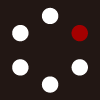
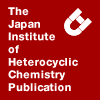
HETEROCYCLES
An International Journal for Reviews and Communications in Heterocyclic ChemistryWeb Edition ISSN: 1881-0942
Published online by The Japan Institute of Heterocyclic Chemistry
e-Journal
Full Text HTML
Received, 15th September, 2009, Accepted, 16th November, 2009, Published online, 17th November, 2009.
DOI: 10.3987/REV-09-660
■ Artificial Macrosphelides as a Novel Apoptosis-Inducing Compound
Yuji Matsuya* and Hideo Nemoto
Faculty of Pharmaceutical Sciences, Toyama University, 2630 Sugitani, Toyama, Toyama 930-0194, Japan
Abstract
Natural 16-membered macrolide compounds, macrosphelides, have recently been disclosed to exert an apoptosis-inducing effect against human lymphoma U937 cells. In this review, molecular design, syntheses, and biological evaluation of artificial macrosphelides are surveyed, focusing on their apoptosis-inducing activity.INTRODUCTION
Macrosphelides are natural macrolide compounds, characterized by a 16-membered trilactone linkage, which have been isolated from Macrosphaeropsis sp. FO-5050 and/or Periconia byssoides.1–5 Since the first isolation and structural elucidation in 1995, thirteen members of natural macrosphelides A—M have been reported to date.1–5 Some of these natural products have been reported to exhibit potent inhibitory activity against adhesion of human leukemia HL-60 cells to human-umbilical-vein endothelial cells (HUVECs).1–5 Adhesion of tumor cells to the vessel wall endothelium of distant organs is a critical step in tumor metastasis, and consequently, macrosphelides have been recognized as a promising lead compound for new anti-metastatic agents. In fact, macrosphelide B was reported to suppress metastasis of B16-BL6 mouse melanoma cells to the lung in vivo through inhibition of cell adhesion mediated by sialyl Lewisx.6 Combined therapy of macrosphelide B and cisplatin induced remarkably lung metastasis inhibition without adverse effect of cisplatin to the host.6 In addition, macrosphelide B and relating compounds was found to exert inhibitory activity against tumor cell growth (colon 26-L5 adenocarcinoma cells).7 Although these findings strongly suggest that macrosphelides can be a promising lead compound for development of a new anticancer chemotherapeutic agent, studies on the anticancer potential of macrosphelides as an index of apoptosis have not been carried out so far.
Apoptosis is a genetically regulated cellular suicide mechanism that plays an essential role in development and in defense of multicellular organism. Because apoptotic cell death has been recognized as an important aspect in the anticancer chemotherapy, regulation of apoptosis is a significant research target in developing novel anticancer agents. In this context, it was revealed that some of natural-type macrosphelides could induce apoptotic cell death of human lymphoma U937 cells.8 Moreover, combined treatment of macrosphelide and mild hyperthermia exhibited significant enhancement in apoptosis, compared with the cases of the compound and hyperthermia alone.9 Thus, drug design inspired by the natural macrosphelide structure may be a promising approach to discovery of a novel apoptosis-inducer.
While a number of studies on macrosphelide synthesis have been reported in past decades,10 a ring-closing metathesis (RCM)-based strategy for macrosphelide syntheses was developed in our laboratory.11,12 Broad applicability and good functional group compatibility of the RCM reaction for macrocycle formation would make the syntheses of diverse non-natural macrosphelide analogues possible. Thus, various artificial macrosphelide derivatives have been designed, synthesized, and assessed on their apoptosis-inducing activity.13–16
In this review, we account for an outline of our recent studies on macrosphelide-based artificial compounds focusing on the apoptosis-inducing activity.
EFFECTS OF NATURAL-TYPE MACROSPHELIDES ON APOPTOSIS INDUCTION8
Representative naturally-occurring macrosphelides (macrosphelide A, B, and E) and analogous compounds (macrosphelide core 1 and diketone 5) are shown in Figure 1. These compounds have a common 16-membered trilactone framework and different oxidation states at the 8- and 14-positions. Macrosphelide E (4) is a C3-epimer of macrosphelide A (2). We consider all of these compounds as “natural-type” macrosphelides. Among them, macrosphelide B (3) and diketone 5 were reported to exhibit anti-proliferative activity against colon 26-L5 cells, although macrosphelide core 1 and macrosphelide A (2) showed no activity.7 These facts suggest that higher oxidation states at the 8- and 14-positions are essential for exerting the antitumor effect. With these results in hand, we screened the compounds 1–5 for their ability to induce apoptosis. Human lymphoma U937 cells were used for the screening (incubation time: 12 h), and the results are shown in Figure 1. Although no activity was observed at 1 µM concentration, it was found that macrosphelide B (3) and diketone 5 clearly induced apoptotic cell death at 10 µM concentration. At higher concentration (100 µM), these compounds brought about secondary necrosis of the cells. The other macrosphelides (1, 2, and 4) did not exhibit any activities, which was parallel with the results obtained by the colon 26-L5 experiments. Figure 2 (A) shows that macrosphelide B (3) and diketone 5 induced DNA fragmentation in a time-dependent manner (10 µM concentration), which is a feature characteristic of apoptosis. In addition, growth of U937 cells was significantly inhibited by exposure with these compounds, especially diketone 5 (10 µM), as shown in Figure 2 (B). Thus, induction of apoptotic cell death may be, at least partially, responsible for the antitumor action of macrosphelide derivatives. Among the tested natural-type macrosphelides, diketone 5, which has the highest oxidation state at the 8- and 14-positions, exhibited the most potent activity.8 Additionally, we have found that the combined treatment of diketone 5 and mild hyperthermia shows significant synergistic enhancement of apoptosis in U937 cells.9
In conjunction with mechanism of action, we have observed following biological responses after treatment of the compound 5; transient increase in intracellular reactive oxygen species (ROS), glutathione (GSH) depletion, Bid activation, lipid peroxidation, mitochondrial membrane potential collapse, Fas externalization, caspase-8 activation, and increase in intracellular calcium ion concentration. All of these responses were completely inhibited by pretreatment of N-acetyl-L-cysteine (NAC), which was known as a GSH precursor and a protecting factor of cells from oxidative stress. These data suggested that a rapid and transient increase of intracellular oxidative stress triggered apoptosis of the U937 cells mainly via the Fas/caspase-8-mediated mitochondrial pathway.8
DESIGN and apoptosis-inducing activity of artificial macrosphelides13–16
Molecular design and syntheses of artificial compounds inspired by biologically active natural products, followed by extensive structure-activity relationship study, have been recognized as one of the most promising approaches for new drug discovery.17 In the light of the novel findings on the potential of natural macrosphelides as apoptosis inducer against tumor cells, we have gone on with a project of artificial macrosphelides, mainly from the following two points of view; (1) macrosphelide analogues with a different ring-size from the natural-type (16-membered ring),14,15 (2) macrosphelide-epothilone hybrid compounds with a heterocyclic side chain.13,15–16,18
(1) Macrosphelide Analogues with 15-Membered and 18-Membered Ring Skeletons14,15
Due to restricted conformational flexibility of macrocyclic compounds compared with open-chain molecules, a three-dimensional feature of the ensemble of possible conformations reflecting their relative stabilities is an important factor directing the biological behavior. Additionally, the two-dimensional array and the spatial arrangement of particular functional groups should play a crucial role for exerting the bioactivities. Therefore, investigation of the bioactivities of macrosphelide analogues with various ring-sizes, which preserve the natural array of functionality, may provide additional guidance for future design of a macrosphelide-based apoptosis inducer. We have thus undertaken synthesis and biological evaluation of several 15-membered and 18-membered macrosphelide analogues.
Our synthesis is outlined in Scheme 1, starting from readily available chiral blocks 6–9. Intermediates 10 and 11 were synthesized by sequential connection of 6–8, and intermediate 12 was obtained using 9 instead of 8. These intermediates were subjected to esterification and the RCM reaction to form macrocyclic products. The cyclization efficiently proceeded at room temperature in the presence of the 2nd Grubbs’ catalyst. After several transformations, macrosphelide analogues 13–20 were obtained.14,15
For the purpose of assessment of macrosphelide analogues on their apoptosis-inducing ability, DNA fragmentation assay was performed, and the results are summarized in Table 1. All experiments were carried out at 10 µM drug concentration using human lymphoma U937 cells, and the percentage of DNA fragmentation was determined after 12 h incubation. It was revealed that artificial macrosphelides having non-natural ring size did not show the apoptosis-inducing activity so much as the natural-type diketone 5 at this concentration. Among them, the compounds 16 and 20 showed rather week apoptosis-inducing activity, and the compound 14 exhibited the activity with maximum potency. At lower drug concentration (1 µM), however, this compound lost the activity (8.5% DNA fragmentation) as well as the diketone compound 5 (11.7% DNA fragmentation). Thus, promising new candidates possessing potent apoptosis-inducing activity over the natural-type macrosphelides have not been found at present in the series of artificial macrosphelide analogues with 15-membered and 18-membered ring skeletons.
(2) Macrosphelides Hybridized with Epothilones: Incorporation of Heterocyclic Side-chain13,15–16,18
Epothilones are 16-membered natural polyketides, which have been reported to show potent cytotoxicity in a broad range of human cancer cell lines through a paclitaxel-like mechanism of action.19–21 These natural macrolide compounds have been reported to take action against a tubulin dynamics and induce mitotic arrest at the G2/M transition as a result of aberrant spindle formation, leading to apoptotic cell death.19–21 One of the structural features of epothilones is a thiazole side-chain, which is supposed to play an important role as a hydrogen-bond acceptor when interacting with tubulin dimer.19–21
Although molecular mechanism of macrosphelides on apoptosis induction still remains unclear, we paid attention to the similarities of chemical structure (16-membered macrolide) and bioactivities (apoptosis induction of tumor cells) between macrosphelides and epothilones. Because hybridization of two or more biologically active natural products has been one of the most promising approaches for new lead discovery, we planned to the syntheses and biological evaluations of novel hybrid compounds, composed of the 16-membered trilactone core structure of macrosphelides and the thiazole-containing side chain of epothilones (Scheme 2).13,15 In addition, several heterocyclic side chains instead of the thiazole ring were also examined.16
Representative synthesis of hybrid compounds is shown in Scheme 3. The same chiral blocks 7 and 8 as used in Scheme 1 were effectively utilized for the syntheses, and heteroaromatic moiety was pre-installed as chiral blocks 21 which were prepared through asymmetric allylation reaction. Successive esterification of these blocks gave various RCM substrates such as 22, which were subjected to RCM using Grubbs’ catalyst to provide 16-membered macrosphelide skeleton such as 23. Subsequent derivatization afforded a variety of hybrid compounds. For example, hydrogenation of 23 over Rh/Al2O3 catalyst proceeded regioselectively to furnish 12,13-dihydro-type macrosphelide framework 24, which were found in natural macrosphelide I and L. Hybrid-type artificial macrosphelides synthesized so far are listed in Table 2.
The results of the DNA fragmentation assay of hybrid compounds are summarized in Table 2. At 10 µM concentration, 12,13-dihydro-type compounds 38 and 40, which have the thiazole side-chain at the 15-position, exhibited relatively high activity, but did not exceed the natural-type diketone 5. However, several hybrid compounds brought about better results in comparison with 5, at low dose conditions (1 µM concentration). Particularly, compound 26 showed the highest DNA fragmentation value (15.1%), being superior to 5 (11.7%). Later investigations revealed that the activities of the hybrid compound 26 were highly dependent on the drug concentration and the incubation time, and the percentage of DNA fragmentation reached over 50% at 3 µM concentration after 8 h incubation.18 This novel artificial macrosphelide analogue was found to exert potent anti-proliferative effects against human colon carcinoma (HCT116) and human gastric cancer (AGS) cells, while it had no effect against human normal dermal fibroblast.18 These biological data were superior to those of the natural-type macrosphelides.
Of course, we had an idea that introduction of an keto group onto the 14-position of the compound 26 may bring an improvement of the activities, because the 8,14-diketo derivative 5, having the highest oxidation states, exhibited the most potent activities among the natural-type macrosphelides. Upon this, 14-keto hybrid compounds 43 and 44 were synthesized utilizing the chiral block 42, which was prepared through an asymmetric hydroxyallylation reaction.22 As shown in Scheme 4, hydroxyallylation of the aldehyde 41 by diisopinocampheyl (Ipc) borane reagent proceeded successfully with excellent enantio- and diastereoselectivities.23 While efficient synthesis of 43 and 44 was achieved, biological results of these new hybrid compounds were disappointing; apoptosis detection 1.8% and 4.9% for 43 and 44, respectively, versus 29.2% for 26 (1 µM concentration, control experiment 1.5%).23
CONCLUSION
In this review, we took an overview of biological aspects of macrosphelides and relating artificial compounds, mainly including our recent researches. During the continuous studies, novel artificial compound 26, in which the thiazole side-chain found in natural epothilones is incorporated, has been found to possess significant biological properties. This compound is considered to be a promising candidate of new apoptosis-inducing agents against tumor cells. Because the macrosphelide skeleton consists of three ester linkages, various combinations of hydroxy-carboxylic acid equivalents make the syntheses of diverse artificial macrosphelide-like derivatives possible. Future development of studies in this area is deeply expected.
ACKNOWLEDGEMENTS
We greatly appreciate Prof. Takashi Kondo and co-workers for supports in biological experiments.
References
1. M. Hayashi, Y.-P. Kim, H. Hiraoka, M. Natori, S. Takamatsu, T. Kawakubo, R. Masuma, K. Komiyama, and S. Ômura, J. Antibiot., 1995, 48, 1435.
2. S. Takamatsu, Y.-P. Kim, M. Hayashi, H. Hiraoka, M. Natori, K. Komiyama, and S. Ômura, J. Antibiot., 1996, 49, 95.
3. T. Sunazuka, T. Hirose, Y. Harigaya, S. Takamatsu, M. Hayashi, K. Komiyama, S. Ômura, P. A. Sprengeler, and A. B. Smith, III, J. Am. Chem. Soc., 1997, 119, 10247. CrossRef
4. T. Yamada, M. Iritani, M. Doi, K. Minoura, T. Ito, and A. Numata, J. Chem. Soc., Perkin Trans. 1, 2001, 3046. CrossRef
5. T. Yamada, K. Minoura, R. Tanaka, and A. Numata, J. Antibiot., 2007, 60, 370. CrossRef
6. A. Fukami, K. Iijima, M. Hayashi, K. Komiyama, and S. Ômura, Biochem. Biophys. Res. Commun., 2002, 291, 1065. CrossRef
7. K. Ishihara, T. Kawaguchi, Y. Matsuya, H. Sakurai, I. Saiki, and H. Nemoto, Eur. J. Org. Chem., 2004, 3973. CrossRef
8. K. Ahmed, Q.-L. Zhao, Y. Matsuya, D.-Y. Yu, L. B. Feril Jr., H. Nemoto, and T. Kondo, Chem. Biol. Interact., 2007, 170, 86. CrossRef
9. K. Ahmed, Q.-L. Zhao, Y. Matsuya, D.-Y. Yu, T. L. Salunga, H. Nemoto, and T. Kondo, Int. J. Hyperthermia, 2007, 23, 353. CrossRef
10. For a review, Y. Matsuya and H. Nemoto, Heterocycles, 2005, 65, 1741, and references cited therein. CrossRef
11. Y. Matsuya, T. Kawaguchi, and H. Nemoto, Org. Lett., 2003, 5, 2939. CrossRef
12. T. Kawaguchi, N. Funamori, Y. Matsuya, and H. Nemoto, J. Org. Chem., 2004, 69, 505. CrossRef
13. Y. Matsuya, T. Kawaguchi, K. Ishihara, K. Ahmed, Q.-L. Zhao, T. Kondo, and H. Nemoto, Org. Lett., 2006, 8, 4609. CrossRef
14. Y. Matsuya, T. Matsushita, K. Sakamoto, and H. Nemoto, Heterocycles, 2009, 77, 483. CrossRef
15. Y. Matsuya, Y. Kobayashi, T. Kawaguchi, A. Hori, Y. Watanabe, K. Ishihara, K. Ahmed, Z.-L. Wei, D.-Y. Yu, Q.-L. Zhao, T. Kondo, and H. Nemoto, Chem. Eur. J., 2009, 15, 5799. CrossRef
16. Y. Matsuya, A. Hori, T. Kawamura, H. F. Emam, K. Ahmed, D.-Y. Yu, T. Kondo, N. Toyooka, and H. Nemoto, Heterocycles, 2010, 80, 579. CrossRef
17. L. F. Tietze, H. P. Bell, and S. Chandrasekhar, Angew. Chem. Int. Ed., 2003, 42, 3996. CrossRef
18. K. Ahmed, Y. Matsuya, H. Nemoto, S. F. H. Zaidi, T. Sugiyama, Y. Yoshihisa, T. Shimizu, and T. Kondo, Chem. Biol. Interact., 2009, 177, 218. CrossRef
19. K. C. Nicolaou, F. Roschangar, and D. Vourloumis, Angew. Chem. Int. Ed., 1998, 37, 2014. CrossRef
20. K.-H. Altmann, M. Wartmann, and T. O’Reilly, Biochim. Biophys. Acta, 2000, 1470, M79.
21. K.-H. Altmann, Curr. Pharm. Des., 2005, 11, 1595. CrossRef
22. P. V. Ramachandran, J. S. Chandra, and M. V. R. Reddy, J. Org. Chem., 2002, 67, 7547. CrossRef
23. Unpublished data.