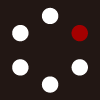
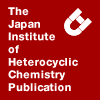
HETEROCYCLES
An International Journal for Reviews and Communications in Heterocyclic ChemistryWeb Edition ISSN: 1881-0942
Published online by The Japan Institute of Heterocyclic Chemistry
e-Journal
Full Text HTML
Received, 30th June, 2010, Accepted, 29th July, 2010, Published online, 2nd August, 2010.
DOI: 10.3987/COM-10-S(E)82
■ A Molecular Orbital Calculation Study on the Interesting Reactivity of Fluorine-Containing 3,4-Dihydro-2H-pyrans with Aromatic Compounds in the Presence of Trifluoroacetic Acid
Norio Ota, Yasuhiro Kamitori,* Eisuke Nishiguchi, Makoto Ishii, and Etsuji Okada*
Department of Chemical Science and Engineering, Graduate School of Engineering, Kobe University, 1-1 Rokkodai-cho, Nada-ku, Kobe 657-8501, Japan
Abstract
The reaction of 6-trifluoromethyl-3,4-dihydro-2H-pyrans (1) with aromatic compounds in trifluoroacetic acid gave 4-arylated dihydropyrans (3) selectively, whereas that of 5-trifluoroacetyl-6-trifluoromethyl-3,4-dihydro-2H-pyrans (2) with aromatic compounds in refluxing trifluoroacetic acid afforded the ring-opening products (4) via exclusive attack of aromatic compounds at the 2-position of 2. These two interesting reactions affording the quite different products from the similar substrates were elucidated on the basis of DFT calculations.INTRODUCTION
In recent years, much attention has been focused on development of new methodologies for the syntheses of various kinds of fluorine-containing heterocycles since these compounds show some interesting and useful biological activities in life-science fields.1-4
In the course of our researches concerning syntheses and reactions of novel fluorine-containing heterocycles, we found that 2,4-diethoxy-6-trifluoromethyl-3,4-dihydro-2H-pyran (1)5 reacted with aromatic compounds in trifluoroacetic acid at ambient temperature to give 4-arylated 3,4-dihydro-2H-pyran derivatives (3) selectively,6 while the ring-opening reaction of 2-ethoxy-4-isobutoxy-5-trifluoroacetyl-6-trifluoromethyl-3,4-dihydro-2H-pyran (2)7 was proceeded to afford 1,1,1,5,5,5-hexafluoro-3-[(E)-3-propylidene]pentane-2,4-diones (4)8 by the reaction with aromatic compounds in refluxing trifluoroacetic acid via exclusive nucleophilic attack of aromatic compounds at the 2-position of 2 (Scheme 1). Additionally, (E)-4-aryl-1,1,1-trifluorobut-3-en-2-ones (3’) were easily obtained by retro hetero Diels-Alder reaction of dihydropyrans (3) in high yields.6 These reactions present useful fluorine-containing C4- and C8-unit (2-trifluoroacetyl vinyl and 4,4-bis(trifluoroacetyl)-1,3-butadienyl systems) introduction methods to various aromatic compounds.
Moreover, enones (3’), and butadienones (4) are useful synthetic building blocks to access a variety of novel fluorine-containing heterocycles. In other words, both reactions shown in Scheme 1 are one of the key steps to construct a broad range of novel fluorine-containing heterocyclic systems.
Here we present interesting acid catalyzed reactions of above two dihydropyran derivatives with aromatic compounds along with the results of molecular orbital calculations. The interesting reactivities of two dihydropyrans, 1 and 2 similar to each other affording the quite different products, 3 and 4, respectively, is elucidated on the basis of DFT calculations.
RESULTS AND DISCUSSION
The reactions of dihydropyrans (1 and 2) with various aromatic compounds occurred in trifluoroacetic acid, which is assumed to be proceeded via the corresponding pyryliums (5 and 6) produced by the elimination of alcohols from 1 and 2 (Scheme 2). In the case of the reaction of 1 at ambient temperature, aromatic compounds would attack the 4-position of 5 selectively to give 4-arylated 4H-pyrans (7a) and consequently afford 4-arylated dihydropyrans (3) as shown in Path A.
In contrast, in the case of the reaction of 2 in refluxing trifluoroacetic acid, the attack of aromatic compounds would occur at the 6-position of pyrylium (6) selectively to give 2H-pyrans (8b) followed by electrocyclic ring-opening to afford butadienones (4) through Path B.
First, we carried out the structural optimization of 5 and 6 using RB3LYP/6-31G* to clarify the reasons why the nucleophilic attack of aromatic compounds occurs selectively at the 4-position of pyrylium (5) and at the 6-position of pyrylium (6). The results are summarized in Figure 1 where the C-C bond lengths and Mulliken bond populations (in parentheses) of the each pyrylium rings of 5 and 6 are indicated together with their energy values. The frontier electron densities of LUMO (frN) on C-6 and C-4, and the LUMO levels of these two pyryliums are also described in Figure 1.
In the both cases of 5 and 6, the values of frN on C-4 are over 0.2 larger than those on C-6. These results suggest that the nucleophilic attack by aromatic nucleus carbon exclusively takes place at C-4 in the both cases of 5 and 6 to give the corresponding intermediates 7a and 8a followed by the formation of final products 3 and 10, respectively, under the kinetically controlled conditions. These predictions are concordant with the experimental results in the case of the reaction of dihydropyrans (1) with aromatic compounds. In contrast to this, above predictions for the reaction of 2 with aromatic compounds are not compatible with the experimental results showing that 1,3-dienes (4) were obtained via 2H-pyrans (8b) selectively without any formation of dihydropyrans (10).
We also estimated the most stable structures of the intermediates 7a,b and 8a,b together with their energy values using benzene as a model of aromatic compounds (Figure 2). The intermediate (7ap) is estimated to be 0.6 kcal/mol more stable than 7bp. This result suggests that the reaction of pyrylium (5) with benzene gives 7ap predominantly under thermodynamically controlled conditions. Therefore, it seems to be reasonable that dihydropyrans (3) were obtained selectively from 1, even if the reaction was controlled thermodynamically. However, the energy difference no more than 0.6 kcal/mol between 7ap and 7bp also predicts the formation of 7bp as a minor product (ca. 30%) in addition to 7ap (ca. 70%),9 which is inconsistent with the experimental results showing no formation of butadienones (9) derived from intermediates (7b). The reaction of pyryliums (5) with aromatic compounds in trifluoroacetic acid would be controlled kinetically to afford 7a.
Meanwhile, intermediate 8bp was calculated to be 2.4 kcal/mol more stable than 8ap. This relatively large energy difference suggests that the reaction of pyrylium (6) with benzene gives 8bp with high selectivity (ca. 98%).9 This prediction is concordant with the experimental results very well. Since the reaction was carried out in refluxing trifluoroaceticacid, the reaction of 2 with aromatic compounds
would be controlled thermodynamically to afford 8b and, consequently, butadienones (4) selectively.
Finally, we also estimated the transition state structure on the electrocyclic ring-opening process from the intermediates (8b) to 1,3-dienes (4). In Figure 3, the optimized transition state structure (TS8; Ar= Ph) and its energy are illustrated together with the most stable structure of 4 (Ar= Ph) and its energy. The energy difference between 8bp and TS8 (Ar= Ph) corresponding to the activation energy of this process was estimated to be ca. 12 kcal/mol. The final product (4; Ar= Ph) was calculated to be ca. 7 kcal/mol more stable than 8bp. The above results suggest that the smooth ring-opening of the intermediate (8b) will be occurred at the temperature of refluxing trifluoroacetic acid to afford 4 irreversibly.
CONCLUSION
The interesting reactions of two dihydropyrans (1) and (2) with aromatic compounds in trifluoroacetic acid giving 4-arylated dihydropyrans (3) and butadienones (4), respectively, could be reasonably interpreted as followings on the basis of DFT calculations. The selective formation of 3 from 1 at ambient temperature could be attributed to the LUMO controlled regioselective attack of aromatic compounds onto the 4-position of pyrylium (5). In contrast to this, the exclusive formation of 4 from 2 under reflux conditions could be rationalized by the thermodynamically controlled reaction of pyrylium (6) with aromatic compounds.
Meanwhile, the results also predict the possible reaction of 1 with aromatic compounds in refluxing trifluoroacetic acid to give butadienones (9) and that of 2 with aromatic compounds in trifluoroacetic acid at lower temperature accessing novel dihydropyrans (10). Further work is under progress on the syntheses of 9 and 10 to confirm above predictions as well as on the synthetic application of 3 and 4 to various fluorine-containing heterocycles having potential biological importance.
COMPUTATIONAL METHODS
All calculations employed in this paper were accomplished using the computer programs packages PC SPARTAN 02 and PC SPARTAN 04.10 All calculations for geometrical optimizations were performed with the 6-31G* basis set at B3LYP 11 level. The starting geometries employed for all optimizations were resulted from molecular mechanics using SYBYL12 force field and subsequent semi-empirical PM313 optimizations. The calculations for transition state geometries and their energies were also taken with the 6-31G* basis set at B3LYP level.
References
1. R. Filler and Y. Kobayashi, ‘Biomedicinal Aspects of Fluorine Chemistry,’ Kodansha & Elsevier Biomedical, Tokyo, 1982.
2. R. Filler, ‘Organofluorine Chemicals and Their Industrial Applications,’ Ellis Horwood, London, 1979.
3. J. T. Welch, Tetrahedron, 1987, 43, 3123. CrossRef
4. R. Filler, Y. Kobayashi, and L. M. Yagupolskii, ‘Organofluorine Compounds in Medicinal Chemistry and Biomedical Applications,’ Elsevier, Amsterdam, 1993.
5. M. Hojo, R. Masuda, and E. Okada, Synthesis, 1989, 215. CrossRef
6. N. Ota, E. Okada, D. Shibata, S. Adachi, and S. Saikawa, Heterocycles, 2010, 80, 515. CrossRef
7. M. Hojo, R. Masuda, and E. Okada, Synthesis, 1990, 347. CrossRef
8. N. Ota, E. Okada, A. Sonoda, N. Muro, D. Shibata, and M. Médebielle, Heterocycles, 2008, 76, 215. CrossRef
9. The ratios of two products 7ap / 7bp and 8ap / 8bp were estimated using the Boltzmann equation.
10. Wavefunction, Inc.
11. A. D. Becke, J. Chem. Phys., 1993, 98, 5648. CrossRef
12. M. Clark, R. D. Cramer III, and N. van Opdensch, J. Computational Chem., 1989, 10, 982. CrossRef
13. J. J. P. Stewart, J. Computer Aided Molecular Design, 1992, 6, 69