thermal analysis, namely by DSC and TGA.INTRODUCTION
The reactions of phosphazenes with difunctional alcohols are widely studied.1-4 The four types of products derived from the reactions of cyclotriphosphazenes with diols (spiro, ansa, open chain, and bridged compounds) are well established.5 Reaction of N3P3Cl6 (trimer) with 1,2-ethane-,1,3-propane- and 1,4-butane-diols (in the presence of pyridine to neutralize the HCl formed) predominantly gave spiro derivatives with 5-, 6-, 7-membered phosphate rings, respectively, whereas ansa derivatives were obtained only in small yields.6 A bridged derivative was observed as a minor product with butanediol, indicating that chain length was a contributing factor in determining derivative-type.5 It was also found that ansa-derivatives are formed using sodium salts of diols as reagents in polar solvents such as THF.7,8 Reaction of N3P3Cl6 with amino-alcohols, diamines and their -NMe derivatives showed a preference for spiro-derivatives, when the linking –(CH2)n– group of the reagent consisted of n = 2,3 moieties.9 Bridged compounds are formed by the reactions of N3P3Cl6 with diamines of increasing chain length (n= 4, 5).10 Guerch et al.11 reported that for the forming of bridged compounds one needs at least chain lengths of five (n>5). The reactions of a trimer with the sodium derivative of the fluorinated diol 2,2,3,3-tetrafluorobutane-1,4-diol (2) and 2,2,3,3,4,4-hexafluoropentane-1,5-diol (3) yield mono-spiro, di-spiro, tri-spiro, ansa, di-ansa and spiro-ansa products.6 The chemical and physical properties of phosphazenes change with the substituted side groups. So, it is possible to design materials with special properties such as inflammable textile fibers and advanced elastomers,12 anticancer agents,13-15 hydraulic fluids and lubricants,16,17 electrical conductivity,18 fertilizers,19,20 and flame retardant properties.21,22 Over the past two decades, a considerable amount of research has been devoted to the synthesis and analysis of cyclic phosphazene trimers substituted with fluorinated groups as lubricants for magnetic recording media,23-27 for example computer hard disks, video and computer tapes, etc.
The reactions of (1) with 2,2,3,3-tetrafluorobutane-1,4-diol (2) and 2,2,3,3,4,4-hexafluoropentane-1,5-diol (3) giving compounds (4, 5, 6, 7, 8), respectively, (Scheme 1) is presented in this work. The new compounds (4-8) have been characterized by elemental analysis, mass spectrometry, FT-IR, 1H and 31P NMR spectroscopy. The thermal properties of the compounds (4-8) were investigated by DSC and TGA.
RESULTS AND DISCUSSION
NMR Spectra
Each of the compounds was characterized by 1H and 31P NMR spectroscopy. The 1H NMR results are provided as part of the analytical data in the synthesis section for each new compound and the 31P NMR chemical shifts and phosphorus–phosphorus coupling constants are summarized in Table 1.
In the proton decoupled 31P NMR spectrum of (4 and 5) peaks at 26.7 and 26.5 ppm were observed (Table 1) which are corresponding to the PCl2 groups of fluoroalkoxide substituted phosphazene. These findings are in agreement with the literature.6,28 Looking at the NMR spectra one can see that the chemical shift of the phosphorous atoms of the spiro-ring depends on the nature of substituent. For example, when the substituted spiro group, such as [-OCH2(CF2)nCH2O-)], where n=2,3 chemical shift between 15.0-21.3 ppm is observed in the 31P NMR spectrum, depending on the degree and type of the substitutent. PCl(R) group of compound (8) give a signal at 25.1 ppm in the proton decoupled 31P NMR spectrum. Two methods were used to quantify the products of the reactions of compounds (1) with various nucleophiles, one method compares the amounts of compounds that were isolated and purified, whereas the other method quantifies the relative amounts of each compound from the proton-decoupled 31P NMR spectrum of the reaction mixture (Table 2). Compound (1) and (2) reacted to the compounds (4) and (6). In Figure 1 is shown the proton-decoupled 31P NMR spectrum of the reaction mixture (Figure 1a), obtained by the reaction of (1) and 2,2,3,3-tetrafluorobutane-1,4-diol (2), as well as the proton-decoupled 31P NMR spectra of the isolated and separated compounds (4) (Figure 1b) and (6) (Figure 1c). The reaction of compound (1) with 2,2,3,3,4,4-hexafluoropentane-1,5-diol (3) resulted mono-spiro (5), di-spiro (7) and mono-ansa (8) products (Figure 2). In the spectrum of reaction mixture (2a) we observed four groups of the peaks belonging to starting material (1), mono-spiro, di-spiro and mono-ansa products (chemical shifts of the all compounds are given in Table 1). The amount of the mono-spiro, di-spiro and mono-ansa products are given in Table 2. Generally, the yield rates obtained from the 31P NMR of the reaction mixture are higher than the calculated yields obtained after the isolation and purification of the products. It is likely that the quantiation of reaction products in the reaction mixture by 31P NMR spectroscopy is more reliable than that after the isolation procedure, because the only sample preparation for the NMR investigation was to filter the reaction mixture, evaporate off the solvent under vacuum and dissolve the residue in CDCl3 solution, whereas, during the chemical separation and purification procedure some material remains in the chromatographic column used for the separation of the individual compounds from the reaction mixture and is lost during purification processes (recrystallization etc.).
Thermal Analysis
The aim of the thermal analysis study was to investigate the influence of the degree and the type of the substituent on the thermal stability of the molecules. Therefore, in this study the unsubstituted trimer, compound (1), the mono-spiro compounds (4 and 5), the di-spiro compounds (6 and 7), and the mono-ansa compound (8) were chosen for the comparison concerning to the thermal properties of the molecules.
The significant data obtained by the thermal analysis methods (TGA/DSC) are given in table 3. The melting points (Tm) of the compounds are determined using the DSC technique. The DSC data show that the melting points of the compounds increase with the substitution. Looking on the melting points of the all compounds, one can see that the mono-spiro compounds (4 and 5) show lower melting points (124 oC and 130 oC) than the di- substituted and mono-ansa substituted compounds. The lowering of the melting point might be due to the presence of H-bonding seen in the FT-IR spectra. Similar effects were observed also in other study.29 The TGA of compounds was recorded between RT and 1000 oC under 50 ml/min N2 flow. It was found that the starting point of the decomposition temperature (Td) of the mono substituted compounds (4 and 5) is different from the decomposition of di-substituted ones. Comparing the onset temperature (Ton) of these molecules, the mono substituted compounds (4 and 5) are decomposing faster than the di-substituted ones, besides, the mono substituted analogues decomposes in several steps. In the thermogram of the compound (5) a loss of mass (6.86 %) is observed at around 70 oC correspond to the loss of 2 mol of water. The presence of the water in the molecule is confirmed by the FT-IR spectrum. The decomposition of mono-ansa compound (8) started already at 168 oC and ended at 268 oC as expected when one compared this with the mono substituted analogues (4 and 5), since the ring stress in mono-ansa compound (8) is higher than the mono substituted (4 and 5) ones.
To resume, in this study, different derivatives of the spiro and ansa cyclotriphosphazenes containing different number of fluoro atoms were successfully synthesized and characterized using various spectroscopic techniques. Additionally, thermal properties of the compounds were determined using thermal analysis methods like TGA and DSC.
EXPERIMENTAL
1. Materials
Hexachlorocyclotriphosphazene (Otsuka Chemical Co. Ltd) was purified by fractional crystallization from hexane. Sodium hydride, 60 % dispersion in mineral oil (Merck), the latter removed by washing with dry heptane (Merck) followed by decantation. Hexane, heptane, ethylacetate, dichloromethane (Merck), 2,2,3,3-tetrafluoro-1,4-butanediol and 2,2,3,3,4,4-hexafluoropentane-1,5-diol, N-methyl-1,3-propane-di amine (Aldrich) were used as received. THF was distilled over a sodium–potassium alloy under an atmosphere of dry argon. All reactions were performed under a dry argon atmosphere. For column chromatography silica gel (230–400 mesh Merck) was used.
2. Methods
Elemental analyses were obtained using a Carlo Erba 1106 Instrument. Mass spectra were recorded on a Bruker MicrOTOF LC-MS spectrometer using the electrospray ionization (ESI) method; 35Cl values were used for calculated masses. Analytical Thin Layer Chromatography (TLC) was performed on Merck silica gel plates (Merck, Kieselgel 60, 0.25 mm thickness) with F254 indicator. Column chromatography was performed on silica gel (Merck, Kieselgel 60, 230-400 mesh; for 3 g crude mixture, 100 g silica gel was used in a column of 3 cm in diameter and 60 cm in length). 1H and 31P NMR spectra were recorded in CDCl3 solutions on a Varian INOVA 500 MHz spectrometer using TMS as an internal reference for 1H NMR and 85 % H3PO4 as an external reference for 31P. The FT-IR spectra were recorded with Bio-Rad 175 FTS. The spectrum resolution was 2 cm-1 and 16 scans were recorded for all samples. The spectra were recorded on the base of KBr pellet technique. The thermal analyses are performed on Mettler Toledo TGA/SDTA 851 and Mettler Toledo DSC 822 devices. The thermo gravimetric analyses are measured between 20 and 1000 oC with 10 oC/min and under the 50 mL/min N2 flow. The DSC data recorded between RT and 350 oC. The heating rate was also 10 oC/min.
3. Synthesis
Reaction of compound (1) with 2,2,3,3-tetrafluorobutane-1,4-diol (2) to form (4), (6).
Compound (1)31 (2 g, 5.5 mmol) and 2,2,3,3-tetrafluoro butanediol (2) (0.9 g, 5.5 mmol) were dissolved in 70 mL of dry THF in a 100 mL three-necked round-bottomed flask. The reaction mixture was cooled in an ice-bath and NaH (60 % oil suspension, 0.44 g, 11 mmol) in 10 mL of dry THF was quickly added to the stirred solution under an argon atmosphere. The reaction was stirred for 23 h at rt and followed by TLC silica gel plates using n-hexane:EtOAc (3:2) and 2 compounds were observed. The reaction mixture was filtered to remove sodium chloride, the solvent removed under reduced pressure and the resulting colourless oil was subjected to column chromatography, using hexane:EtOAc (3:2) as eluent. The first product is the mono-spiro derivative (4) (mp 114-115 oC), Yield 1.37 g, 55 %). Anal. Calcd for C8H14Cl2F4N5O2P3: C 21.26, H 3.12, N 15.49 %. Found: C 21.20, H 3.15, N 15.47 %. MS (ESI): m/z: calcd. for 452.05, Found: 453. 1H NMR (CDCl3 solution): δ 1.71-1.8 (m, 2H, -NHCH2CH2CH2NCH3-), 2.4 (broad, 1H, NH), 3.52 (d, 3H, NCH3), 3.81-3.95(m, 2H, CH3NCH2), 4.13-4.2 (m, 2H, NCH2), 4.3-4.56 (m, 4H, POCH2(CF2)2OCH2). IR (KBr, cm-1), ν=1200 (P=N), ν= 530 (P-Cl), ν= 700 (P-N), ν= 3235 (broad, N-H), ν=1000 (N-C), ν= 1130 (C-C), ν= 2900 (C-H), ν=839 (P-O), ν= 1111 (C-F), ν=1150 (C-C), ν=1087 (C-O) and ν=2951-2817 cm-1(C-H). The second product is the di-spiro derivative (6) (mp 221-222 oC), (Yield 0.25 g, 8 %). Anal. Calcd for C13H22F8N5O4P3 : C 28.02, H 3.98, N 12.57 %. Found: C 28.08, H 3.91, N 12.63 %. MS(ESI): m/z: calcd. for 557, Found: 558. 1H NMR (CDCl3 solution δ 1.71-1.81 (m, 2H, -NHCH2CH2CH2NCH3-), 2.42 (broad, 1H, NH), 3.52 (d, 3H, NCH3), 3.81-3.94(m, 2H, CH3NCH2), 4.13-4.21 (m, 2H, NCH2), 4.31-4.56 (m, 4H, POCH2(CF2)2OCH2). IR (KBr, cm-1), ν=1200 (P=N), ν= 530 (P-Cl), ν= 700 ((P-N), ν= 3321 (broad, N-H), ν=1000 (N-C), ν= 1130 (C-C), ν= 2900 (C-H), ν=839 (P-O), ν= 1111 (C-F), ν=1150 (C-C), ν=1087 (C-O) and ν=2951-2817 cm-1(C-H). Both products were crystallized from n-hexane:dichloromethane (1:3) and obtained as white crystals.
Reaction of compound (1) with 2,2,3,3,4,4-hexafluoropentane-1,5-diol (3) to form (5), (7), (8).
Compound (1)31 (2 g, 5.5 mmol) and 2,2,3,3,4,4-hexafluoropentane-1,5-diol (3) (1.17 g, 5.5 mmol) were dissolved in 70 mL of dry THF in a 100 mL three-necked round-bottomed flask. The reaction mixture was cooled in an ice-bath and NaH (60 % oil suspension, 0.44 g, 11 mmol) in 10 mL of dry THF was quickly added to the stirred solution under an argon atmosphere. The reaction was stirred for 23 h at rt and followed by TLC silica gel plates using hexane: EtOAc (2:1) and 4 compounds were observed. The reaction mixture was filtered to remove sodium chloride, the solvent removed under reduced pressure and the resulting colourless oil was subjected to column chromatography, using n-hexane: EtOAc (2:1) as eluent. The first product is starting material (1) (0.6 g, 30 %). The second product is (8) mono-ansa derivative (mp 164-165 oC) (yield 0.19 g, 7 %). Anal. Calcd for C9H14Cl2F6N5O2P3 : C 21.53, H 2.81, N 13.95 %. Found: C 21.60, H 2.78, N 13.97 %. MS (ESI): m/z: calcd for 501, Found: 501. 1H NMR (CDCl3 solution): δ 1.71-1.78 (m, 2H, -NHCH2CH2CH2NCH3-), 2.4 (broad, 1H, NH), 3.55 (d, 3H, NCH3), 3.85-3.95 (m, 2H, CH3NCH2), 3.88-4.2 (m, 2H, NCH2), 4.3-4.41 (m, 4H, POCH2(CF2)3OCH2). IR (KBr, cm-1), ν=1200 (P=N), ν= 530 (P-Cl), ν= 700 (P-N), ν= 3429 (sharp, N-H), ν=1000 (N-C), ν= 1130 (C-C), ν= 2900 (C-H), ν=839 (P-O); ν= 1111 (C-F), ν=1150 (C-C), ν=1087 (C-O) and ν=2951-2817 (C-H). The band at 3429 cm-1 assigned to the N-H vibration is very sharp and the frequency of the vibration is higher than the other compounds, which indicates that there is a no H-bond formed between the molecules. The third product is the mono-spiro derivative (5) (mp 122-123 oC) (Yield 0.2 g, 7 %). Anal. Calcd for C9H14Cl2F6N5O2P3: C 21.53, H 2.81, N 13.95 %. Found: C 21.57, H 2.76, N, 13.99 %. MS(ESI): m/z: calcd for 501, Found 502.08. 1H NMR (CDCl3 solution): δ 1.7-1.8 (m, 2H, -NHCH2CH2CH2NCH3-), 2.4 (broad, 1H, NH), 3.52 (d, 3H, NCH3), 3.81-3.98(m, 2H, CH3NCH2), 4.13-4.19 (m, 2H, NCH2), 4.4-4.53 (m, 4H, POCH2(CF2)3OCH2). IR (KBr, cm-1), ν=1200 ((P=N), ν= 530 ((P-Cl), ν= 700 ((P-N), ν= 3344 (broad, (N-H), ν=1000 ((N-C), ν= 1130 ((C-C), ν= 2900 ((C-H), ν=839 (P-O); ν= 1111 (C-F), ν=1150 (C-C), ν=1087 (C-O) and ν=2951-2817 cm-1(C-H). The fourth product is di-spiro (7) (mp 210-211 oC) (Yield 1,06 g, 30 %), isolated as white powders and recrystallized from n-hexane-CH2Cl2 (1:2). Anal. Calcd for C14H18F12N5O4P3 : C 26.22, H 2.83, N 10.92 %. Found: C 26.24, H 2.80, N 10.87 %. MS(ESI): m/z: calcd for 641, Found: 642. 1H NMR (CDCl3 solution): δ 1.71-1.79 (m, 2H, -NHCH2CH2CH2NCH3-), 2.41 (broad, 1H, NH), 3.52 (d, 3H, NCH3), 3.81-3.96(m, 2H, CH3NCH2), 4.14-4.20 (m, 2H, NCH2), 4.4-4.55 (m, 4H, POCH2(CF2)3OCH2). IR (KBr, cm-1), ν= 1200 (P=N), ν= 530 (P-Cl), ν= 700 (P-N), ν= 3360 (broad, N-H), ν=1000 (N-C), ν= 1130 (C-C), ν= 2900 (C-H), ν=839 (P-O); ν= 1111 (C-F), ν=1150 (C-C), ν=1087 (C-O) and ν=2951-2817 (C-H).
ACKNOWLEDGEMENTS
We thank the Otsuka Chemical Co. Ltd for gifts of N3P3Cl6 and Gebze Institute of Technology (GIT) for the provided financial support (grant no: BAP 2008-A-02).
References
1. M. G. Muralidhara, N. Grover, and V. Chandrasekhar, Polyhedron, 1993, 12, 1509. CrossRef
2. S. J. Coles, D. B. Davies, R. J. Eaton, M. B. Hursthouse, A. Kılıc¸ R. A. Shaw, and A. Uslu, Dalton Trans., 2006, 1302. CrossRef
3. N. Satish Kumar and K.C. Kumara Swamy, Polyhedron, 2004, 23, 979. CrossRef
4. H. A. Al-Madfa, R. A. Shaw, and S. Ture, Phosphorus, Sulfur, and Silicon, 1990, 53, 333. CrossRef
5. H. A. Al-Madfa, A. H. Alkubaisi, H. G. Parkers, and R. A. Shaw, Heterocycles, 1989, 28, 347. CrossRef
6. a) S. Beşli, S. J. Coles, D. B. Davies, R. J. Eaton, A. Kılıç, and R. A. Shaw, Polyhedron, 2006, 25, 963; CrossRef b) S. Beşli, S. J. Coles, D. Davarcı, D. B. Davies, M. B. Hurthouse, and A. Kılıç, Polyhedron, 2007, 26, 5283. CrossRef
7. K. Brandt, T. Kupka, J. Drozd, J. C. van de Grampel, A. Meetsma, and A. P. Jekel, Inorg. Chim. Acta, 1995, 228, 187. CrossRef
8. S. J. Coles, D. B. Davies, R. J. Eaton, M. B. Hursthouse, A. Kılıç, R. A. Shaw, and Y. Çiftçi, Polyhedron, 2006, 25, 953. CrossRef
9. R. A. Shaw, Phosphorus Sulfur, and Silicon, 1989, 45, 103. CrossRef
10. G. Guerch, J. F. Labarre, R. Lahana, R. Rocques, F. Sournies, and J. Galy, J. Mol. Struct., 1983, 99, 275. CrossRef
11. G. Guerch, J. F. Labarre, R. Lahana, F. Sournies, J. Galy, R. Enjalbert, J. Galy, and J. P. Declercq, Inorg. Chim. Acta, 1984, 83, L33. CrossRef
12. H. R Allcock, M. E. Napierala, C. G. Cameron, and S. J. M. O’Connor, Macromolecules, 1996, 29, 1951. CrossRef
13. J. F. Labarre, Top. Curr. Sci., 1982, 102, 1.
14. J. F. Labarre, Top. Curr. Sci., 1985, 129, 173.
15. K. Brandt and Z. Jedlinski, Makromol. Chem. Suppl., 1985, 9, 169. CrossRef
16. R. E. Singler, A. J. Deome, D. A. Dunn, and M. J. Bieberich, Ind. Eng. Chem., Prod. Res. Dev., 1986, 25, 46. CrossRef
17. M. A. Keller and C. S. Saba, Anal. Chem., 1996, 68, 3489. CrossRef
18. K. Inoue, T. Yamauchi, T. Itoh, and E. Ihara, J. Inorg. and Organometal. Polymers and Materials, 2007, 17, 367. CrossRef
19. W. Vanek, Angew Chem., Int. Ed. Engl., 1969, 8, 617. CrossRef
20. S. S. Krishnamurty and A. C. Sau, Inorg. Chem. Radiochem., 1978, 21, 41. CrossRef
21. C. W. Allen, In The Chemistry of Inorganic Homo- and Hetero-Cycles; ed. by. I. Haiduc and B. D. Sowerby, Academic Press: London, 1987, 2, 501.
22. C. W. Allen, J. Fire Sci., 1993, 11, 320. CrossRef
23. J. L. Lin and J. T. Yates, J. Vac. Sci. Technol. A., 1995, 13, 1867. CrossRef
24. C. L. Jiaa, Y. Liu, and C. Gao, Trib. Trans., 2000, 43, 659. CrossRef
25. V. Raman, D. Gillis, and R. Volter, J. Tribol, 2000, 122, 444. CrossRef
26. Q. Zhao, H. J. Kang, and F. E. Talke, Lubrication Eng., 2001, 57, 15.
27. R. J. Waltman, B. Lensfield, and J. Pacansky, Chem. Mat., 1997, 9, 2185. CrossRef
28. H. S.Wu and S. S. Meng, Ind. Eng. Chem. Res., 1998, 37, 675. CrossRef
29. B. Yu. Sultanly, A. N. Shnulin, R. E. Aliev, and M. N. Maharramov, J. Appl. Spectroscopy, 2003, 70, 5. CrossRef
30. H. İbişoğlu, F. Hacıvelioğlu, and A. Kılıç, Heterocycles, 2008, 75, 839. CrossRef
31. E. S. Kumar, M. G. Muralidhara, and V. Chandrasekhar, Polyhedron, 1995, 14, 1571 CrossRef