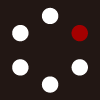
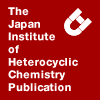
HETEROCYCLES
An International Journal for Reviews and Communications in Heterocyclic ChemistryWeb Edition ISSN: 1881-0942
Published online by The Japan Institute of Heterocyclic Chemistry
e-Journal
Full Text HTML
Received, 30th June, 2010, Accepted, 10th August, 2010, Published online, 12th August, 2010.
DOI: 10.3987/COM-10-S(E)83
■ Modification of D-Ring Moiety of Steroids − A Novel Palladium Catalyzed Baeyer-Villiger Type Rearrangement of Cyclic Silylenol Ether Derivatives
Minoru Tamiya,* Futa Takada, Nobuhisa Isaka, Nahoko Iimura, and Masaji Ishiguro*
Chemical Biology Laboratory, Department of Applied Life Sciences, Niigata University of Pharmacy and Applied Life Sciences, 265-1, Higashijima, Niigata 956-8603, Japan
Abstract
A novel Baeyer–Villiger type rearrangement reaction of cyclic silylenol ether derived from dehydroepiandrosterone via oxygen and catalytic amount of palladium acetate in the presence of DMSO has been developed. The reaction proceeded at low temperature and high pressure of oxygen to afford corresponding α,β-unsaturated lactones in moderate yields.Steroids are ubiquitous in many biologically important compounds including natural products, clinical medicines, and hormones.1 Because of these reasons, many methods and unique reactions for the modification of steroidal skeletons2 have been developed. Due to the rigidity of the skeleton and the stereochemical property of steroid, the steroidal reactions sometime make themselves very unique. Here we report the unexpected and unique side-reaction, which may demand steroidal backbone of the reactant, found during the course of an oxidative conversion of steroid. Thus, the treatment of cyclic silylenol ether derivative (1)3 under the Larock protocol of Saegusa oxidation,4 using catalytic amount of Pd(OAc)2 (0.1 equiv.)5 and O2 as re-oxidant of palladium(0) in DMSO at 60 °C, gave desired α,β-enone 2 in 75% yield along with a side-product in 6% (Scheme 1; Table 1, entry 1). However, the side-product was not obtained when p-benzoquinone was used as a re-oxidant of palladium(0). This result suggested that O2 probably plays a critical role in the formation of the side-product. According to 1H and 13C NMR, IR, and Mass spectra, the side-product was anticipated as α,β-unsaturated lactone 3.6 To confirm this prospect, the side-product was treated with catalytic amount of Pd/C under hydrogen gas. The product of the hydrogenation showed spectroscopic properties identical to lactone 4 derived from ketone 5. Two possible reaction pathways could be envisioned from these results: 1) Baeyer–Villiger rearrangement proceeded after the formation of the corresponding enone; 2) Baeyer–Villiger type rearrangement occurred before unsaturation of the α,β-position. For the determination of the reaction pathway, enone 2 was subjected to catalytic amount of Pd(OAc)2 and O2 at atmosphere, which resulted in no reaction (Scheme 1). According to these results mentioned above, lactone 4 was probably produced by Baeyer–Villiger type rearrangement of corresponding ketone followed by the unsaturation of α,β-position of carbonyl via β-hydride elimination of palladium in one pod. Because the conversion of cyclic ketone to the corresponding α,β-unsaturated lactone by using catalytic amount of bivalent palladium and O2 is completely a novel transformation to our knowledge, we investigated to gain a further insight into this reaction.
We investigated the reaction conditions for producing lactone 3 as a major product. Concerning the reaction temperature, the silylenol ether (1) was treated with Pd(OAc)2 (0.1 equiv.) and O2 (1 atm) in DMSO (0.06 M) at room temperature to afford lactone 3 in 35% yield along with enone 2 in 45% yield (Table 1, entry 2). These results indicated that low temperature would be necessary for the formation of lactone 3. Because it is impossible to perform the reaction at lower temperature due to high freezing point of DMSO (16~19 °C), other solvent should be applied for the investigation. For the alternative solvent, we examined DMF, DMA, and CH2Cl2 that, unfortunately, gave low yields of lactone 3 (entries 3–5). We next examined CH2Cl2 as a co-solvent of DMSO, which could stand from freezing at low temperature (DMSO/CH2Cl2=1/1, fp. ~–20 °C). To make a comparison between DMSO and DMSO/CH2Cl2 co-solvent system, the reaction was performed at room temperature to afford lactone 3 in 37% yield along with enone 2 in 42% yield (entry 6). With the ideal solvents in hand, we next examined the reaction at 0 °C and –15 °C (entries 7, 8). In these conditions, lactone 3 (ca. 43%) was major product to enone 2 (ca. 35%). Further enhancement of the yield of lactone 3 (ca. 50%) was observed in more diluted conditions (0.06 M to 0.03 M) (entries 9, 10).
The other trimethylsilylenol ethers 6 and 7 possessing tri-substituted double bond at C5–C6 position (Δ5) were also converted to lactone (B) in 54% and 56% yield, respectively, along with enone (A) in 38% and 30% yield without any touch of Δ5 (Scheme 2). Since compound 10 formed single crystals suitable for X-ray analysis, the structure of 10 was unambiguously established by the single-crystal X-ray analysis.7 The triethylsilylenol ether 8 and the trans-fused bicyclic compound 138 were also converted to enone (9: 46% and 14: 47%) and lactone (10: 44% and 15: 19%) at 0 °C, while the cis-fused compound 16 was quickly converted solely to enone 17 in 85% yield without any trace of lactone 18. This suggests that the trans junction of CD ring moiety of steroid is suitable for the rearrangement.
In an attempt to increase the yield of lactone, we then examined a variety of Pd catalysts (Table 2), comparing with Pd(OAc)2 (entry 1). The Pd(II) catalysts such as PdCl2 and Pd(COCF3)2 were not effective to afford the lactone (entries 2 and 3), whereas Pd(0) catalysts such as Pd(PPh3)4 and Pd(dba)2 were able to give the lactone (entries 4–7). These results indicate that Pd(II) species resulted from the oxidation of Pd(0) by O2 are possibly important intermediates for the formation of the lactone. Among the catalysts examined, Pd(OAc)2 was the most suitable reagent for this reaction.
All the results described above indicate that O2 is important in this rearrangement reaction. Thus, we next examined the effects of O2 pressure (Table 3). Although 8 atm O2 atmosphere showed no significant difference in a yield of lactone 10 at room temperature and/or 0 °C (entry 1), the treatment of silylenol ether 6 at lower temperature (0 °C and –15 °C) gave lactone 10 in a yield of 53% and 61% respectively exceeding the yield of enone 9 (entries 2 and 3). Furthermore, the reaction at 28 atm gave the best result, affording lactone 10 in 70% yield (entry 5) although the reaction at 20 atm gave only a little improvement of a yield of lactone 10 (63%, entry 4). It is noted that this reaction also proceeded with 5 mol% of Pd(OAc)2 (entry 6).
Based on the above results, we anticipate that formation of palladium(II) complex I-b by the reaction of silylenol ether and three-membered palladium peroxide,9 resulting from palladium catalytic cycle, followed by cyclization of I-b, rearrangement of III, and β-hydride elimination of alkylpalladium complex IV gave lactone B as outlined in Scheme 3. The high pressure of O2 probably accelerated the formation of palladium peroxide and low temperature may avoid the β-hydride elimination pathway from I-b to II. The conformation of the trans-fused CD-ring may allow the formation of the intermediate III (see, 13,14-trans-I-b) whereas cis-fused CD-ring system (see, 13,14-cis-I-b) would disturb the formation of the intermediate III due to the steric hindrance at the β-face of the 5-membered ring. However, the other possible reaction mechanism cannot be ruled out.
In summary, we have developed Baeyer–Villiger type rearrangement reaction of silylenol ether derivatives promoted by 5 mol% of Pd (OAc)2 at high pressure of O2 and low temperature. At this point, the substrates of this rearrangement reaction were limited to the 17-oxosteroid derivatives; however, more scopes of this reaction are now under investigation.
ACKNOWLEDGEMENTS
Generous financial support by the Grant-in-Aid for Young Scientists (B) from JSPS (to M.T.) is gratefully acknowledged.
References
1. D. A. Williams and T. L. Lemke, 'Foye’s Principals of Medicinal Chemistry (fifth edition)', Lippincott Williams & Wilkins, Philadelphia, 2002, pp. 653–717; D. A. Williams and T. L. Lemke, 'Comprehensive Natural Products Chemistry', Vol. 2, ed. by D. E. Cane, Elsevier, Amsterdam, 1999, pp. 267–318.
2. D. N. Kirk and M. P. Hartshorn, 'Steroid Reaction Mechanisms', Elsevier, Amsterdam, 1968, pp. 24–438.
3. Compound 1, 2, and 3 are all contaminated with 3-OMe derivatives 6, 9, and 10 respectively in the ratio of 9:1. For the synthesis of compound 5, see: H. Suginome, Y. Nakayama, and H. Senboku, J. Chem. Soc., Perkin Trans. 1, 1992, 1837. CrossRef
4. R. C. Larock and N. H. Lee, Tetrahedron Lett., 1991, 31, 5911; CrossRef R. C. Larock, T. R. Hightower, G. A. Kraus, P. Hahn, and D. Zheng, Tetrahedron Lett., 1995, 36, 2423. CrossRef
5. Contaminated by-products were removed by filtration. Thus, after dissolution of Pd(OAc)2 in hot benzene and its filtration, the filtrate was concentrated in vacuo to give pure Pd(OAc)2.
6. Chemical data of 3: 1H NMR (500 MHz, CDCl3): δ=6.86 (dd, J = 2.1, 9.9 Hz, 1H), 6.04 (dd, J = 2.9, 9.9 Hz, 1H), 3.37 (s, 3H), 2.89 (t, J = 2.9 Hz, 1H), 2.38–2.30 (m, 1H), 2.23 (td, J = 2.9, 13.2 Hz, 1H), 2.01 (td, J = 3.4, 12.0 Hz, 1H), 1.90–1.70 (m, 4H), 1.63–1.51 (m, 2H), 1.44–1.32 (m, 1H), 1.38 (s, 3H), 1.25–1.18 (m, 1H), 1.12–1.05 (m, 1H), 1.02 (s, 3H), 0.98–0.89 (m, 2H), 0.74–0.71 (m, 1H), 0.54 (dd, J = 5.1, 8.0 Hz, 1H) ppm; 13C NMR (125 MHz, CDCl3): δ=164.0, 145.5, 121.5, 83.6, 81.4, 56.7, 48.1, 47.6, 43.5, 38.2, 34.6, 34.0, 33.2, 30.6, 24.7, 23.2, 21.1, 19.1, 18.5, 13.2 ppm; IR (NaCl): ṽ = 2946, 2869, 1722, 1457, 1381, 1097, 816 cm–1; MS (EI): m/z (%): 316 (14)[M+], 301 (45), 284 (37), 261 (100), 243 (11), 226 (5), 211 (8), 159 (20), 123 (15), 105 (22), 91 (31), 79 (25), 55 (16).
7. The structure of lactone 10 was determined by X-ray crystallographic analysis. CCDC-788111 contains the supplementary crystallographic data for this paper. These data can be obtained free of charge from the Cambridge Crystallographic Data Centre via www.ccdc.cam.ac.uk/data̲request/cif.
8. A. R. Daniewski and J. Kiegiel, Synthetic Commun., 1988, 18, 115; W. Peng, P. Tang, X. Hu, J. O. Liu, and B. Yu, Bioorg. Med. Chem. Lett., 2007, 17, 5506. CrossRef
9. J. E. Lyons, 'Oxygen Complexes and Oxygen Activation by Transition Metals', A. E. Martell, D. T. Sawyer, Eds., Plenum Press, New York, 1988, pp. 233; G. Wilke, H. Schott, P. Heimbach, Angew. Chem. Int., Ed. Engl., 1967, 6, 92; CrossRef for a review of palladium catalytic cycle, see: J. Muzart, Chem. Asian J., 2006, 1, 508. CrossRef
10. General Procedure: The reactions were carried out in an HPG-10-1 (Taiatsu Techno®) autoclave. To a solution of silylenol ether 6 (99.2 mg, 0.265 mmol) in CH2Cl2/DMSO (1/1, 12 mL) was added Pd(OAc)2 (2.8 mg, 0.012 mmol) at –15 °C. After O2 was pumped into the autoclave to reach 28 atm, the reaction mixture was stirred for 141 h at –15 °C. After venting O2, the reaction was stopped by adding brine and the mixture was extracted with EtOAc (×3). The combined organic extracts were washed with saturated aqueous NaHCO3 and brine, dried (Na2SO4), and concentrated in vacuo. The residue was purified by column chromatography (hexane/EtOAc = 8/2) to afford 9 (19.9 mg, 25%) as a white solid and 10 (59.5 mg, 73%) as a white solid.