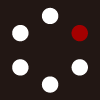
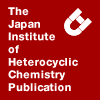
HETEROCYCLES
An International Journal for Reviews and Communications in Heterocyclic ChemistryWeb Edition ISSN: 1881-0942
Published online by The Japan Institute of Heterocyclic Chemistry
e-Journal
Full Text HTML
Received, 10th July, 2009, Accepted, 4th September, 2009, Published online, 4th September, 2009.
DOI: 10.3987/COM-09-S(S)50
■ Stereoselective Preparation of Oxygenated Heterocycles Using Stereocontrolled Tandem Double-Allylation of Carbonyl Compounds with a Boron-Silicon Reagent
Umakanthan Sivasubramaniam and Dennis G. Hall*
Department of Chemistry, University of Alberta, Edmonton, Alberta T6G 2G2, Canada
Abstract
A one-pot three-component reaction between carbonyl double-allylation reagent 1 and aldehydes was optimized to provide a high diastereoselectivity in the formation of all-cis trisubstituted tetrahydrofurans. A similar procedure applied to dicarbonyl substrates provided an oxabicyclic compound embedding an 8-membered medium ring.Saturated oxygen-containing heterocycles such as tetrahydrofurans and pyrans are key components of numerous natural products and pharmaceutical drugs.1 There is a need to develop rapid means for synthesizing these important classes of heterocycles from simple components.1 In this regard, we have recently developed the carbonyl double allylation reagent 1, which is based on boron and silicon (Figure 1).2,3 This optically pure reagent adds onto aldehydes first via an allylboration (transition state 2), to give a secondary alcohol product, 3, with concomitant unmasking of an allylic silane (Eq. 1). The allylic silane unit of 3 can then react chemoselectively with a second aldehyde to provide all-cis trisubstituted tetrahydrofurans 5 through cyclization onto oxonium intermediate 4.4
In our initial studies, we found that the diastereoselectivity of this process was higher when performed in two distinct steps with isolation of allylic silanes 3. Although a one-pot sequential addition of two aldehydes afforded the desired products in excellent enantioselectivity, the diastereoselectivity was notably lower compared to the two-step process.2 When using the one-pot procedure with a final temperature of 0 °C, a significant proportion of 1,2-trans diastereomer was observed. In a related application, we showed one example where a ketoaldehyde, 6, can be utilized to promote first an intermolecular allylboration, then an intramolecular allylsilation5 that provides oxabicyclic product 7 embedding a 7-membered carbocycle. As such, it became apparent that this approach could be useful in providing medium rings following an opening of the oxygen bridge. Thus, in this study our objective was twofold: 1. Develop a highly diastereoselective one-pot three-component reaction procedure to prepare trisubstituted tetrahydrofurans 5 from reagent 1 and two different aldehydes, and 2. Expand this procedure to the use of dicarbonyl substrates as a means to access medium ring systems.
The optimization of a one-pot three-component procedure for tetrahydrofurans 5 focused on identifying the optimal reaction temperature for the second aldehyde allylation. Thus, using hydrocinnamaldehyde as the first aldehyde substrate with reagent 1 and boron trifluoride etherate at –78 °C,6 benzaldehyde was added as the second aldehyde at this temperature. The final temperature at which the solution was warmed up to was varied and the yield and diastereoselectivity in the formation of 1,2,4-trisubstituted tetrahydrofuran 5a was assessed (Table 1). Although a temperature of 0 °C led to a shorter reaction time, the resulting diastereoselectivity ratio of 7:1 was disappointing (entry 1). On the other hand, maintaining the temperature of the second allylation at lower temperatures led to a higher stereoselectivity (entry 2). When reacted at –40 °C for 20 hours, the model reaction afforded a single diastereomer, however in a low yield of 40% (entry 3). Increasing the reaction time to 48 hours led to an appreciable increase of the yield of 5a with excellent diastereoselectivity (entry 4). None of the 1,2-trans diastereomer was observed in the crude product, and the desired all-cis product 5a was obtained in 91–94% ee. Using these optimal conditions, a few more examples of products were compiled to ascertain the reproducibility and reliability of this procedure. Thus, all-cis trisubstituted tetrahydrofurans 5a-5c were all obtained in very high diastereoselectivity (Figure 2). In the case of 5c, 3-tert-butyldimethylsiloxy propanal was employed as the starting aldehyde but the TBS protecting group was removed either during the work-up and the purification on silica column.
The application of this one-pot double allylation procedure was evaluated with a series of dicarbonyl substrates to explore the limits of this method as a means to access medium ring, oxygen-bridged carbocycles. In our previous study, a 7-membered carbocycle was made successfully (Figure 1, Eq. 2).2 Ketoaldehydes were chosen so as to minimize intermolecular allylation for the allylsilation, which, as the second allylation, will occur on the phenylketone. The first allylation with the allylic boronate unit is expected to occur on the aldehyde, and the second allylation on the hindered phenylketone should be slow despite its intramolecular nature. Using reagent 1 and the shortest dicarbonyl substrate, keto-aldehyde 8a, we first examined conditions similar to the above tetrahydrofurans, at normal concentration (Table 2). Unfortunately, at 0.1 M concentration, none of the desired product 9a was obtained (entry 1). The difficult intramolecular ketone allylation is evident by the isolation of 60% of product 11a, which originates from a second, intermolecular allylation on the aldehyde unit of 8a. It was expected that this undesired product could be suppressed by working at a lower concentration that would disfavor the intermolecular process. In the event, diluting the reaction concentration down to 0.004 M led to a 60% yield of the desired product 9a with only 10% of side-product 11a (entry 3). Remarkably, product 9a embeds an 8-membered carbocycle. These conditions, however, failed to deliver the 9- and 10-membered carbocycles with the longer ketoaldehydes 8b and 8c (entries 4-5).
In summary, by employing carbonyl double-allylation reagent 1, a one-pot three-component synthesis of all-cis trisubstituted tetrahydrofurans was optimized to provide a high enantio- and diastereoselectivity. Using a dicarbonyl substrate in a similar procedure under dilute conditions, an oxabicyclic compound embedding an 8-membered medium ring was isolated in good yield. Carbocycles of this sort are difficult to obtain using other methods. Overall, these results further expand the versatility of boron-silicon double-allylation reagent 1. Applications to the synthesis of biologically active natural products are underway.
EXPERIMENTAL
All reactions were carried out under an argon atmosphere using flame-dried glassware. CH2Cl2, methanol, and toluene were distilled over CaH2. THF was distilled over sodium/benzophenone. All aldehydes were purified by Kugelrohr distillation prior to use.
One-pot three-component syntheses of all-cis trisubstituted tetrahydrofurans 5a-5c
General procedure: In a reaction vessel, the aldehyde R1CHO (0.50 mmol) and reagent 1 (0.50 mmol) in 3 mL of CH2Cl2 were mixed and cooled to _78 oC. Next, BF3·Et2O (0.50 mmol) was added and the mixture was stirred at _78 oC for 13_16 h. Then, benzaldehyde (0.50 mmol) was added and stirred at _78 oC for 2 h at _78 oC. Thereafter, the reaction mixture was warmed to _40 oC (same bath) and stirred for 24_72 h at _40 oC. The reaction mixture was quenched with sat. aqueous NaHCO3 (20 mL) and extracted with Et2O (4 x 5 mL). The combined organic layers were dried over anhydrous Na2SO4, filtered, and the solvent was removed by rotary evaporator. The all-cis stereochemistry of the products was proved using nOe experiments.2
2-Phenyl-5-(2’-phenylethyl)-3-vinyltetrahydrofuran 5a
Preparation of this compound was described previously.2 Analytical data of product 5a was identical to that reported in this reference.
5-Heptyl-2-phenyl-3-vinyltetrahydrofuran 5b
The reaction scale was 0.45 mmol. Flash chromatography (0_5% EtOAc in hexanes) provided a clear liquid (66 mg, 50% yield). TLC (25% EtOAc in hexanes, phosphomolybdic acid/Ce(IV)sulfate/aq. H2SO4): Rf 0.69; 1H NMR (CDCl3, 300 MHz): δ 7.35-7.20 (m, 5H), 5.18 (ddd, J = 9.0, 10.2, 17.1 Hz, 1H), 5.05 (d, J = 8.2 Hz, 1H), 4.90 (ddd, J = 0.8, 2.0, 17.0 Hz, 1H), 4.76 (dd, J = 2.1, 10.2 Hz, 1H), 4.02 (qd, J = 6.0, 9.3 Hz, 1H), 3.18 (p, J = 8.4 Hz, 1H), 2.20 (ddd, J = 5.4, 7.5, 12.3 Hz, 1H), 1.85 (m, 1H), 1.68 (m, 1H), 1.40-1.25 (m, 11H), 0.90 (m, 3H); 13C NMR (CDCl3, 100 MHz): δ 140.6, 138.7, 127.7, 126.9, 126.8, 114.7, 83.1, 79.6, 48.9, 38.3, 35.6, 31.8, 30.0, 29.3, 26.5, 22.7, 14.1; IR (CDCl3, cast film): cm–1 3067, 3030, 2956, 2928, 2857, 1730, 1641, 1494, 1454, 1359, 1110, 1095, 995, 912, 721, 698; HRMS (ES, m/z) calculated for C19H28ONa: 295.2032, found: 295.2032 [(M+Na)+].
2-(5-Phenyl-4-vinyltetrahydrofuran-2-yl)ethanol 5c
The alcohol was protected with a TBS in the starting aldehyde, but it was removed during the workup or the purification on silica column. The reaction scale was 0.43 mmol. Flash chromatography (10_80% EtOAc in hexanes) provided a yellow liquid (58 mg, 56 % yield). TLC (50% EtOAc in hexanes, KMnO4 stain): Rf 0.50; 1H NMR (CDCl3, 300 MHz): δ 7.32 (m, 2H), δ 7.21 (m, 3H), δ 5.18 (ddd, J = 8.7, 9.9, 17.1 Hz, 1H), δ 5.09 (d, J = 8.4 Hz, 1H), 4.92 (ddd, J = 0.9, 2.0, 17.1 Hz, 1H), 4.78 (ddd, J = 0.8, 2.0, 9.9 Hz, 1H), 4.22 (m, 1H), 3.89 (q, J = 5.4 Hz, 2H), 3.18 (p, J = 8.7 Hz, 1H), 2.60 (t, J = 5.4, 1H), 2.23 (ddd, J = 5.4, 7.2, 12.3 Hz, 1H), 2.02 (m, 2H), 1.65 (td, 9.3, 12.3 Hz, 1H); 13C NMR (CDCl3, 100 MHz): δ 139.9, 138.1, 127.9, 127.1, 126.7, 115.3, 83.7, 79.3, 62.0, 48.4, 38.6, 37.5; IR (CHCl3, cast film): cm–1 3388, 3076, 3029, 2929, 2878, 1641, 1604, 1493, 1453, 1068, 914, 752, 722, 699; HRMS (ES, m/z) calculated for C14H18O2Na: 241.1199, found: 241.1195 [(M+Na)+].
Synthesis of oxabicyclic compound 9a: 9-Oxa-1-Phenyl-8-vinylbicyclo[4.2.1]nonane
In a 250 mL round bottom flask, ketoaldehyde 8a (126 mg, 0.66 mmol, 1.05 equiv) and reagent 1 (193 mg, 0.63 mmol, 1.00 equiv) in 150 mL of CH2Cl2 were mixed and cooled to _78 oC and stirred for 30 minutes. Next, BF3·Et2O (0.13 mL, 1.00 mmol, 1.50 equiv) was added to the flask and stirred for 12 h at _78 oC, followed by 9 h at 0 oC (different bath). The reaction mixture was quenched with sat. aqueous NaHCO3 (20 mL) and extracted with Et2O (4 x 25 mL). The combined organic layers were dried over anhydrous Na2SO4, filtered and the solvent was removed by rotary evaporator. Flash chromatography (0_5% EtOAc in hexanes) provided a clear oil (85.0 mg, 60.0% yield). TLC (25% EtOAc in hexanes, magic stain): Rf 0.69; 1H NMR (CDCl3, 300 MHz): δ 7.48 (dd, J = 1.4, 8.5 Hz, 2H), 7.31 (m, 3H), 5.99 (ddd, J = 7.3, 10.2, 17.5 Hz, 1H), 5.15 (ddd, J = 1.0, 1.9, 10.3 Hz, 1H), 5.00 (ddd, 1.2, 1.9, 17.0 Hz, 1H), 4.70 (m, 1H), 3.02 (dt, J = 5.1, 8.2 Hz, 1H), 2.71 (q, J = 9.9 Hz, 1H), 2.44 (td, J = 9.4, 12.9 Hz, 1H), 2.10 (m, 3H), 1.72 (m, 5H); 13C NMR (CDCl3, 100 MHz): δ 148.4, 136.7, 128.1, 126.1, 124.9, 117.7, 87.0, 76.6, 56.1, 44.5, 38.4, 34.7, 25.1, 24.9; IR (CDCl3, cast film): cm–1 3077, 3026, 2927, 2859, 1638, 1602, 1492, 1470, 1446, 1350, 1095, 1072, 1013, 914, 753, 700; HRMS (EI, m/z) calculated for C16H20O: 228.1514, found: 228.1515 [M+]. HPLC: Chiralcel OD (0.46 cm i.d. X 25 cm), 5% i-PrOH/hexanes, 0.50 mL/min., UV detection at 210 nm, major peak at 7.1 min., minor peak at 8.5 min., 93% ee. A small quantity of 11a, 5-[5-(5-Oxo-5-phenyl-pentyl)-3-vinyl-tetrahydro-furan-2-yl]-1-phenyl-pentan-1one, was also isolated. Flash chromatography (0_5% EtOAc in hexanes) yielded a white gel (28 mg, 10 % yield). TLC (25% EtOAc in hexanes, magic stain): Rf 0.39; 1H NMR (CDCl3, 400 MHz): δ 7.95 (m, 4H), 7.55 (m, 2H), 7.46 (m, 4H), 5.74 (ddd, J = 9.0, 9.3, 18.0 Hz, 1H), 5.00 (d, J = 5.2 Hz, 1H), 4.97 (s, 1H), 3.79 (m, 2H), 2.97 (q, J = 8.4 Hz, 4H), 2.81 (m, 1H), 2.19 (ddd, J = 6.8, 8.0, 15.8 Hz, 1H), 1.76 (m, 5H), 1.46 (m, 8H); 13C NMR (CDCl3, 100 MHz): δ 200.3, 138.7, 137.1, 132.8, 128.5, 128.0, 115.0, 81.3, 78.4, 46.7, 38.5, 38.3, 36.2, 31.6, 26.2, 24.5; IR (CDCl3, cast film): cm–1 3066, 2936, 2862, 1686, 1598, 1449, 1224, 1002, 752, 691; HRMS (ES, m/z) calculated for C28H34O3Na: 441.2400, found: 441.2402 [(M+Na)+]. [α]25D 16.61 (c = 0.92, CHCl3).
ACKNOWLEDGMENTS
The authors are grateful to the Natural Sciences and Engineering Research Council (NSERC) of Canada for financial support of this research.
References
1. a) M. C. Elliott, J. Chem. Soc., Perkin Trans. 1, 2002, 2301; CrossRef b) J. P. Wolfe and M. B. Hay, Tetrahedron, 2007, 63, 261. CrossRef
2. F. Peng and D. G. Hall, J. Am. Chem. Soc., 2007, 129, 3070. CrossRef
3. For other examples of carbonyl double allylation reagents, see: a) W. R. Roush and P. T. Grover, Tetrahedron Lett., 1990, 31, 7567; CrossRef b) A. G. M. Barrett and J. W. Malecha, J. Org. Chem., 1991, 56, 5243; CrossRef c) W. R. Roush and P. T. Grover, Tetrahedron, 1992, 48, 1981; CrossRef d) J. A. Hunt and W. R. Roush, J. Org. Chem., 1997, 62, 1112; CrossRef e) W. R. Roush, A. N. Pinchuk, and G. C. Micalizio, Tetrahedron Lett., 2000, 41, 9413; CrossRef f) A. G. M. Barrett, D. C. Braddock, P. D. de Koning, A. J. P. White, and D. J. Williams, J. Org. Chem., 2000, 65, 375; CrossRef g) E. M. Flamme and W. R. Roush, J. Am. Chem. Soc., 2002, 124, 13644; CrossRef h) J. H. Smitrovich and K. A. Woerpel, Synthesis, 2002, 2778; CrossRef i) T. K. Sarkar, S. A. Haque, and A. Basak, Angew. Chem. Int. Ed., 2004, 43, 1417. CrossRef
4. a) P. Mohr, Tetrahedron Lett., 1993, 34, 6251; CrossRef b) J. H. Cassidy, S. P. Marsden, and G. Stemp, Synlett, 1997, 1411; CrossRef c) C. Meyer and J. Cossy, Tetrahedron Lett., 1997, 38, 7861. CrossRef
5. a) I. E. Markó and A. Mekhalfia, Tetrahedron Lett., 1992, 33, 1799; CrossRef b) M. Suginome, T. Iwanami, Y. Ito, J. Org. Chem., 1998, 63, 6096; CrossRef c) M. Suginome, T. Iwanami, and Y. Ito, Chem. Commun., 1999, 2537. CrossRef
6. a) L. Carosi and D. G. Hall, Angew. Chem. Int. Ed., 2007, 46, 5913; CrossRef b) D. G. Hall, Synlett, 2007, 1644. CrossRef