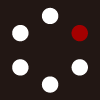
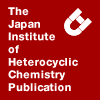
HETEROCYCLES
An International Journal for Reviews and Communications in Heterocyclic ChemistryWeb Edition ISSN: 1881-0942
Published online by The Japan Institute of Heterocyclic Chemistry
e-Journal
Full Text HTML
Received, 24th October, 2009, Accepted, 26th November, 2009, Published online, 27th November, 2009.
DOI: 10.3987/COM-09-11863
■ Synthesis of Optically Active γ-Valerolactone and γ-Nonanolactone via Optical Resolution Using Chiral Amine Derived from Amino Acid
Kenichi Yumoto, Morifumi Hasegawa, and Hiroaki Toshima*
Department of Bioresource Science, College of Agriculture, Ibaraki University, ,
Abstract
Optically active γ-valerolactone and γ-nonanolactone have been synthesized via optical resolution using a newly developed chiral amine derived from L-phenylalanine. Both racemic γ-lactones were transformed to corresponding diastereomeric amides by amidation with the optical resolution agent. Fractional crystallization of diastereomeric amides, recrystallization of each diastereomer, and subsequent hydrolysis gave optically active γ-valerolactone and γ-nonanolactone with sufficient enantiomeric excess and isolated yield. The optical resolution agent was recovered after hydrolysis.γ-Lactones exist widely in nature. Many γ-lactones exist as fragrance, flavor and odor of fruits.1 γ-Valerolactone is contained in strawberry and apricot with sweet, herbal and caramel odors. γ-Nonanolactone is contained in mango and raspberry with fatty-sweet, coconut and caramel odors.2,3 Other lactones are also known as pheromones of insects.4,5 These γ-lactones which were reported by many researchers regarding the bioactivities such as fragrance in fruits and pheromones in insects were usually optically active forms. Therefore, development of an efficient method to obtain optically active γ-lactones is an important subject for chemists.6,7 In addition, optically active γ-lactones are very useful chiral building blocks.8-12 For example, it is very difficult to synthesize optically active γ-hydroxyl carboxylic acid and γ-hydroxyl ester from corresponding γ-keto acid and γ-keto ester by asymmetric reduction. However, optically active γ-lactones are easily transformed to the corresponding optically active γ-hydroxyl carboxylic acids and γ-hydroxyl esters (Figure 1).13 Therefore, we have challenged the practical synthesis of optically active γ-lactones.
Reported synthetic routes to optically active γ-lactones were categorized into synthesis from natural products,14 enzymatic asymmetric synthesis,15 enzymatic resolution of racemic lactones,16 asymmetric synthesis17-19, and optical resolution using resolution agents.20 At the beginning of our study, we attempted the asymmetric reduction of methyl 4-oxopentanoate by using chiral oxazaborolidine-type catalyst21,22 that carried out asymmetric reduction of aliphatic23 and aromatic ketones.24 We have applied this method of asymmetric reduction of methyl 4-oxopentanoate to give methyl (R)-4-hydroxypentanoate with only 37% ee. We have also attempted the method using a chiral tartaric acid derivative and Zn(BH4)2 with some modifications of the reported procedure.25 This optical purity was only 30% ee of the same substrate. These asymmetric reductions did not perform sufficient asymmetric induction of γ-keto ester.
We tried optical resolution of γ-valerolactone to obtain both enantiomers. Although optical resolution of racemic compounds was a very classic method for not only γ-lactones but also carboxylic acids, it is useful method at the present time. Optical resolution of δ-decanolactone via amidation with (R)-(+)-1-phenylethylamine26 was reported to give the corresponding diastereomer with 97 - 98% de by Nohira et al.27 We attempted to produce diastereomeric N-(R)-1-phenylethyl-4-hydroxypentanamide which was obtained via ring opening amidation of γ-valerolactone (1) with (R)-(+)-1-phenylethylamine. Chromatographic separation (via silica gel column) of N-(R)-1-phenylethyl-4-hydroxypentanamide to each diastereomeric amide was difficult under several conditions. It might be found that the length of alkyl side chains affects to the optical resolution of simple lactones. Fractional crystallization was impossible for an oily diastereomeric amide mixture. However, we have succeeded in optical resolution of tricyclic lactone, which is the synthetic intermediate of diterpene syn-copalol,28 via ring opening amidation with (R)-(+)-1-phenylethylamine.29
To overcome the drawback by adding one asymmetric center, we designed a new optical resolution agent, (S)-2-amino-3-phenyl-N-[(R)-1-phenylethyl]propanamide (3) that was derived from L-phenylalanine (Figure 2). Because each diastereomer of N-(R)-1-phenylethyl-4-hydroxypentanamide possesses only two chiral centers originating from amine and lactone, the difference in physical properties of diastereomers might not be so clear. As we have succeeded with complicated tricyclic lactone which possesses multiple chiral centers, the resulting diastereomers can be separated easily by adding one asymmetric center. Based on our experiences,29 we designed a new optical resolution agent (3) that has multiple chiral centers. The new optical resolution agent (3) was reported as supporting ligands in Ru catalyst.30) L-Phenylalanine was protected by a tert-butoxycarbonyl (Boc) group before amidation with (R)-(+)-1-phenylethylamine. The amidation between (R)-(+)-1-phenylethylamine and N-Boc-L-phenylalanine was carried out with dicyclohexylcarbodiimide and 1-hydroxybenzotriazole in CH2Cl2 (Figure 2). The Boc group was deprotected under acidic conditions.
Ring opening amidation of γ-valerolactone (1) and 3 with trimethylaluminum gave (S)-2-[4-hydroxypentanamide]-3-phenyl-N-[(R)-1-phenylethyl]propanamide (4) (Figure3).
Although we attempted silica gel chromatography (CHCl3 : n-hexane = 2 : 1, Rf = 0.3) for the separation of diastereomeric amides (4), it was difficult to separate each diastereomer. Next we tried fractional crystallization on 4 (798 mg) from mixed solution (CHCl3 : n-hexane). A colorless crystal (4-a) (300 mg, 38%) that was separated out was determined to be 65% de ((R)-form rich) by chiral HPLC using a Daicel Chiralcel OD-H column. The absolute configuration at the C-4 position of diastereomeric amides ((4R)-4 and (4S)-4) were determined by specific rotation of corresponding γ-valerolactones ((R)-1 and (S)-1) that were obtained after hydrolysis. After three additional recrystallizations, a colorless crystal ((4R)-4e) (116 mg, 15%, > 99% de) was obtained. This second colorless crystal (4-d) (480 mg, 60%) that was crystallized from mother liquor at the fractional crystallization of 4 was 28% de ((S)-form rich). After four additional recrystallizations, a colorless crystal ((4S)-4) (116 mg, 15%, 94% de) was obtained. Diastereomers mixture (4) (504 mg, 63%) was collected from the remaining solution. We have applied 3 for optical resolution of γ-nonanolactone (2) possessing a longer alkyl side chain than that of γ-valerolactone (1). The corresponding diastereomeric amide (5) was prepared by same method as described for 4. The fractional crystallization on 5 (600 mg) from mixed solution (CHCl3 : n-hexane) gave a colorless crystal (5-a) (260 mg, 43%, 64% de, (R)-form rich). The absolute configuration at the C-4 position of (4R)-5 and (4S)-5 were determined by specific rotation of the corresponding γ-nonanolactones ((R)-2 and (S)-2) that were obtained after hydrolysis. After two additional recrystallizations, a colorless crystal ((4R)-5) (91 mg, 15%, 99% de) was obtained. This second colorless crystal (5-c) (340 mg, 57%) that was crystallized from mother liquor at the fractional crystallization of 5 was 47% de ((S)-form rich). After an additional recrystallization, a colorless crystal ((4S)-5) (50 mg, 8%, > 99% de) was obtained. Diastereomers mixture (5) (420 mg, 70%) was collected from the remaining solution. We succeeded in separating diastereomeric amide (4) prepared from γ-valerolactone (1) (short alkyl side chain) and 5 prepared from γ-nonanolactone (2) (long alkyl side chain) by using the newly designed optical resolution agent (3).
To obtain the optically active γ-lactones, we attempted hydrolysis on the corresponding diastereomeric amides ((4R)-4, (4S)-4, (4R)-5 and (4S)-5) under usual condition (6 M HCl in MeOH, reflux) (Figure 4). All four diastereomeric amides were exclusively transformed to the corresponding γ-lactones ((R)-1), ((S)-1), ((R)-2) and ((S)-2) without producing γ-hydroxyl carboxylic acids. The specific rotations of obtained ((R)-1), ((S)-1), ((R)-2) and ((S)-2) were almost identical to the literature values. In addition, the optical resolution agents (3) cleaved from the above four diastereomeric amides were recovered after hydrolysis with > 90% yield.
Optically active γ-valerolactone (1) and γ-nonanolactone (2) with sufficient enantiomeric excess were obtained by fractional crystallization of the corresponding diastereomeric amides (4 and 5) that were produced by amidation with a newly designed optical resolution agent (3) and subsequent hydrolysis. After hydrolysis, the optical resolution agent (3) was recovered with > 90% yield. Obtained optically active γ-lactones can be applied to produce artificial fruit flavors.3 In addition, obtained optically active γ-lactones are not only useful building blocks, but also useful starting materials for the syntheses of γ-hydroxyl carboxylic acids and γ-hydroxyl esters.8-12 Due to our success of optical resolution of two γ-lactones with different alkyl side chain lengths, the optical resolution agent (3) is expected to apply to other γ-lactones as well. We have also been studying optical resolution of δ-lactones by using 3 with similar results as γ-lactones.
EXPERIMENTAL
General Experimental Procedures.
NMR spectra were measured with a JEOL FT NMR JNM-A500 spectrometer. Chemical shifts of 1H NMR spectra were referenced to tetramethylsilane (0.0 ppm). Chemical shifts of 13C NMR spectra were referenced to the center signal of CD3Cl (77.0 ppm). Optical purity and diastereomeric excess were measured with a Shimadzu LC9A, SPD-6AV HPLC system by using a Daicel Chiralcel OD-H column (250 4.6 mm, 5 µm). Enantiomeric excess of methyl 4-hydroxypentanoate was analyzed by following HPLC conditions; UV detection, 210 nm; eluent, 2-PrOH : n-hexane = 1 : 100, flow rate, 0.5 mL/min; column temperature, 30 °C. Diastereomeric excess of several diastereomeric amides were analyzed by following HPLC conditions; UV detection, 254 nm; eluent, 2-PrOH : n-hexane = 5 : 95, flow rate, 1 mL/min; column temperature, 30 °C. MS and HRMS were measured with a JEOL JMS-GC mate II/B. Specific rotation was measured with a JASCO P-2100 polarimeter by using a 1.8 mm 100 mm cell at 589 nm. IR spectra were measured with a JASCO FT/IR-4100 spectrometer.
(S)-2-[N-Boc-Amino]-3-phenyl-N-[(R)-1-phenylethyl]propanamide.
A solution of (R)-(+)-1-phenylethylamine (472 mg, 3.90 mmol) in CH2Cl2 (4 mL) was added to a solution of N-Boc-L-phenylalanine (1007 mg, 3.80 mmol), 1-hydroxybenzotriazole (510 mg, 3.77 mmol) and dicyclohexylcarbodiimide (784 mg, 3.80 mmol) in CH2Cl2 (4 mL) at room temperature. The mixture was stirred at room temperature overnight. After reaction, insoluble materials were removed by filtration. The filtrate was evaporated under reduced pressure to give a yellow crude oil, which was purified by silica gel column chromatography (n-hexane : EtOAc = 2 : 1). (S)-2-[N-Boc-Amino]-3-phenyl-N-[(R)-1- phenylethyl]propanamide was obtained (1260 mg, 3.42 mmol, 90%). 1H NMR (500 MHz, CDCl3): δ 7.30-7.16 (10H), 5.87 (d, 1H, J = 7.9 Hz), 5.07 (m, 1H), 4.99 (m, 1H), 4.28 (m, 1H), 3.12 (dd, 1H, J = 13.5, 6.1 Hz), 2.99 (dd, 1H, J = 13.5, 8.1 Hz), 1.41 (s, 9H), 1.29 (d, 3H, J = 6.4 Hz); 13C NMR (126 MHz, CDCl3): δ 170.2, 155.5, 142.9, 136.9, 129.4, 128.6, 128.5, 127.2, 126.8, 126.0, 80.0, 56.0, 48.7, 38.6, 28.3, 21.7; IR (KBr) cm-1: 3367, 2925, 2854, 1652, 1540, 1456, 1376, 1165, 1048; MS (EI) m/z 368 (M+, 10), 312 (16), 295 (4), 251 (11), 220 (6), 177 (6), 164 (59), 146 (3), 120 (100), 90 (11), 73 (10); HRMS (EI) calcd for C22H28N2O3 368.2100, found 368.2107; mp 98-99 °C; [α]D25 +40.1° (c 0.61, MeOH).
(S)-2-Amino-3-phenyl-N-[(R)-1-phenylethyl]propanamide (3).
A solution of 4 M HCl in MeOH (10 mL) was added to a solution of (S)-2-[N-Boc-amino]-3-phenyl-N- [(R)-1-phenylethyl]propanamide (1260 mg, 3.42 mmol) in MeOH (2 mL) at room temperature and mixed for 1 h. The mixture was neutralized with saturated aqueous NaHCO3. The solution was extracted with EtOAc, washed with brine and dried over anhydrous Na2SO4. The extract was evaporated under reduced pressure to give colorless oil which was purified by silica gel column chromatography (EtOAc). A colorless compound (3) was obtained (890 mg, 3.32 mmol, 97%). 1H NMR (500 MHz, CDCl3): δ 7.46 (d, 1H, J = 8.2 Hz), 7.27-7.17 (10H), 5.10 (m, 1H), 3.65 (dd, 1H, J = 8.7, 4.6 Hz), 3.20 (dd, 1H, J = 13.8, 4.6 Hz), 2.75 (dd, 1H, J = 13.8, 8.7 Hz), 1.45 (d, 3H, J = 7.0 Hz); 13C NMR (126 MHz, CDCl3): δ 173.2, 143.3, 137.8, 129.4, 129.3, 128.6, 128.5, 127.1, 126.7, 126.0, 56.3, 48.2, 40.9, 21.9; IR (KBr) cm-1: 3309, 3029, 2925, 1651, 1517, 1500, 1454, 754, 700; MS (EI) m/z 268 (M+, 3), 251 (1), 217 (10), 191 (2), 177 (11), 160 (30), 149 (4), 120 (100), 105 (32), 90 (12), 77 (9); HRMS (EI) calcd for C17H20N2O 268.1576, found 268.1571; [α]D25 +92 ° (c 0.70, MeOH), lit.,30 [α]D25 +100 ° (c 1.00, CH2Cl2)).
(S)-2-[4-Hydroxypentanamide]-3-phenyl-N-[(R)-1-phenylethyl]propanamide (4).
A solution of 2 M trimethylaluminum in toluene (3 mL, 6.00 mmol) was added to a solution of 3 (662 mg, 2.47 mmol) in CH2Cl2 (5 mL) at 0 °C. The solution was stirred at 0 °C for 1 h. Then the solution of γ-valerolactone (1) (250 mg, 2.50 mmol) in CH2Cl2 (4 mL) was added to the solution at room temperature. The mixture was stirred at room temperature overnight. Then the reaction was quenched with water. The solution was extracted with EtOAc, washed with brine and dried over anhydrous Na2SO4. The extract was evaporated under reduced pressure to give colorless oil, which was purified by silica gel column chromatography (EtOAc). A colorless compound (4) was obtained (810 mg, 2.20 mmol, 89%).
(S)-2-[(R)-4-Hydroxypentanamide]-3-phenyl-N-[(R)-1-phenylethyl]propanamide ((4R)-4).
Solidified 4 (798 mg) was dissolved in CHCl3 (71 mL). Insoluble materials were filtered off. n-Hexane (120 mL) was added to the resulting CHCl3 solution at room temperature. After storing the solution at 4 °C overnight, a colorless crystal (4-a) (300 mg, 38%, 65% de ((R)-form rich) was separated out. Recrystallization of 4-a (CHCl3 30 mL + n-hexane 45 mL at 4 °C overnight) gave a colorless crystal (4-b) (145 mg, 18%, 86% de). Recrystallization of 4-b (CHCl3 15 mL + n-hexane 22 mL at 4 °C overnight) gave a colorless crystal (4-c) (128 mg, 16%, 94% de). Recrystallization of 4-c (CHCl3 13 mL + n-hexane 19 mL at room temperature for 1 h) gave a colorless crystal ((4R)-4) (116 mg, 15%, > 99% de). 1H NMR (500 MHz, CDCl3): δ 7.34-7.14 (10H), 6.32 (d, 1H, J = 8.5 Hz), 5.94 (d, 1H, J = 8.2 Hz), 4.95 (m, 1H), 4.60 (m, 1H), 3.69 (m, 1H), 3.15 (dd, 1H, J = 13.6, 6.1 Hz), 2.98 (dd, 1H, J = 13.6, 9.2 Hz), 2.31 (m, 1H), 2.26 (m, 1H), 1.74 (m, 2H), 1.27 (d, 3H, J = 7.0 Hz) , 1.15 (d, 3H, J = 6.1 Hz); 13C NMR (126 MHz, CDCl3): δ 173.5, 169.7, 142.8, 136.9, 129.4, 128.8, 128.6, 127.4, 127.1, 126.0, 67.5, 54.7, 49.0, 38.5, 34.1, 33.2, 23.7, 21.7; IR (KBr) cm-1: 3277, 2969, 2928, 1643, 1555, 1496, 1454, 745, 699; MS (EI) m/z 368 (M+, 2), 350 (4), 292 (2), 268 (2), 251 (11), 221 (12), 202 (16), 120 (100), 105 (45), 91 (10), 73 (10); HRMS (EI) calcd for C22H28N2O3 368.2100, found 368.2085; mp 140-142 °C; [α]D26 + 25 ° (c 0.75, MeOH); Retention time (tR), 16.2 min.
(S)-2-[(S)-4-Hydroxypentanamide]-3-phenyl-N-[(R)-1-phenylethyl]propanamide ((4S)-4).
This second colorless crystal (4-d) (480 mg, 60%) that was crystallized from mother liquor at the fractional crystallization of 4 was 28% de ((S)-form rich). Recrystallization of 4-d (CHCl3 48 mL + n-hexane 74 mL at 4 °C overnight) gave a colorless crystal (4-e) (380 mg, 48%, 44% de). Recrystallization of 4-e (CHCl3 34 mL + n-hexane 76 mL at 4 °C overnight) gave a colorless crystal (4-f) (230 mg, 29%, 75% de). Recrystallization of 4-f (CHCl3 21 mL + n-hexane 46 mL at room temperature for 1 h) gave a colorless crystal (4-g) (145 mg, 18%, 90% de). Recrystallization of 4-g (CHCl3 13 mL + n-hexane 29 mL at room temperature for 1 h) gave a colorless crystal ((4S)-4) (116 mg, 15%, 94% de). Diastereomers mixture (4) (504 mg, 63%) was collected from the remaining solution. 1H NMR (500 MHz, CDCl3): δ 7.34-7.14 (10H), 6.32 (d, 1H, J = 7.9 Hz), 5.85 (d, 1H, J = 7.3 Hz), 4.95 (m, 1H), 4.59 (m, 1H), 3.75 (m, 1H), 3.15 (dd, 1H, J = 13.6, 5.9 Hz), 2.96 (dd, 1H, J = 13.6, 8.5 Hz), 2.32 (m, 2H), 1.76-1.73 (2H), 1.26 (d, 3H, J = 7.0 Hz) , 1.15 (d, 3H, J = 6.1 Hz); 13C NMR (126 MHz, CDCl3): δ 173.4, 169.7, 142.8, 136.8, 129.3, 128.7, 128.6, 127.3, 127.0, 126.0, 67.2, 54.7, 49.0, 38.6, 34.1, 33.0, 23.6, 21.7; IR (KBr) cm-1: 3285, 2970, 2925, 1638, 1540, 1496, 1450, 746, 700; MS (EI) m/z 368 (M+, 5), 350 (11), 292 (11), 251 (12), 221 (8), 202 (46), 120 (100), 105 (90), 91 (39), 73 (52); HRMS (EI) calcd for C22H28N2O3 368.2100, found 368.2107; mp 147-148 °C; [α]D26 + 35 ° (c 0.70, MeOH); tR, 13.4 min.
(S)-2-[4-Hydroxynonanamide]-3-phenyl-N-[(R)-1-phenylethyl]propanamide (5).
A solution of 2 M trimethylaluminum in toluene (3 mL, 6.00 mmol) was added to a solution of 3 (886 mg, 3.30 mmol) in CH2Cl2 (5 mL) at 0 °C. The solution was stirred at 0 °C for 1 h. Then the solution of γ-nonanolactone (2) (514 mg, 3.29 mmol) in CH2Cl2 (4 mL) was added to the solution at room temperature. The mixture was stirred at room temperature overnight. Then the reaction was quenched with water. The solution was extracted with EtOAc, washed with brine and dried over anhydrous Na2SO4. The extract was evaporated under reduced pressure to give colorless oil, which was purified by silica gel column chromatography (EtOAc). A colorless compound (5) was obtained (1309 mg, 3.09 mmol, 93%).
(S)-2-[(R)-4-Hydroxynonanamide]-3-phenyl-N-[(R)-1-phenylethyl]propanamide ((4R)-5).
Solidified 5 (600 mg) was dissolved in CHCl3 (40 mL) at room temperature. n-Hexane (60 mL) was added to the CHCl3 solution. The solution was stored at 4 °C overnight. After filtration, a colorless crystal (5-a) (260 mg, 43%, 64% de, (R)-form rich) was separated out. Recrystallization of 5-a (CHCl3 17 mL + n-hexane 26 mL at 4 °C overnight) gave a colorless crystal (5-b) (160 mg, 27%, 91% de). Recrystallization of 5-b (CHCl3 10 mL + n-hexane 16 mL at room temperature for 1 h) gave a colorless crystal ((4R)-5) (91 mg, 15%, 99% de). 1H NMR (500 MHz, CDCl3): δ 7.33-7.15 (10H), 6.25 (d, 1H, J = 7.9 Hz), 5.96 (d, 1H, J = 6.7 Hz), 4.97 (m, 1H), 4.61 (m, 1H), 3.48 (m, 1H), 3.16 (dd, 1H, J = 13.4, 6.0 Hz), 2.98 (dd, 1H, J = 13.4, 8.4 Hz), 2.34 (m, 1H), 2.27 (m, 1H), 1.78 (m, 2H), 1.23-1.26 (8H), 1.27 (d, 3H, J = 7.0 Hz), 0.89 (t, 3H, J = 7.0 Hz); 13C NMR(126 MHz, CDCl3): δ 173.6, 169.7, 142.8, 136.9, 129.4, 128.8, 128.6, 127.4, 127.1, 126.0, 71.6, 54.6, 49.0, 38.4, 37.8, 33.3, 32.4, 31.8, 25.3, 22.6, 21.7, 14.0; IR (KBr) cm-1 : 3309, 3029, 2924, 1652, 1518, 1500, 1454, 753, 703; MS (EI) m/z 424 (M+, 2), 406 (5), 353 (3), 315 (6), 277 (20), 251 (24), 231 (3), 201 (3), 168 (11), 120 (100), 105 (98), 61 (20), 55 (33); HRMS (EI) calcd for C26H36N2O3 424.2726, found 424.2717; mp 143-144 °C; [α]D26 + 19 ° (c 1.86, MeOH); tR, 11.7 min.
(S)-2-[(S)-4-Hydroxynonanamide]-3-phenyl-N-[(R)-1-phenylethyl]propanamide ((4S)-5).
This second colorless crystal (5-c) (340 mg, 57%) that was crystallized from mother liquor at the fractional crystallization of 5 was 47% de ((S)-form rich). Recrystallization of 5-c (CHCl3 23 mL + n-hexane 34 mL at 4 °C overnight) gave a colorless crystal ((4S)-5) (50 mg, 8%, > 99% de). Diastereomers mixture (5) (420 mg, 70%) was collected from the remaining solution. 1H NMR (500 MHz, CDCl3): δ 7.33-7.14 (10H), 6.35 (d, 1H, J = 7.3 Hz), 5.89 (d, 1H, J = 7.3 Hz), 4.95 (m, 1H), 4.59 (m, 1H), 3.53 (m, 1H), 3.16 (dd, 1H, J = 13.7, 6.0 Hz), 2.96 (dd, 1H, J = 13.7, 8.4 Hz), 2.32 (m, 2H), 1.78 (m, 2H), 1.23-1.25 (8H), 1.26 (d, 3H, J = 7.0 Hz) , 0.88 (t, 3H, J = 6.9 Hz); 13C NMR (126 MHz, CDCl3): δ 173.6, 169.8, 142.8, 136.8, 129.4, 128.7, 128.6, 127.3, 127.0, 126.0, 71.2, 54.7, 49.0, 38.5, 37.6, 33.0, 32.3, 31.8, 25.3, 22.6, 21.7, 14.0; IR (KBr) cm-1 : 3309, 3029, 2924, 1651, 1516, 1500, 1454, 756, 701; MS (EI) m/z 424 (M+, 1), 406 (3), 353 (2), 315 (3), 277 (8), 251 (10), 231 (2), 201 (2), 168 (6), 120 (100), 105 (45), 61 (19), 55 (19); HRMS (EI) calcd for C26H36N2O3 424.2726, found 424.2723; mp 155-156 °C; [α]D26 + 34 ° (c 1.12, MeOH); tR, 9.3 min.
(R)-Valerolactone ((R)-1).
A solution of 6 M HCl in MeOH (20 mL) was added to the solution of (4R)-4 (795 mg, 2.16 mmol) in MeOH (6 mL) at room temperature. The mixture was stirred for 3 h under reflux. Then mixture was cooled down at room temperature and neutralized with saturated aqueous NaHCO3. The mixture was extracted with EtOAc, washed with brine and dried over anhydrous Na2SO4. The extract was evaporated under reduced pressure to give colorless oil. Purification of the obtained colorless oil by silica gel column chromatography (EtOAc) gave colorless oil ((R)-1) (200 mg, 2.00 mmol, 93%, [α]D22 +29 ° (c 1.00, MeOH), lit.,6 [α]D23 +30 ° (c 0.85, CH2Cl2)) and 3 (558 mg, 2.08 mmol, 96%). 1H NMR (500 MHz, CDCl3): δ 4.65 (m, 1H), 2.53-2.57 (2H), 2.37 (m, 1H), 1.84 (m, 1H), 1.42 (d, 3H, J = 6.4 Hz); 13C NMR(126 MHz, CDCl3): δ 177.1, 77.3, 29.6, 29.0, 20.9; IR (KBr) cm-1: 2981, 2879, 1776, 1461, 1422, 1388, 1345, 1302, 1280, 1225, 1198, 1173, 1121, 1094, 1057, 999, 942, 826, 803; MS (EI) m/z 100 (M+, 13), 99 (19), 85 (99), 83 (78), 72 (28), 70 (43), 57 (100); HRMS (EI) calcd for C5H8O2 100.0524, found 100.0535.
(S)-Valerolactone ((S)-1).
Using the same method as described for (R)-1, the separated amide ((4S)-4) (362 mg, 0.98 mmol) was transformed to (S)-1 (98 mg, 0.98 mmol, > 99%, [α]D23 -29 ° (c 1.00, MeOH), lit.,6 [α]D23 -30 ° (c 1.29, CH2Cl2)) and 3 (260 mg, 0.97 mmol, 99%). 1H NMR (500 MHz, CDCl3): δ 4.65 (m, 1H), 2.54-2.57 (2H), 2.36 (m, 1H), 1.83 (m, 1H), 1.42 (d, 3H, J = 6.4 Hz); 13C NMR(126 MHz, CDCl3): δ 177.1, 77.3, 29.6, 29.0, 20.9; IR (KBr) cm-1: 2980, 2876, 1774, 1461, 1422, 1387, 1345, 1302, 1281, 1225, 1197, 1173, 1121, 1094, 1058, 999, 942, 828, 804; MS (EI) m/z 100 (M+, 15), 99 (14), 85 (100), 83 (66), 72 (22), 70 (35), 57 (93); HRMS (EI) calcd for C5H8O2 100.0524, found 100.0532.
(R)-Nonanolactone ((R)-2).
Using the same method as described for (R)-1, the separated amide ((4R)-5) (512 mg, 1.21 mmol) was transformed to (R)-2 (187 mg, 1.20 mmol, 99%, [α]D22 + 44 ° (c 2.00, MeOH), lit.,9 [α]D24 + 48 ° (c 1.82, MeOH)) and 3 (231 mg, 0.86 mmol, 99%). 1H NMR (500 MHz, CDCl3): δ 4.49 (m, 1H), 2.54-2.51 (2H), 2.32 (m, 1H), 1.85 (m, 1H), 1.74 (m, 1H), 1.59 (m, 1H), 1.30-1.49 (6H), 0.90 (t, 3H, J = 6.9 Hz); 13C NMR(126 MHz, CDCl3): δ 177.2, 81.0, 35.5, 31.4, 28.8, 27.9, 24.8, 22.4, 13.8; IR (KBr) cm-1: 2957, 1776, 1461, 1354, 1184, 1127, 1022, 915, 804; MS (EI) m/z 156 (M+, 12), 138 (37), 128 (65), 114 (64), 101 (52), 100 (96), 99 (79), 86 (89), 85 (100), 83 (55), 70 (46), 58 (84), 57 (98); HRMS (EI) calcd for C9H16O2 156.1150, found 156.1162.
(S)-Nonanolactone ((S)-2).
Using the same method as described for (R)-1, the separated amide ((4S)-5) (480 mg, 1.13 mmol) was transformed to (S)-2 (172 mg, 1.10 mmol, 97%, [α]D22 -46 ° (c 2.00, MeOH), lit.,9 [α]D24 -48 ° (c 1.70, MeOH)) and 3 (240 mg, 0.89 mmol, 97% ). 1H NMR (500 MHz, CDCl3): δ 4.49 (m, 1H), 2.55-2.52 (2H), 2.32 (m, 1H), 1.85 (m, 1H), 1.74 (m, 1H), 1.60 (m, 1H), 1.30-1.49 (6H), 0.90 (t, 3H, J = 6.9 Hz); 13C NMR(126 MHz, CDCl3): δ 177.2, 81.0, 35.5, 31.4, 28.8, 27.9, 24.8, 22.4, 13.8; IR (KBr) cm-1: 2957, 1776, 1461, 1355, 1183, 1127, 1022, 915, 801; MS (EI) m/z 156 (M+, 13), 138 (32), 128 (51), 114 (45), 101 (27), 100 (65), 99 (44), 86 (12), 85 (100), 83 (58), 70 (23), 58 (47), 57 (63); HRMS (EI) calcd for C9H16O2 156.1150, found 156.1143.
ACKNOWLEDGEMENTS
We appreciate Eiweiss Chemical Corporation for supplying di-tert-butyl dicarbonate.
References
1. E. Guichard, ‘Fruit Flavors: Chiral γ-Lactones, Key Compounds to Apricot Flavor,’ ed. by R. L. Rouseff and M. M. Leahy, American Chemical Society Publication, 1995, pp. 258-267.
2. A. Mosandl and C. Günther, J. Agric. Food Chem., 1989, 37, 413. CrossRef
3. A. Mosandl, U. Hener, U. Hangenauer-Hener, and A. Kustermann, J. Agric. Food Chem., 1990, 38, 767. CrossRef
4. Y. Naoshima, H. Hasegawa, and T. Saeki, Agric. Biol. Chem., 1987, 51, 3417.
5. J. H. Tumlinson, M. G. Klein, R. E. Doolittel, T. L. Ladd, and A. T. Proveaux, Science, 1997, 197, 789. CrossRef
6. K. Mori, Tetrahedron, 1975, 31, 3011. CrossRef
7. J. Kula, M. Sikora, H. Sadowska, and J. Piwowarski, Tetrahedron, 1996, 52, 11321. CrossRef
8. E. Santaniello, P. Ferraboschi, P. Grisenti, and A. Manzocchi, Chem. Rev., 1992, 92, 1071. CrossRef
9. Y. Miyashita and K. Mori, Agric. Biol. Chem., 1981, 45, 2521.
10. J. I. Brauman and A. J. Pandell, J. Am. Chem. Soc., 1967, 89, 5421. CrossRef
11. G. Juszkiewicz, M. Asztemborska, and J. Jurczak, Polish J. Chem., 2002, 76, 1701.
12. H. J. Gorissen, J. V. Hoeck, A. M. Mockel, G. H. Journée, C. Delatour, and V. R. Libert, Chirality, 1992, 4, 286. CrossRef
13. T. Osawa, E. Mieno, T. Harada, and O. Takayasu, J. Mol. Catal. A., 2003, 200, 315. CrossRef
14. C. Papageorgiou and C. Benezra, Tetrahedron Lett., 1984, 25, 6041. CrossRef
15. A. L. Gutman, K. Zuobi, and A. Boltansky, Tetrahedron Lett., 1987, 28, 3861. CrossRef
16. Y. Shimotori and T. Miyakoshi, J. Oleo Sci., 2006, 55, 629.
17. H. C. Brown, S. V. Kulkarni, and U. S. Racherla, J. Org. Chem., 1994, 59, 365. CrossRef
18. I. Ojima, T. Kogure, and M. Kumagai, J. Org. Chem., 1977, 42, 1671. CrossRef
19. L. Thijs, P. P. Waanders, E. H. M. Stokkingreef, and B. Zwanenburg, Recl. Trav. Chim. Pays-Bas., 1986, 105, 332.
20. K. Tanaka, D. Kuchiki, and M. R. Caira, Tetrahedron: Asymmetry, 2006, 17, 1678. CrossRef
21. E. J. Corey and C. J. Helal, Angew. Chem. Int. Ed., 1998, 37, 1986. CrossRef
22. S. Itsuno and K. Ito, J. Org. Chem., 1984, 49, 555. CrossRef
23. S. Itsuno M. Nakano, K. Miyazaki, H. Masuda, and K. Ito, J. Chem. Soc., Perkin Trans. 1, 1985, 2039. CrossRef
24. S. Itsuno, K. Ito, A. Hirano, and S. Nakahama, J. Chem. Soc., Chem. Commun., 1983, 469. CrossRef
25. K. D. Sugi, T. Nagata, T. Yamada, and T. Mukaiyama, Chem. Lett., 1996, 1081. CrossRef
26. E. Juaristi, J. Escalante, J. L. León-Romo, and A. Reyes, Tetrahedron: Asymmetry, 1998, 9, 715. CrossRef
27. H. Nohira, K. Mizuguchi, T. Murata, Y. Yazaki, M. Kanazawa, Y. Aoki, and M. Nohira, Heterocycles, 2000, 52, 1359. CrossRef
28. H. Toshima, H. Oikawa, H Yada, H. Ono, T. Toyomasu, and T. Sassa, Biosci. Biotechnol. Biochem., 2002, 66, 2504. CrossRef
29. H. Toshima, M. Hasegawa, H. Nakajima and R. Kawamoto, unpublished results.
30. P. Pelagatti, M. Carcelli, F. Calbiani, C. Cassi, L Elviri, C Pelizzi, U Rizzotti, and D. Rogolino, Organometallics, 2005, 24, 5836. CrossRef