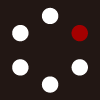
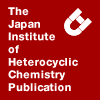
HETEROCYCLES
An International Journal for Reviews and Communications in Heterocyclic ChemistryWeb Edition ISSN: 1881-0942
Published online by The Japan Institute of Heterocyclic Chemistry
e-Journal
Full Text HTML
Received, 16th June, 2010, Accepted, 16th August, 2010, Published online, 17th August, 2010.
DOI: 10.3987/COM-10-S(E)59
■ The Preparation of Ketene Dithioacetals and Thiophenes from Chloropyridines Containing an Active Methylene Group
Keith R. Sturrock,* David H. Bremner, and Grant Wishart
School of Contemporary Sciences, University of Abertay Dundee, Bell Street, Dundee, DD1 1HG
Scotland, U.K.
Abstract
The base catalysed reaction of carbon disulphide with the active methylene groups of chloropyridines 4 and 7, followed by alkylation with reagents which also contain active methylene groups, lead to ketene dithioacetals. Further reaction with base afforded highly substituted thiophenes.INTRODUCTION
Our work1-3 on the synthesis of thienopyridines required us to prepare a series of α-halogenated pyridines containing a methylene moiety activated by an electron withdrawing group. We earlier reported1,2 that reaction of pyridine 3-acetonitrile N-oxide 1 with refluxing POCl3, using the method reported by Okuda and Robison4 gave the desired chloropyridines 3 and 5 with 4 as a byproduct. Similarly2 we obtained 6, 7 and 8 from ethyl 3-pyridylacetate N-oxide 2 (Equation 1). The ratio1, 2 of isomers differs for each substituent at the 3-position (Table 1). It is well known5-12 that polyfunctional ketene dithioacetals are available from reaction of carbon disulphide with functionalized carbionic species followed by alkylation of the heteroanions including cyano(3-pyridyl)ketene dimethyldithioacetal11 from 3-cyanomethylpyridine. Furthermore, it has also been reported5-10 that treatment of appropriately functionalized ketene dithioacetals with base allows entry into a range of highly substituted heterocycles. In this work, haloalkanes with electron withdrawing groups on the same carbon as the halogen were employed as alkylating agents in the base catalysed nucleophilic addition reaction of 4 and 7 with carbon disulphide. This gave ketene dithioacetals with the required functionality for cyclisation to form thiophenes.
RESULTS AND DISCUSSION
When 2-chloro-5-cyanomethylpyridine 4 and carbon disulphide were treated with a slight excess of sodium hydride in DMSO under anhydrous conditions followed by addition of ethyl chloroacetate, ketene dithioacetal 9 was synthesised in 58% yield. The infrared spectrum showed the molecule to contain a nitrile group (2209 cm-1) and an aliphatic ester (1736 cm-1). The 1H NMR corroborated the structure, showing signals for two ethyl thioacetate groups and a 2,5 disubstituted pyridine. Further treatment of 9 with sodium ethoxide in ethanol gave thiophene 10 (55%). The infrared spectrum for this compound showed a primary amine (3447 and 3343 cm-1) and two ester groups (1724, 1661 cm-1). The absorbance at 1724 cm-1 can be interpreted as being due to the aliphatic ethyl ester. The second, at 1661cm-1 can be assigned to the ester directly attached to the thiophene ring, having a similar absorption wavenumber as an anthranilate ester. There will be some hydrogen bonding between the ester carbonyl and the amino hydrogens and this phenomenon is known13 to move the absorbance to a lower wavenumber. It is thought that the mechanism for the formation of 9 proceeds by base catalysed abstraction of the methylene protons of 4 and subsequent formation, with carbon disulphide, of a ketene dithioacetal dianion. These anions are then alkylated with ethyl chloroacetate. The thiophene 10 is subsequently formed by a Thorpe14 type cyclisation of the active methylene adjacent to the sulphur onto the nitrile. Ketene ditioacetal 11 was similarly prepared from 4 (54%) using chloroacetonitrile as the alkylating agent and 11 was readily cyclised to the corresponding thiophene 12 (51%) with sodium ethoxide again probably via a Thorpe type reaction.
This methodology was extended to ethyl 2-chloro-5-pyridylacetate 7, to produce ketene dithioacetal 13. The infrared spectrum showed a carbonyl stretching absorbance in the expected region for aliphatic esters (1736 cm-1). This was unexpected, as it also contains an α,β-unsaturated ethyl ester group and a second peak should have been observed at a lower wavenumber, typically,13 1730-1705 cm-1. The 1H NMR however supported the structure, showing signals for two ethyl thioacetate groups and an ethyl ester group. Further reaction of 13 with sodium ethoxide in ethanol lead to the production of thiophene 14 in 33% yield. Again two carbonyl stretching absorbances were observed in the IR spectrum, an aliphatic ester (1748 cm-1), and a salicylate type ester at 1666cm-1. It is presumed that formation is by a Dieckmann15 cyclisation of one of the methylene groups onto the vinyl ester. Reaction of 7 with chloroacetonitrile under the same reaction conditions, gave 15 in 57% yield. The IR spectrum this time showed a carbonyl stretching absorbance typical13 of an α,β-unsaturated ester group (1720 cm-1). Further reaction of 15 with base afforded 16 in 46% yield, again by a Dieckmann cyclisation.
In conclusion, novel ketene dithioacetals can be conveniently prepared, leading to polyfunctional thiophenes (Scheme 1) and we believe this method can be applied in the synthesis of a variety similar highly substituted thiophenes.
EXPERIMENTAL
1.0. General
Melting points were determined using an Electrothermal melting point apparatus and are uncorrected. Infrared spectra were recorded on a Perkin-Elmer Paragon spectrophotometer. 1H NMR spectra were recorded on a Jeol PMX 60i spectrometer. Column chromatography was performed using pressurised short path columns with Kieselgel 60, particle size < 0.063 mm (Merck No. 7729) and reactions were monitored with Merck DC-Alufoilien 60 F254 (Merck No. 5554) which were visualised by ultraviolet irradiation.
1.1. Preparation of ketene dithioacetals.
1.1.1. General procedure; 2-chloro-5-(1-cyano-2,2-di(ethyloxycarbonylmethylthio)-1-vinyl)pyridine (9) as an example.
2-Chloro-5-cyanomethylpyridine 4 (2.0 g, 13.1 mmol) and carbon disulphide (1.1 g, 14.4 mmol) were dissolved in DMSO (40 mL) in a 2-neck round bottom flask fitted with a gas inlet tube under dry nitrogen and sodium hydride (0.66 g, 27.5 mmol) was added in portions with constant stirring and the mixture was stirred for 2 h. Ethyl chloroacetate (3.38 g, 27.5 mmol) was added and stirring continued for another hour, then poured onto ice (100 g) and extracted with EtOAc (3 x 50 mL). The extracts were combined, dried with magnesium sulphate, filtered and the solvent evaporated to give a pale yellow oil (4.46 g) which was chromatographed on silica gel using petroleum ether/EtOAc to give 9 as a colourless oil (58%). Bp (decomp); Anal. Calcd for C16H17ClN2O4S2: C, 47.9; H, 4.3; N, 7.0%. Found: C, 48.0; H, 4.4; N, 7.1%; IR νmax (film)/cm-1 2982, 2209, 1736; 1H NMR δH (CDCl3) 1.28 (3H, t, J=7.2 Hz, CH3), 1.32 (3H, t, J=7.2 Hz, CH3), 3.72 (2H, s, SCH2), 3.80 (2H, s, SCH2), 4.16 (2H, q, J=7.2 Hz, CO2CH2), 4.20 (2H, q, J=7.2 Hz, CO2CH2), 7.33 (1H, d, J=8.4 Hz, H-3), 7.85 (1H, dd, J=8.4 & 2.4 Hz, H-4), 8.52 (1H, d, J=2.4 Hz, H-6).
1.1.2. 2-Chloro-5-(1-cyano-2,2-di(cyanomethylthio)-1-vinyl)pyridine (11)
Off white crystals (61%, EtOAc). Mp 101-102 °C; Anal. Calcd for C11H7ClN4S2: C, 47.0; H, 2.4; N, 18.3%. Found: C, 46.9; H, 2.3; N, 18.3%; IR νmax(KBr)/cm-1 2978, 2234; 1H NMR δH (CDCl3) 4.48 (4H, s, SCH2), 7.38 (1H, d, J=8.4 Hz, H-3), 7.66 (1H, dd, J=8.4 & 2.4 Hz, H-4), 8.32 (1H, d, J=2.4 Hz, H-6).
1.1.3. 2-Chloro-5-(1-ethyloxycarbonyl-2,2-di(ethyloxycarbonylmethylthio)-1-vinyl)pyridine (13)
Colourless oil (43%); bp (0.4) 190 °C;Anal. Calcd for C18H22ClNO6S2: C, 49.3; H, 4.9; N, 3.1%. Found: C, 49.6; H, 4.8; N, 3.3%; IR νmax(film)/cm-1 2982, 2937, 1736; 1H NMR δH (CDCl3) 1.24 (3H, t, J=7.2 Hz, CH3), 1.28 (6H, t, J=7.2 Hz, CH3), 3.56 (2H, s, SCH2), 3.68 (2H, s, SCH2), 4.08 (4H, q, J=7.2 Hz, CO2CH2), 4.14 (2H, q, J=7.2 Hz, CO2CH2), 7.28 (1H, d, J=8.4 Hz, H-3), 7.72 (1H, dd, J=8.4 & 2.4 Hz, H-4), 8.34 (1H, d, J=2.4 Hz, H-6).
1.1.4. 2-Chloro-5-(1-ethyloxycarbonyl-2,2-di(cyanomethylthio)-1-vinyl)pyridine (15)
Pale yellow needles (57%, EtOH). Mp 98-99 °C; Anal. Calcd for C14H12ClN3O2S2: C, 47.5; H, 3.4; N, 11.9%. Found: C, 47.4; H, 3.0; N, 11.7%; IR νmax(KBr)/cm-1 2976, 2246, 1720; 1H NMR δH (CDCl3) 1.25 (3H, t, J=7.2 Hz, CH3), 3.56 (2H, s, SCH2), 3.68 (2H, s, SCH2), 4.24 (2H, q, J=7.2 Hz, CO2CH2), 7.24 (1H, d, J=8.4 Hz, H-3), 7.58 (1H, dd, J=8.4 & 2.4 Hz, H-4), 8.24 (1H, d, J=2.4 Hz, H-6).
1.2. Preparation of thiophenes
1.2.1. General procedure; 2-chloro-5-(2-ethyloxycarbonyl-5-ethyloxycarbonylmethylthio-3- aminothienyl)pyridine (10) as an example.
Ketene dithioacetal 9 (0.5 g, 1.1 mmol) was dissolved in EtOH (20 mL) in a 50 mL round bottom flask and sodium ethoxide (0.16 g, 2.4 mmol) added. The mixture was stirred at room temperature for 24 h. The solvent was removed in vacuo, the residue flooded with water and extracted with EtOAc (3 x 25 mL). The combined extracts were dried with magnesium sulphate, filtered and the solvent removed to give thiophene 10 (55%) after recrystallisation from EtOAc. Mp 94-95 °C; Anal. Calcd for C16H17ClN2O4S2: C, 47.9; H, 4.3; N, 7.0%. Found: C, 48.1; H, 4.2; N, 6.9%; IR νmax(KBr)/cm-1 3447, 3343, 2967, 1724, 1661; 1H NMR δH (CDCl3) 1.17 (3H, t, J=7.2 Hz, CH3), 1.26 (3H, t, J=7.2 Hz, CH3), 3.46 (2H, s, SCH2), 4.02 (2H, q, J=7.2 Hz, CO2CH2), 4.18 (2H, q, J=7.2 Hz, CO2CH2), 5.36 (2H, s, NH2), 7.30 (1H, d, J=8.4 Hz, H-3), 7.64 (1H, dd, J=8.4 & 2.4 Hz, H-4), 8.26 (1H, d, J=2.4 Hz, H-6).
1.2.2. 2-Chloro-5-(2-cyano-5-cyanomethylthio-3-aminothienyl)pyridine (12)
Pale yellow crystals (51%, EtOAc). Mp 113-114 °C; Anal. Calcd for: C12H7ClN3S2: C, 49.2; H, 2.4; N, 14.4%. Found: C, 49.1; H, 2.4; N, 14.2%; IR νmax(KBr)/cm-1 3443, 3339, 2982, 2210; 1H NMR δH (CDCl3) 4.38 (2H, s, SCH2), 5.42 (2H, s, NH2), 7.32 (1H, d, J=8.4 Hz, H-3), 7.68 (1H, dd, J=8.4 & 2.4 Hz, H-4), 8.36 (1H, d, J=2.4 Hz, H-6).
1.2.3. 2-Chloro-5-(2-ethyloxycarbonyl-5-ethyloxycarbonyl methylthio-3-hydroxythienyl)pyridine (14)
Pale yellow crystals (33%, EtOAc). Mp 74-75 °C; Anal. Calcd for: C16H16ClNO5S2: C; 47.8; H, 4.0; N, 3.4%. Found: C, 47.8; H, 3.7; N, 3.3%; IR νmax(KBr)/cm-1 3462, 2978, 2914, 1748, 1666; 1H NMR δH (CDCl3) 1.20 (3H, t, J=7.2 Hz, CH3), 1.32 (3H, t, J=7.2 Hz, CH3), 3.48 (2H, s, SCH2), 4.02 (2H, q, J=7.2 Hz, CO2CH2), 4.24 (2H, q, J=7.2 Hz, CO2CH2), 7.22 (1H, d, J=8.4 Hz, H-3), 7.66 (1H, dd, J=8.4 & 2.4 Hz, H-4), 8.34 (1H, d, J=2.4 Hz, H-6), 9.70 (1H, s, OH).
1.2.4. 2-Chloro-5-(2-cyano-5-cyanomethylthio-3-hydroxythienyl)pyridine (16)
Pale yellow prisms (46%, EtOAc). Mp 101-102 °C; Anal. Calcd for C12H6ClN3OS2: C, 46.8; H, 1.9; N, 13.6%. Found: C, 46.9; H, 1.7; N, 13.5%; IR νmax(KBr)/cm-1 3452, 2975, 2926, 2211; 1H NMR δH (CDCl3) 4.04 (2H, s, SCH2), 7.58 (1H, d, J=8.4 Hz, H-3), 7.86 (1H, dd, J=8.4 & 2.4 Hz, H-4), 8.42 (1H, d, J=2.4 Hz, H-6).
ACKNOWLEDGEMENTS
We thank UAD for the studentships (KRS & GW), and Synthetic Chemicals Ltd for some financial support.
References
1. D. H. Bremner, A. D. Dunn, and K. A. Wilson, Synthesis, 1992, 528. CrossRef
2. D. H. Bremner, A. D. Dunn, K. A. Wilson, K. R. Sturrock, and G. Wishart, Synthesis, 1997, 949. CrossRef
3. D. H. Bremner, A. D. Dunn, K. A. Wilson, K. R. Sturrock, and G. Wishart, Synthesis, 1998, 1095. CrossRef
4. S. Okuda and M. M. Robison, J. Am. Chem. Soc., 1959, 81, 740. CrossRef
5. R. Gompper, E. Kutter, and W. Toepfl, Liebigs Ann. Chem., 1962, 659, 90. CrossRef
6. R. Gompper and H. Schaefer, Chem. Ber., 1967, 100, 591. CrossRef
7. M. Augustin, R. Schmidt, and W. Rudorf, Z. Chem., 1977, 17, 289.
8. K. Clarke, W. R. Fox, and R. M. Scrowston, J. Chem. Soc., Perkin Trans. 1, 1980, 1029. CrossRef
9. M. Kolb, Synthesis, 1990, 171. CrossRef
10. R. M. Mohareb, S. M. Sherif, A. Hashabi, and N. I. Abdel-Sayed, Coll. Czech. Chem. Comm., 1995, 60, 1578. CrossRef
11. R. B Katz, M. B. Mitchell, and P. G. Sammes, Tetrahedron, 1989, 45, 1801. CrossRef
12. D. Thomae, E. Perspicace, D. Henryon, Z. Xu, S. Schneider, S. Hesse, G. Kirsch, and P. Seck, Tetrahedron, 2009, 65, 10453 (and references therein). CrossRef
13. A. D. Cross and R. A. Jones, ‘An Introduction to Practical Infrared Spectroscopy’, Butterworths, London, 1969, pp. 45-100.
14. H. Baron, F. G. P. Remfry, and J. F. Thorpe, J. Chem. Soc., 1904, 85, 1726.
15. W. Dieckmann, Ber., 1894, 27, 102.