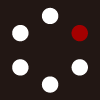
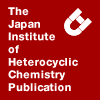
HETEROCYCLES
An International Journal for Reviews and Communications in Heterocyclic ChemistryWeb Edition ISSN: 1881-0942
Published online by The Japan Institute of Heterocyclic Chemistry
e-Journal
Full Text HTML
Received, 22nd June, 2009, Accepted, 31st July, 2009, Published online, 3rd August, 2009.
DOI: 10.3987/COM-09-11780
■ Formation Pathway of Novel Cycloadduct Obtained by Reaction of 3,5-Disubstituted 4-Oxo-4H-pyrazole 1,2-Dioxide with Dimethyl Acetylenedicarboxylate
Yasuyuki Yoshitake, Koki Yamaguchi, and Kazunobu Harano*
Laboratory of Molecular Design, Sojo University, 4-22-1, Ikeda, Kumamoto-shi, 860-0082, Japan
Abstract
A new formation pathway via [3,3]-sigmatropic rearrangement of the 1,3-dipolar reaction product was proposed for the novel nitrogen-free compound formed from the reaction of 3,5-disubstituted 4-oxo-4H-pyrazole 1,2-dioxide with dimethyl acetylenedicarboxylate.INTRODUCTION
Freeman et al. reported that the reaction of 2-methyl-5-phenyl-4-oxo-4H-pyrazole 1,2-dioxide (1a) with dimethyl acethylenedicarboxylate (2) gave a nitrogen-free compound, 4-oxo-8-oxabicyclo[3.2.1]octa-2,6-diene derivative (6a) via intermediates 4a and 5a in place of the normal 1,3-dipolar reaction product (3a) and 6a was also obtained from the reaction of the mono N-oxide (1am) with 2.1
However, it is doubtful that the proposed reaction mechanism involves a thermally forbidden 1,3-sigmatropic rearrangement (3a -> 4) of the N-O bond. The semiempirical molecular orbital (MO) calculation could not reproduce the proposed reaction mechanism i.e., formation of the 1,3-rearrangement reaction product.2
In this paper, a possible thermally allowed pathway is discussed on the basis of the frontier molecular orbital (FMO) analysis and MO calculations.
RESULTS AND DISCUSSION
In order to confirm the structure of the nitrogen-free compound (6a), we have made a single crystal, and performed X-ray crystallographic analysis. As shown in Figure 1, the formation of 1,8-oxabicyclo[3.2.1]octa-3,6-dien-2-one skeleton could be confirmed, suggesting that the 1,3-dipolar reactions occurred at the methyl nitrone moiety3b if the 1,3-shift mechanism would be operating.
In connection with the neutral-type reaction4 behavior of 3,5-bis(methoxycarbonyl)-4-oxo-4H-pyrazole 1,2-dioxide (1b) towards electron-deficient dipolarophiles,3,5 the reaction of 1b with dimethyl acethylenedicarboxylate (2) was studied with intention of obtaining a corresponding 1:2 cycloadduct (8).3c
Contrary to our expectation, the products obtained from the reaction of 1b with 2 were a nitrogen free compound, 4-oxo-8-oxa-bicyclo[3.2.1]octa-2,6-diene derivative (6b) and an isoxazole derivative (7) (see Figure 2). The structure of 6b was established on the basis of the NMR spectral data for 6a.
On the basis of the molecular orbital theory,7 we tried to explain the reaction mechanism using thermally allowed pericyclic reactions.
Inspection of the primary 1,3-dipolar reaction product (3a,b) indicates that the cycloadduct has a 1,5-diene system suitable for thermally allowed [3,3]-sigmatropic rearrangement (Scheme 3).
If the [3,3]-sigmatropic shift occurred, 5-oxo-2,3-diazabicyclo[2.1.1]hex-2-ene-2-oxide skeleton 9 may be produced. The rearrangement product 9 is assumed to be an 1,3-dipolar cycloadduct of nitrous oxide and a cyclopropanone derivative which acts as a 1,3-dipole8,9 (Figure 3).
Therefore, the extrusion reaction of N2O is retro 1,3-dipolar reaction of the [3,3]-sigmatropic rearrangement product 9. Thus formed cyclobutene 10 readily undergoes electrocyclic reaction to give a butadiene moiety. The resulting penta-2,4-dienal 11 may cyclize to a pyran derivative 5b, which acts as 4π diene to give the final product 6. The FMO interaction for the final cycloaddition is depicted in Scheme 3.
On these assumptions, we tried to locate the transition state (TS) of the trigger reaction, that is, [3,3]-sigmatropic rearrangement of the 1,3-dipolar cycloadduct. The results are summarized in Table 1.
As shown in Figure 4a, the TS for the parent compound A could be successfully located in all MO methods used.10,11 The reaction barrier at B3LYP using 6-311++G(d,p) basis sets are 32.72 (ZPE corrected 30.51) kcal/mol. The interacting bond distances for N----O and C----C are 2.88 and 2.49Å, respectively, in which bond breaking precedes bond making. This is strong contrast to the HF-3-21G TS geometry (see Figure 4a).
As for 3b, the DFT calculations with full structure optimization were performed using the PM3-optimized structure. The reaction barriers at B3LYP using 6-31G(d) and 6-31+G(d) basis sets are 41.74 (ZPE corrected 39.41) and 38.15 (ZPE corrected 35.94) kcal/mol, respectively, those are comparable to the values using the B3LYP functional for the Cope rearrangement of 1,5-hexadiene.12 Each transition structure was calculated to be a looser one in comparison with a less strained TS for simple model compound B (Figure 5), showing a significantly twisted boat form.
The relative reactivity of [3,3]-shift between 3a and 3b can be explained in terms of the FMO three system interaction,13 in which the substituent effect on HOMO energy levels plays an important role.
Taking into account of not only the substituent and solvent effects but also the calculation result that the reaction barrier of the 1,3-shift is considerably high, the newly proposed reaction pathway via [3,3]-sigmatropic rearrangement seems to be plausible. This mechanism is compatibly applicable to the
formation pathway of 6a from the mono N-oxide (1am).
In conclusion, we can safely say that the nitrogen-free reaction product 6 is formed from a multi-step cascade reaction in which all reactions are thermally allowed.
EXPERIMENTAL
Melting points were uncorrected. The IR spectra were taken with a Hitachi 270-30 spectrophotometer. 1H-NMR and 13C-NMR spectra were taken with JEOL JNM-EX 270 (270 MHz) and JNM-A 500 (500 MHz) spectrometers for ca.10% solution with TMS as an internal standard; chemical shifts are expressed as δ values and the coupling constants (J) are expressed in Hz. UV spectra were recorded on a Shimadzu UV-2500PC spectrophotometer.
Materials
2-methyl-5-phenyl-4-oxo-4H-pyrazole 1,2-dioxide (1a) and 3,5-bis(methoxycarbonyl)-4-oxo-4H-pyrazole 1,2-dioxide (1b) were prepared by the previously reported methods.1c
Reaction of 1a with 2
A solution of 1.2 g (5 mmol) of la in 2 mL of benzene and 2 mL of 2 was heated under reflux for 6 h. The mixture was cooled and the benzene and excess ester were removed under vacuum. The residue was chromatographed on silica gel. The products separated were crystallized from appropriate solvents.
6b: Yield 30%. Yellow oil. IR (NaCl): 1738 (C=O). 1H NMR (500 MHz, CDCl3): δ (ppm) 3.61 (3H, s, C12-Me), 3.67 (3H, s, C24-Me), 3.74 (3H, s, C32-Me), 3.88 (3H, s, C28-Me), 3.91 (3H, s, C16-Me), 3.92 (3H, s, C20-Me). 13C-NMR (CDCl3, 125 MHz): δ (ppm) 52.0 (C20), 52.3 (C16, C28 or C32), 52.6 (C16, C28, C32), 52.8 (C16, C28 or C32), 53.2 (C12, C24), 112.7 (C5), 113.5 (C1), 116.5 (C7), 143.9 (C6), 145.1 (C3), 156.0 (C2), 156.6 (C13, C17 or C29), 156.7 (C13, C17 or C29), 159.3 (C13, C17 or C29), 163.5 (C21), 164.3 (C25), 168.6 (C9). FAB-MS (m/z): 469 (M+-1).
7: Yield 56%. Pale yellow powder. mp 110 °C. IR (KBr) cm-1: 1754 (C=O). 1H-NMR (CDCl3, 500 MHz): δ (ppm) 3.98 (3H, s, C13-Me), 4.01 (6H, s, C9-Me, C17-Me). 13C-NMR (CDCl3, 125 MHz): δ (ppm) 154.2 (C3), 117.8 (C4), 155.5 (C5), 160.0 (C6), 53.6 (C9, C17), 158.4 (C10), 53.5 (C13), 159.1 (C14). FAB-MS (m/z): 244 (M++1). Anal. Calcd. for C9H9NO7: C, 44.45; H, 3.73; N, 5.76. Found: C, 44.66; H, 3.71; N, 5.80.
Compound 6a was prepared according to the method described in a previous paper.1b
Molecular Orbital (MO) Calculations
PM6 calculations10d were run through Winmostar interface using MOPAC2009 on a DEL Dimension9200 computer. The ab initio and density functional theory (DFT) computations11 were carried out on a HIT Linux cluster server (4CPU) made up of dual 1.6 GHz Itanium 2 processors. The PM6-optimized structures were used as initial structures for the ab initio and DFT calculations. All the transition structures were confirmed with IRC calculation and harmonic vibrational frequency analysis. The calculation data are available for downloading14 or upon request through E-mail.
Single Crystal X-Ray Analysis of 7 and 6a
A colorless prism crystal of C9H9NO7 having approximate dimensions of 0.20 x 0.10 x 0.30 mm was mounted on a glass fiber. The reflection data were measured on RIGAKU AFC7R four-circle autodiffractometer with a graphite monochromated Mo-Kα radiation (50 kV-150 mA) and rotating anode generator. The data were collected at a temperature of 20 + 1 ℃ to a maximum 2θ value of 55.0°. The structures were solved by direct method (SIR-92),15a and hydrogen atoms were placed at the calculation. A full-matrix least-squares technique with anisotropic thermal parameters for non-hydrogen atoms and riding model for hydrogen atoms. All calculations were performed using the CrystalStructure15 crystallographic software package.
7; C9H9NO7, F.W. =243.17, monoclinic, space group P21/n (#14), The systematic absences of: h0l: h+l ± 2n, 0k0: k ± 2n, uniquely determine the space group to be: P21/n (#14). a=8.132(4), b=10.782(2), c=12.828(3) Å, β=98.96(3)°, V=1110.9(6) Å3, Dc=1.454 gcm-3, Z=4, R=0.060 for 2267 observed reflections (I > 3.00σ(I)), Rw=0.120, CCDC reference number 733853.
Similarly, the crystal structure of 6a was determined. Crystal Data of 6a; C22H20O10, M=444.39, orthorhombic, space group P212121 (#19), a=10.40 (4), b=25.0 (1), c=8.29 (4) Å, V=2154 (16) Å3, Dc=1.370 gcm-3, Z=4, R=0.035 for 2702 observed reflections (I > 3.00σ(I)), Rw=0.048, CCDC reference number 695901.
ACKNOWLEDGEMENTS
We thank Miss K. Takimoto and Miss M. Otani (students of Faculty of Pharmaceutical Sciences, Kumamoto University) for experimental assistance.
References
1. a) J. P. Freeman, J. J. Gannon, and D. L. Surbey, J. Org. Chem., 1969, 34, 187; CrossRef b) J. P. Freeman and M. L. Hoare, J. Org. Chem., 1971, 36, 19; CrossRef c) A. Kotali and V. P. Papageorgiou Org. Prep. Proced. Int., 1991, 23, 611, and references cited therein; CrossRef d) S. Spyroudis and A. Varvoglis, Chimika Chronika, New Series, 1982, 11, 173; e) A. Kotali and P. G. Tsoungas, Heterocycles, 1989, 29, 1615. CrossRef
2. The UHF-PM6 calculation on the reaction paths using the parent compound (A) indicated that the reaction barrier of the [3,3]-shift is lower than that of the [1,3]-shift by 16 kcal/mol. The single point B3LYP/6-31G(d)/PM6 calculations on the PM3-optimized TS structures showed that the corresponding energy difference increases to 44.07 kcal/mol.
3. a) Y. Yoshitake, 2003, “Pericyclic Reaction of Pyrazolone-N,N-dioxides”, Doctoral Dissertation, Kumamoto University; b) N-Phenyl maleimide reacted at the methyl nitrone moiety of 1a; c) The reaction of 1b with N-phenyl maleimide gave the 1:2 cycloadduct.3a.
4. R. Sustmann, Tetrahedron Lett., 1971, 2717. CrossRef
5. a) M. Eto, K. Harano, Y. Yoshitake, and T. Hisano, J. Heterocycl. Chem., 1993, 30, 1557; CrossRef b) M. Eto, Y. Yoshitake, K. Harano, and T. Hisano, J. Chem. Soc. Perkin Trans. 2, 1994, 1337; CrossRef c) Y. Yoshitake, M. Eto, and K. Harano, Heterocycles, 1997, 45, 1873; CrossRef d) Y. Yoshitake, M. Eto, and K. Harano, Tetrahedron Lett., 1998, 39, 2761; CrossRef e) Y. Yoshitake, M. Eto, and K. Harano, Chem. Pharm. Bull., 1999, 47, 601.
6. C. K. Johnson, ORTEP ORNL-3794, Oak Ridge National Laboratory, Tennessee, U. S. A., 1965.
7. I. Fleming, Frontier Orbitals and Organic Chemical Reactions, Wiley, London, 1976.
8. N. J. Turro, S. S. Edelson, J. R. Williams, T. R. Darling, and W. B. Hammond, J. Am. Chem. Soc., 1969, 91, 2283. CrossRef
9. S. S. Edelson and N. J. Turro, J. Am. Chem. Soc., 1970, 92, 2770. CrossRef
10. a) J. J. P. Stewart, J. Am. Chem. Soc., 1985, 107, 3902; CrossRef b) J. J. P. Stewart, J. Comp. Chem., 1989, 10, 209; CrossRef J. Comp. Chem., 1989, 10, 221; CrossRef c) AM1 and PM3 calculation are performed using MOPAC; J. J. P. Stewart, QCPE Bull., 1989, 9, 10; d) J. J. P. Stewart, Optimization of Parameters for Semiempirical Methods V: Modification of NDDO Approximations and Application to 70 Elements, J. Mol. Modeling, 2007, 13, 1173. CrossRef
11. M. J. Frisch, G. W. Trucks, H. B. Schlegel, G. E. Scuseria, M. A. Robb, J. R. Cheeseman, J. A. Montgomery Jr., T. Vreven, K. N. Kudin, J. C. Burant, J. M. Millam, S. S. Iyengar, J. Tomasi, V. Barone, B. Mennucci, M. Cossi, G. Scalmani, N. Rega, G. A. Petersson, H. Nakatsuji, M. Hada, M. Ehara, K. Toyota, R. Fukuda, J. Hasegawa, M. Ishida, T. Nakajima, Y. Honda, O. Kitao, H. Nakai, M. Klene, X. Li, J. E. Knox, H. P. Hratchian, J. B. Cross, V. Bakken, C. Adamo, J. Jaramillo, R. Gomperts, R. E. Stratmann, O. Yazyev, A. J. Austin, R. Cammi, C. Pomelli, J. W. Ochterski, P. Y. Ayala, K. Morokuma, G. A. Voth, P. Salvador, J. J. Dannenberg, V. G. Zakrzewski, S. Dapprich, A. D. Daniels, M. C. Strain, O. Farkas, D. K. Malick, A. D. Rabuck, K. Raghavachari, J. B. Foresman, J. V. Ortiz, Q. Cui, A. G. Baboul, S. Clifford, J. Cioslowski, B. B. Stefanov, G. Liu, A. Liashenko, P. Piskorz, I. Komaromi, R. L. Martin, D. J. Fox, T. Keith, M. A. Al-Laham, C. Y. Peng, A. Nanayakkara, M. Challacombe, P. M. W. Gill, B. Johnson, W. Chen, M. W. Wong, C. Gonzalez and J. A. Pople, Gaussian Inc., Wallingford CT, 2004.
12. O. Weist, K. A. Black, and K. N. Houk, J. Am. Chem Soc., 1994, 116, 10336. CrossRef
13. S. Inagaki, H. Fujimoto, and K. Fukui, J. Am. Chem. Soc., 1976, 98, 4693; CrossRef S. Inagaki and Y. Hirabayashi, ibid., 99, 7418. CrossRef
14. The MO calculation data are available for downloading at our website (http://pharm.ph.sojo-u.ac.jp/~kumayaku/)..
15. a) SIR92: A. Altomare, G. Cascarano, C. Giacovazzo, A. Guagliardi, M. Burla, G. Polidori, and M. Camalli, J. Appl. Cryst., 1994, 27, 435; CrossRef b) CrystalStructure 3.6.0: Crystal Structure Analysis Package, Rigaku and Rigaku/MSC, USA, 2000-2004; c) CRYSTALS Issue 10: D. J. Watkin, C. K. Prout, J. R. Carruthers, and P. W. Betteridge, Chemical Crystallography Laboratory, Oxford, UK., 1996.