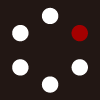
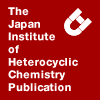
HETEROCYCLES
An International Journal for Reviews and Communications in Heterocyclic ChemistryWeb Edition ISSN: 1881-0942
Published online by The Japan Institute of Heterocyclic Chemistry
e-Journal
Full Text HTML
Received, 11th May, 2010, Accepted, 25th June, 2010, Published online, 28th June, 2010.
DOI: 10.3987/REV-10-675
■ Recent Advances in the Application of the Heck Reaction in the Synthesis of Heterocyclic Compounds
Majid M. Heravi* and Azadeh Fazeli
Department of Chemistry, School of Sciences, Azzahra University, Vanak, Tehran, Iran
Abstract
This brief review represents the synthesis of heterocyclic compounds via application of Heck reaction in recent years.CONTENTS
1 Introduction
2 Synthesis of aza heterocyclic compounds
2. 1 Cyclization via reactions of aryl halides
2. 2 Cyclization via reactions of vinyl halides
2. 3 Cyclization via functionalization of aromatic C-H bonds
3 Synthesis of oxygen-bearing heterocyclic compounds
3. 1 Cyclization via reactions of aryl halides
3. 2 Cyclization via reactions of vinyl halides
3. 3 Cyclization via functionalization of aromatic C-H bonds
4 Synthesis of heterocyclic compounds containing sulfur, sulfur and nitrogen, sulfur and oxygen
5 Conclusion
6 Acknowledgements
7 References and notes
1. INTRODUCTION
Heterocyclic compounds are worthy of attention for many reasons, chief among which are their biological activities, which many important drugs having one or more hetero atoms in their cyclic structures. Therefore, organic chemists have been making extensive efforts to produce heterocyclic compounds by developing new and efficient synthetic transformations. Over the past few decades, palladium-catalyzed coupling reactions have been extensively studied.1 The impact of the palladium-catalyzed cross-coupling reactions, discovered during the 1970s, has been considerable and continues to be the focus of much organometallic research.2 Palladium-catalyzed reactions for carbon-carbon bond formation, including Suzuki,3 Heck,4 Sonogashira,1 Tsuji–Trost5 as well as other reactions, have gained a predominant place in the arsenal of organic chemists. Palladium catalysis usually allows selective reactions with high turnover numbers and turnover frequencies under rather mild conditions. A number of palladium catalysts are commercially available and their reactivity, stability and selectivity can be tuned by ligands (phosphines, carbenes, amines, etc.) and/or additives.6
Palladium-catalyzed processes have proven to be a powerful and useful tool for the synthesis of heterocycles. Palladium has found such wide application, since it affects an extraordinary number of very different reactions, including many carbon-carbon bond-forming reactions, under relatively mild reaction conditions. Furthermore, palladium can usually be used in only catalytic amounts and tolerates a wide variety of functional groups, thus avoiding protection group complexity. Most palladium-based methodology proceeds stereo- and regioselectively in excellent yields.7 The wide utility of palladium in organic synthesis is vivid from the too many number of name reactions where the deep influences of this versatile transition metal enable it in the formation of C-C, C-O, C-N and even C-S bond under relatively mild conditions. The catalytic requirement and excellent tolerance of functional groups avoiding the protection-deprotection chemistry has made possible the use of palladium in the synthesis of small to large ring heterocyclic compounds.8 Catalytic amount of palladium is required for successful conversion, the catalyzed processes are in fact strongly dependent on other factors e.g. Base, ligand, temperature, additives and solvents.8 Palladium catalysis has achieved the status of an indispensable tool for both common and state-of-the art organic synthesis. Among basic types of palladium-catalyzed transformation such as, e.g., allylic substitution of cross coupling may seem to be more advanced, none can match Heck chemistry in resourceful versatility, the overwhelming ability to spawn new, hitherto unexpected applications, and resolving challenges.9 There are many advantages associated with Pd-mediated reactions, particularly ease of scale-up and tolerance to water and/or other functional groups, such as ketones, esters, amides, ethers, or heterocyclic rings, which supply poly functional molecules. The Heck reaction is one of the most important carbon-carbon bond-forming reactions and has been used in a variety of complex natural product syntheses. The interest in the Heck reaction has recently increased significantly. Perhaps the most dramatic progress to date is the development of an enantioselective variant.10 In the following sections we are trying to cover and update the recent application of Heck reaction in the synthesis of variety of heterocyclic compounds.10
2. SYNTHESIS OF AZA HETEROCYCLIC COMPOUNDS
2.1 CYCLIZATION VIA REACTIONS OF ARYL HALIDES
The cascade process utilized a Pd(OAc)2/ PPh3 precatalyst combination for the intramolecular Heck reaction which affords 2 in situ. The known instability of 2 dictated a search for mild reaction conditions. Using dichloromethane as solvent allowed the reaction to be carried out at room temperature. A subsequent Ag(I) catalyzed imine to azomethine and ylide to cycloaddition cascade led to spiro-oxindoles regiospecifically in good yield with a reaction time of 16–18 h for the total cascade (Scheme 1).11
The intramolecular Heck reaction of aromatic amines and ethers using palladium/imidazolium salts was described. The use of tetra-n-butylammonium halide salts facilitated the reactivity of aromatic chlorides. An unexpected and novel palladium mediated cyclization was also described leading to the formation of a tricyclic adduct (Scheme 2, 3, 4).12 Further studies on substrate 5 were frustrated by problems of isomer separation; however, Caddick and Kofie have tentatively assigned the product as a mixture of regioisomers 6, 7, 8 (yields, between 20 and 70%) (Scheme 2).12
However, Caddick and Kofie were able to isolate a single product 10 from reaction of substrates 9. They found that the iodide and bromide 9 proceeded to give the product 10 in good yields (I, 82%, 1 h; Br, 60%, 5 h) but the chloride 9 gave only 4 % of product 10. However, repetition of the reaction but using tetra-n-butylammonium bromide or iodide, had a dramatic effect, improving the yield of 10 from 4% to 28–32% (Scheme 3).12
Scheme 412 presents the results of studies on intramolecular Heck reactions using Pd2dba3 and imidazolium salt A.
Allylation of aldehyde 13 with allyl bromide and unactivated zinc in DMF for 30 min smoothly afforded in 98% yield homoallylic alcohol 14 as a yellow oil, which set the stage for 5-exo cyclization via an intramolecular Heck reaction. Treatment of 14 with Pd(OAc)2 (0.05 equiv), PPh3 (0.1 equiv) and TEA (2 equiv) in MeCN at 70 oC for 3.5 h led to the desired cyclopenta[c]pyridine intermediate 15 in 80% yield (Scheme 5).13
From N-methyl-2-iodoaniline and commercially available cyclopentane-1,3-dione, the condensation product 20 (X= I) was synthesized by heating overnight without any solvent. The key synthetic step was the Pd-catalyzed cyclization of 20 (X= I) to 21 in the presence of Pd(OAc)2 (5 mol%) and tri(o-tolyl)phosphine ((2-MeC6H4)3P) in DMF under microwave heating (100 oC, 5 min) to afford, after column chromatography and recrystallization, 99% of 21. However, further improvements could be made if it was possible to replace the 2-iodoaniline with the much cheaper 2-bromoaniline as the precursor of 20 (X= Br). Unfortunately, the latter turned out to be less reactive in the cyclization reaction [30% conversion to 21 after 30 min]. However, upon conventional heating at 120 oC overnight, 21 was isolated in 95% yield (Scheme 6).14
A novel one-pot-synthesis of highly substituted indol-2-ones using a combination of Ugi and Heck reaction (U-4CRHeck) is described. The synthesized indol-2-ones represent an interesting pharmacological scaffold with four potential points of diversity. Thus, this novel reaction-type is amenable to combinatorial high-throughput screening (Scheme 7).15
The one-pot synthesis of 2-aryl- and 2-vinylindoles 29 based on a palladium-catalyzed intramolecular
Heck reaction was reported. Intramolecular Heck reactions of the resulting 2-chloroanilino enamines 28 were achieved using an in situ generated palladium complex derived from an N-hetercyclic carbine (Scheme 8).16
Synthesis of five and six membered heterocycles, indulines, 2,3-dihydrobenzofurans (Scheme 10),17 chromans, 1,2,3,4-tetrahydroquinolines (Scheme 11),17 and 1,2,3,4- tetrahydroisoquinolines (Scheme 9),17 in 70-99% yield by a ligand-free palladium catalyzed reductive Heck cyclization of phenyl bromides
and chlorides, under mild conditions, was reported. Water was found to be essential for these reactions.
In the presence of 10% of Pd(OAc)2(PPh3)2 and 2 equiv of iPr2NEt in acetonitrile at 82 °C, 36 yielded to a mixture of two cyclized products (3/1 ratio) in a very good yield. Under the reductive conditions, two aldehydes in a 3/1 ratio were obtained in poor yield. The major one was identical with 39 and the minor one with 40. Therefore, the stereochemical outcome of the reductive and non reductive cyclizations was not influenced by the presence of the aminal even if a loss of the enantiomeric purity was observe (Scheme 12).18
The various kinds of N-acyldihydropyridones 41 were conveniently prepared from heteroaryl Grignald reagents and N-acylpyridinium salts. Subsequently, dihydropyridones 41 were converted to 42 by use of an intramolecular Heck cyclization (Scheme 13).19
Ugi-Smiles/Heck coupling/isomerization cascade can be done in a one-pot procedure by destroying the residual isocyanide before the Pd coupling (Scheme 14).20
The palladium-mediated transformation of 3,4-dihydro-2(1H)-pyridinones 49 featuring a (2-bromophenyl)ethyl substituent in the 5-position produced spirocyclic products, imides 51 and amides 52. The formation of these products can be explained by insertion of the enamide double bond into the initial aryl-Pd bond followed by oxidation or reduction of the organopalladium intermediate. Alternatively, formation of these spiro compounds might proceed via acyliminium ion intermediates (Scheme 15).21
A tandem sequence involving palladium-catalyzed sequential Heck-reduction-cyclization transformations in mild conditions has been developed for the synthesis of oxindoles. The protocol involved inexpensive reagents and did not require any additives such as bases or ligands (Scheme 16).22
The best result for cyclization was obtained when 58 was treated with 3 mol% of Pd(PPh3)4 and 1 equiv of Ag3PO4 in DMSO to give 59 (Scheme 17).23
Treatment of N-alkylbenzazepinone 60 with Pd(OAc)2 in DMF containing K2CO3 (2 equiv) and Bu4N+Br- (1 equiv) at 110 oC for 2 h provided the desired tetracyclic ring structure of dehydroisoindolinobenzazepinone 61 91% yield. Subsequent palladium-catalyzed hydrogenation of the dehydroisoindolinobenzazepinone 61 readily furnished the corresponding isoindolinobenzazepinone 62 in 83% yield (Scheme 18).24
Weinrich and Beck reported the palladium-catalyzed one-pot N-alkylation/Heck cyclization of anilines to substituted indoles employing Pd(OAc)2/XPhos (Scheme 19).25
A simple method for the preparation of 1,7-naphthyridine and 1,6-naphthyridine from the corresponding aminopyridine starting materials was presented. Crude 68 was treated with DMF and a catalytic amount of triethylammonium iodide in ethyl ether (generated by mixing TEA and HI) and heated to 70 °C for 16 h to cleanly provide 69 in 76% yield over the two steps. Formation of 73 under these conditions occurred via a multistep sequence involving Heck coupling, palladium-mediated oxidation of the allylic alcohol to the aldehyde, double bond isomerization, cyclization, and dehydration (Scheme 20).26
The use of condition a and condition b in the reaction mixture was found to be critical for the endo-type cyclization to provide 75 and 76. The yields were 75 (54%) and 76 (47%) (Scheme 21).27
Amides 77, when irradiated in a microwave vessel using standard Heck conditions [3 equiv TEA, 5 mol% Pd(OAc)2, 10 mol% P(o-tolyl)3, DMF], afforded the unexpected 6-exo-trig derivatives in 15 minutes. The structures of 78 were confirmed by NMR analyses (Scheme 22).28
3-Bromopyridine-4-carbaldehyde 79 was tethered with suitably electron withdrawing group substituted alkenes via Heck coupling followed by aldol reaction in dioxane at 150 oC under a catalytic system of Pd(OAc)2/PPh3/NaOAc to afford the corresponding isoquinolines in good yields (Scheme 23).29
It has been shown that 2-iodoaniline 82 undergoes coupling and cyclization with an array of α,β-unsaturated carbonyl compounds 83 in the presence of a palladium catalyst along with a base to afford quinolones 84. The present reaction is applicable to the synthesis of 2,3- and 2,4-disubstituted quinolines by the variation of the starting α,β-unsaturated ketones (Scheme 24).30
Tetrahydropyrido[2,3-c]coumarin derivatives were synthesized by intramolecular radical cyclization and heck coupling. This method allowed the synthesis of the backbone of the santiagonamine alkaloid (Scheme 25).31
87 was treated with a catalytic amount of palladium(II) acetate in the presence of (x-phos) and potassium acetate in bis(2-methoxyethyl)ether at 80 oC for 3 days, after which the desired Heck-cyclization product 88 was isolated in 33% yield together with 41% of the deallylated and debrominated naphthalene 89. In the second reaction, 87 was treated with a catalytic amount of tetrakis(triphenylphosphine) palladium(0) and 4 equiv of sodium acetate in boiling ethanol. After a reaction time of 4 days the desired Heck-reaction product 88 was isolated in 36% yield. Although these two reactions give similar results, the latter reaction conditions were selected to perform the Heck-cyclization of naphthalene 87 since the desired Heck-cyclization product was formed predominantly under these conditions and could easily be purified by column chromatography (Scheme 26).32
The palladium-mediated intramolecular cyclization of 90 was believed to give rise to the formation of 4-methylene intermediate 91, which is formed as the Heck cyclization product and is likely to isomerize to the more conjugated and thus, more stable isomer 92. Indeed, after treatment of the N-protected 1,4-naphthoquinone 90 with palladium(II) acetate and an excess of sodium carbonate in boiling acetonitrile for 3 h, none of the 4-methylene intermediate 91 was detected in the reaction mixture. Instead, 93 was obtained as the major reaction product and it was isolated in 45% yield together with 13% of the desired 92 (Scheme 27).32
Reaction of 95 with Pd(PPh3)4 under the conditions used for cyclisation of the tertiary amides (TEA, EtCN, 100 oC) gave the two cyclized products 97 (4-methyl-5-nitroisoquinolin-1-one) and 98 (4-methylene-5-nitro-3,4-dihydroisoquinolin-1-one) in a 1:1 ratio, along with the dehalogenated amide 99 (Scheme 28).33
Conducted with substrate 100 by applying the concept of Jeffery’s two-phase protocol in the presence of Pd(OAc)2, KOAc and tetrabutylammonium bromide (TBAB) in dry DMF under a nitrogen atmosphere for 6 h. The eight-membered exo-Heck product 101 was obtained in 72% yield without any contamination of the endo-Heck product 102 (Scheme 29).34
Substrate 103 was allowed to react with phosphine-free Jeffery’s two-phase protocol, that is, Pd(OAc)2/KOAc/TBAB/DMF/90 oC/N2 conditions and pleasingly the cyclized product 104 was obtained in 59% yield. When the reaction was performed with the same substrate 103 using Pd(OAc)2 as catalyst, NaOAc as base, and Ph3P as ligand in dry DMA at 90 oC for 3.5 h the corresponding exo-Heck product 105 was obtained as the sole product in 82% yield (without TBAB as phase transfer catalyst) (Scheme 30).35
The cyclization reaction to give compounds 107 was performed with a catalyst system containing 10 mol% of palladium(II)acetate, 1.0 equiv of tetrabutylammonium chloride (TBAC) and 2.5 equiv of sodium hydrogen carbonate in dimethylacetamide at 90 oC (Scheme 31).36
In the case of compounds 108, better results were obtained using 10 mol% of palladium(II)-acetate, 20 mol% of triphenylphosphine, 3.0 equiv of potassium acetate in acetonitrile at reflux. In these conditions, besides the γ-carbolinones 109, the isomeric γ-carbolinones with exocyclic double bond, 110 were also obtained (Scheme 32).36
A synthetic route to pharmaceutically important tricyclic pyrrolopyrimidines was developed. The method employed a palladium-mediated Heck cyclization as the critical step in the construction of the final six membered rings (Scheme 33).37
In the presence of a catalytic (5%) amount of Pd(OAc)2, AcOK in DMF (90 °C, 2 h) the dihydropyridine 113 was regioselectively converted into 114. Therefore, 114 resulted from an anti carbopalladation then a syn β-elimination and an isomerisation of the double bond. Acidic hydrolysis of the aminal group in 114 afforded the aldehyde 115. A tandem cyclization–hydride capture performed on 113, in the presence of 5% of Pd(OAc)2(PPh3)2 and piperidinium formate, gave the two diastereomeric products 117 (94% de) and 119 (95% de) in a 7:3 ratio. Aminals 117 and 119 were respectively converted into the aldehydes 118 and 120 by acidic hydrolysis (Scheme 34).38
Kiely and Guiry described cyclisations of 121 using Pd complexes generated from (R)-BINAP 124 and the phosphinamine ligands 125–128 (Scheme 35).39
Using Pd(OAc)2/PCy3 (tricyclohexylphosphine) under standard conditions, the intramolecular Heck cyclization of 129 was found to proceed to completion rapidly. In related intramolecular Heck cyclization reactions of benzamides, a range of double bond isomers have been reported. In this study, none of the bridgehead double bond isomer was formed, and 131 was observed as the major product, along with trace amounts of 132 and 133 double bond isomers. Investigation of a range of bases at the optimum reaction temperature (140 oC) showed that DIPEA gave comparable results to those obtained in the presence of MeNCy2 (dicyclohexylmethylamine), however, TEA, K2CO3 and 2,6-lutidine gave lower conversion levels (Scheme 36).40
A novel approach towards the construction of the galanthamine skeleton was demonstrated by the Pd- catalyzed cyclization of N-[2-(1,4-dioxa-spiro[4,5]dec-7-en-8-yl)ethyl] 2-iodo-4-methoxy-N-methyl benzamide, with formation of the benzazepine ring and creation of a quaternary carbon (Scheme 37).41
Compound 136 was subjected to Heck reaction condition using Pd(OAc)2, Ph3P, and K2CO3 in MeCN, the desired intramolecular cyclization was achieved to provide dispiro-compound 137. The ethylene ketal group of 137 was removed easily when put in contact with silical gel in methanol to give ketone 138 in 95% yield. Subsequent removal of the benzyl group in 138 with SnCl4 was accompanied by a spontaneous intramolecular Michael addition to afford tetracyclic oxolycoraminone 139 in 75% yield. Simultaneous reduction of both the ketone and amide groups of 139 with LAH afforded (+)-140 with excellent diastereoselectivity (de >95%) (Scheme 38).42
The Heck reaction was carried out in N,N-dimethylacetamide using MeNCy2 (dicyclohexylmethylamine) as a base, Et4NCl as promoter, and Pd(OAc)2 as precatalyst. All reactions gave the 8-endo products 142 exclusively in up to 72% yield. Bromo analogues required longer reaction times (12 h) in contrast to the corresponding iodo compounds (4 h) (Scheme 39).43
The Heck reaction was carried out first, giving a selective reaction with the aryl iodide in the presence of an aryl bromide 144. Very good chemoselectivity was observed, giving products 145 exclusively in 82% yield. The carbamate 145 (X= NC(O)OC(CH3)3) was deprotected using TFA to give the free aniline 145 (X= NH). Compound 145 (X= NH) gave the dibenzo[b,f]azepine 146 in 74% yield. Phenol 145 (X= OH) was obtained in 82% yield using 2-iodophenol and 144. The cyclization of 145 (X= OH) using the catalytic conditions developed by Buchwald and coworkers gave 146 (X= O) in 60% yield (Scheme 40).43
Heck cyclization on the Ugi product 149 proceeded smoothly to produce the seven membered lactam 150 in excellent yield (Scheme 41).44
Substrate 151 cleanly underwent microwave-assisted Heck cyclization to generate 152 in 90% yield (Scheme 42).44
The resin-bound Ugi adduct 153 was washed with DMF, MeOH, and CH2Cl2, and then subjected to two different solid-phase Heck cyclization conditions. The first set of reaction conditions utilized Bu4NCl, KOAc, Ph3P, and Pd(OAc)2 in DMF while the second example mimicked their solution phase conditions described above (149, 151) with the sole difference being the addition of Bu4NCl. Following the Heck reactions, the resin was washed with DMF, DMF/water 1:1, DMF, MeOH, and CH2Cl2 . The cyclized adducts were cleaved from the resin (1:1 TFA/CH2Cl2) and the resulting acids were methylated utilizing (trimethylsilyl)diazomethane in MeOH, to produce ester 154 with moderate yields (33–44%) (Scheme 43).44
Aldehydes containing a vinylogous bromide were condensed with 4-butenamine and then treated with phenylTosMIC and potassium carbonate to give the corresponding imidazoles. The imidazoles were then subjected to the Heck reaction to give the desired imidazo[1,5-a]azepines (Scheme 44).45
Compounds 158, obtained by alkylation of 157 with 2-bromobenzyl bromide, were subjected to different Heck reaction conditions using Pd(OAc)2 as catalyst in the presence of a base in DMF. 158 were fully converted to the cyclized benzazepines 159 using either K2CO3 or Oct3N as a base. The reaction was very selective since only 159 were obtained among the different products, which could be formed during the reaction. Since the reaction products 159 were isolated by precipitation in Et2O and filtration, the use of lipophilic Oct3N was preferred because it was readily eliminated during this operation (Scheme 45).46
2. 2 CYCLIZATION VIA REACTIONS OF VINYL HALIDES
The Heck reaction of (E)-160 occurred under somewhat harsher conditions: Pd(PPh3)4 (20 mol%), Ag2CO3 (2 equiv) in acetonitrile at 50 °C for 5 h, furnishing after chromatographic purification the diene (Z)-161 in 44% yield (74% based on converted product) along with 40% of starting (E)-160 (Scheme 46).47
The intramolecular heck cyclization of N-allyl-, -aryl- or -benzyl-5-allyl-2-pyrrolidinones and N-allyl-, -aryl-, or -benzyl-6-allyl-2-piperidinones, prepared through allyltrimethylsilan addition to the corresponding cyclic N-acyliminium ions, afforded indolizidinones, quinolizinones and benzoazepinones in moderate to good yields (56-90%) (Scheme 47).48
An efficient approach to the bridged framework of the indole alkaloid ervitsine , featuring a ring-closing metathesis reaction from a 2,3-disubstituted indole followed by a vinyl halide Heck cyclization upon the resulting cycloheptane ring, was described (Scheme 48).49
The precursors 176 on reaction with Pd(OAc)2 (10 mol%), PPh3 (0.25 equiv) and Cs2CO3 (1.2 equiv) in DMF at 90–100 oC yielded the fused tetrahydropyridine derivatives 177 in good to excellent yields via 6-exo-trig cyclization (Scheme 49).50
But when N-methallylated derivatives 178 were subjected to the Heck reaction under the same reaction conditions (Scheme 49)50 they gave cyclopropa[d]fused isoquinoline derivatives 179 (Scheme 50).50
Asymmetric intramolecular Heck cyclization of enamide 180 using Ag3PO4 as a halide scavenger in combination with Pd·(R)-BINAP complex in DMF at room temperature provided 181 in 85% enantiomeric excess, while reactions conducted in tetrahydrofuran gave dieneamide 182 as the major product (Scheme 51).51
2. 3 CYCLIZATION VIA FUNCTIONALIZATION OF AROMATIC C-H BONDS
The Heck reaction was effective in the presence of a Pd(OAc)2/PPh3 catalytic system and silver carbonate as base. The reaction was performed in excellent yield with 0.1 equiv of palladium catalyst in 2 h (Scheme 52).52
Protected indole 185 was submitted to the Heck coupling reaction and afforded the desired product 186 in an excellent yield. The cyclization occurred with only 0.05 equiv of Pd(OAc)2 in 1 h (Scheme 53).52
The cyclization of 187 (0.05 equiv of Pd(OAc)2) afforded 188 in 59% yield and was then improved up to 70% when 0.1 equiv of Pd(OAc)2 was used (Scheme 54).52
N-electron withdrawing protecting groups such as Boc or SO2Ph prevented cyclization. However, N-Me and N-EOM compounds 189 (R1= Me, R2= Me) and 189 (R1= EOM, R2= EOM) allowed the intramolecular cyclization with excellent yield (89-92%) (Scheme 55).52
Beccalli and coworkers reported the synthesis of tricyclic fused quinolone and naphthyridone derivatives, by an intramolecular Heck cyclization. They also reported the use of microwave irradiation to obtain, in some cases, better yields of cyclized products (Scheme 56, 57).53
The short total synthesis of paullone 198 and dimethyl paullone 199 via a novel palladium-catalyzed intramolecular coupling using the o-bromo- and o-iodo anilides of indoles (195 and 196) and N-methyl indole 197 was described (Scheme 58).54
The intramolecular ring closure of 202 was performed in the presence of Pd(OAc)2 (0.05 equiv), PPh3 (0.1 equiv), and silver carbonate (2 equiv) in DMF at 100 oC for 1 h to give 203 in excellent yield Sequential deprotection of 203 was achieved by removal of the N-Boc group (1N NaOH and 1,4-dioxane) and then the N-EOM group (1N HCl and 1,4-dioxane) to afford, respectively, 204 and 205 in good yield (Scheme 59).55
Heck cyclization procedure was applied on 207 to afford 208 in 82% yield. Removal of the Boc group of 208 was effective by treatment with a solution of trifluoroacetic acid in dichloromethane at room temperature to give 209 in 88% yield (Scheme 60).55
The ring closure of 210 and 211 were carried out when 0.1 equiv of Pd(OAc)2, PPh3 and Ag2CO3 in dimethylformamide were used and the cyclization was performed at 140 oC (Scheme 61).55
The cyclization reaction of 215 was performed in the presence of 0.1 equiv of palladium catalyst at 100 oC for 2 h to afford 216 in 92–96% yield. A two-step deprotection sequence was developed to reach compounds 217. Removal of the N-Boc group was first carried out by treatment of 216 with trifluoroacetic acid in dichloromethane at room temperature followed by the second deprotection in the presence of TBAF in refluxing THF. The derivatives 217 were obtained in 40–43% yield (two-step yield) (Scheme 62).55
Lee and coworkers synthesized novel tetracyclic fused indole derivatives via the intramolecular Heck reaction of indole-containing Baylis–Hillman adducts in good to moderate yields (Scheme 63).56
A new synthetic protocol has been developed for the arylation of secondary and N-alkylated amide Heck precursors by the implementation of the palladium-catalyzed intramolecular Heck reaction strategies (Scheme 64).57
Nitrogenated heteropolycyclic systems were obtained by intramolecular palladium-catalyzed coupling reactions promoted by microwave irradiation (Scheme 65).58
The intramolecular process, obtained using Pd(OAc)2 as precatalyst, AcOK as base, TBAC as additive and DMA as solvent, afforded two regioisomers 228 and 229 arising from the cyclization on position 2 or 4 of the pyridyl ring, in 1:3 ratio in favour of the para position. Similarly tricyclic systems 231 was obtained when 230 were treated with Pd(OAc)2, AcOH, TBAC and DMA at 100 oC (Scheme 66).59
The tertiary amide 232 was then treated according to the reported conditions, to give the cyclized product 233 in good yield (Scheme 67).59
3. SYNTHESIS OF OXYGEN- BEARING HETEROCYCLIC COMPOUNDS
3. 1 CYCLIZATION VIA REATIONS OF ARYL HALIDES
The ester 234 was subjected to Heck reaction under standard conditions (10 mol% Pd(OAc)2, 20 mol% (o-tol)3P and n-Bu3N in DMF). The cyclization reaction was smoothly preceded in a 5-exo-trig manner to give rise to a spiro-lactone 235 in 95% yields. Finally, in the next stages, 1,13-dihydroxyherbertene 236 was obtained in 100% yield (Scheme 68).60
The catalytic combination of Pd2(dba)3/HP(t-Bu)3.BF4 and DABCO gave an unusual intramolecular Heck reaction with dihydronaphthalene substrates, yielding formal anti-hydride elimination products in good to excellent yields under mild conditions. For dibromo substrates, multiple Heck reactions was possible when an external acceptor was added to afford more highly functionalized products (Scheme 69).61
When ortho-iodobenzyl allyl ether was treated with 5 mol% PdCl2, 1.5 equiv (n-Bu)3N and 1 equiv HCO2NH4 at 60 oC for 24 h, it disappeared completely and the product 241 was obtained in 71% isolated yields (Scheme 70).62
An enzymatically generated diene diol was utilized as homochiral starting material in the total synthesis of (+)-codeine featuring a Mitsunobu inversion and two intramolecular Heck cyclizations. The first Heck cyclization was mediated by Pd(OAc)2 and provided aldehyde 243 in 82% yield, which was converted to vinyl bromide 244 by a Wittig reaction. The second Heck cyclization, performed according to Trost’s conditions, gave a low yield (44%) of the complete phenanthrene skeleton in 245 (Scheme 71).63
Enzymatic dihydroxylation of β-bromoethylbenzene provided a homochiral diene diol that served as starting material for the synthesis of the complete morphinan skeleton via an intramolecular Heck cyclization. Protection of the C-6 hydroxyl as the silyl ether 247 (R= TBS) provided the key intermediate for the Heck reaction leading to the pentacyclic carbamate 248 in 74% yield (Scheme 72).64
These olefins 250 cyclized to the corresponding 5-desoxipterocarpens 251 (R=H, OMe) in the presence of 5 mol% of Pd(OAc)2 and Pterocarpen 253 was obtained from 252 through an intramolecular Heck reaction in the presence of 5 mol% of Pd(OAc)2. Finally, coumestan 254 was prepared in quantitative yield by oxidation of 253 with DDQ in THF (Scheme 73).65
Synthesis of 257 and 259, in 74% yield by a ligand-free palladium catalyzed reductive Heck cyclization of phenyl bromides, under mild conditions, was reported. Water was found to be essential for this reaction (Scheme 74).17
Intramolecular Heck reaction of 260, followed by hydrogenation, smoothly proceeded to afford the lactone 261 in 71% yields. Finally, in the next stages, 1,15-dihydroxyherbetene 262 was obtained in 83% yield (Scheme 75).60
The modified reagent system [Pd(OCOCF3)2/P(2-furyl)3/EtNiPr2] gave enhanced conversions compared with standard conditions [Pd(OAc)2/PPh3/NEt3] for intramolecular Heck cyclizations, and when used in supercritical carbon dioxide (scCO2) results in the suppression of double bond isomerisation to minimal levels, which is otherwise a serious competing side reaction in conventional solvents (Scheme 76).66
An in situ formed catalyst of Pd(OAc)2 and ligand 271 (10 mol%, Pd : L ratio = 1 : 2) was utilized and the reaction was performed in CHCl3, using 3 equiv of Cy2MeN as a base. The reaction mixture was heated to 80 oC for 48 h under an inert atmosphere (Scheme 77).67
Compound 272 was reacted under standard Heck condition to give a mixture of three isomeric 5-nitro-1-benzopyrans (273-274-275) in an 18:4:1 ratio. Reductive N-heteroannulation of 273 using 10 mol% palladium diacetate (Pd(OAc)2), 20 mol% 1,3-bis(diphenylphosphino)propane (dppp) in DMF at 120 oC and 4 atm of carbon monoxide gave an acceptable 63% yield of the fused indole 276 after 70 h (Scheme 78).68
Allyl 2-iodobenzyl ether 266, allyl 2-iodobenzoate 277, cyclohexenyl 2-iodobenzyl ether 280, and cyclohexenyl 2-iodobenzoate 283 were subjected to Heck conditions [Pd(OAc)2 (10 mol%), PPh3 (20 mol%), TEA (2 equiv), AgNO3 (1 equiv), MeCN, 80 oC], and the products were isolated by flash chromatography. For ether-tethered aryl iodides 266 and 280, cyclization gave high yields of products 267+269 and 281+282. Ester-tethered aryl iodides 277 and 283 gave only unreacted starting material and deiodinated, uncyclized products 279 and 286. Ester-tethered cyclization products 278 and 284+285 were produced in trace amounts. Oxidation of cyclic ethers 281+282 with PCC provided cyclic esters 284+285 in good yield (59%) (Scheme 79).69
Heck reaction with iodide 287 (X=I), bromide 287 (X=Br) and triflate 287 (X=OTf) under various conditions led to the formation of 288 with different results. The usual ligands for asymmetric Heck reactions, BINAP or the oxazoline C, led to very low enantioselection or no conversion at all. The conversion rates could be increased by the addition of silver carbonate, but this had almost no positive effect on the enantiomeric excess. Other ligands ((R)-PHANEPHOS, (S, S)ET-BPE, ligand E) were either inactive or produced tricycles with low stereoinduction. The use of the JOSIPHOS ligand D, employed primarily in hydrogenation reactions, gave a remarkably high enantiomeric excess. Recrystallisation of this product gave a virtually enantiopure material. Additionally, the use of bromide 287 (X=Br) gave almost the same enantiomeric excess but with lesser yield than the use of 287 (X=I). However, in this case, the conversion of starting material was complete. The reaction gave rise to the hydrogenated product 287 (X=H), which in the case of the iodide was only observed with an addition of silver carbonate. The change from a bromide to a triflate or non-aflate leaving group had almost no effect on the stereoinduction of the product, although the yields were relatively lower (Scheme 80).70
When the intramolecular Heck reaction was carried out with 289 in the presence of 10 mol% of Pd(OAc)2 as a catalyst and KOAc (2.75 equiv) as a base and tetrabutylammonium bromide as a promoter in DMF at 120 oC for 4–6 h, the 8-exo cyclized product 290 was obtained as the major product in 78 % yield, along with the 9-endo cyclized 291 as a minor product in 20% yield (Scheme 81).71
When the intramolecular Heck reaction was performed with precursor 292 in the presence of Pd(OAc)2 as a catalyst, KOAc as a base, and tetrabutylammonium bromide (TBAB) as an additive in dry DMF as solvent for 2 h under a nitrogen atmosphere, the eight-membered naphthoxocine compound 293 was obtained in excellent yield (86%) (Scheme 82).72
Syntheses of nine-membered oxa-heterocyclic compounds by the application of the intramolecular Heck reaction have been difficult to develop. Herein, Majumdarand and Chattopadhyay, described the synthesis of this class of compounds through the 9-endo-trig cyclization and 8-endo-trig cyclization, respectively a rare mode of cyclization in the literature (Scheme 83).73
3. 2 CYCLIZATION VIA REACTIONS OF VINYL HALIDES
The vinyl iodide 299 was prepared starting from propargyl bromide and trans-cinnamyl alcohol in three steps and was subjected to intramolecular Heck reactions to give the product as a mixture of two isomers in a ratio of 9:1, with the (Z,E)-product 300 predominating (Scheme 84).74
The intramolecular Heck reaction was performed with O-allylated 301 in the presence of Pd(OAc)2, PPh3, Cs2CO3 and TBAC (tetrabutylammonium chloride) in DMF at 80-85 oC to afford pyran derivatives 302 (Scheme 85).75
O-Methallylated compounds 303 and aromatized compounds were subjected to intramolecular Heck reaction under the same conditions (Pd(OAc)2, PPh3, Cs2CO3 and TBAC (tetrabutylammonium chloride) in DMF at 80-85 oC) to afford pyrans 304 (Scheme 86).75
An efficient and convenient method for the synthesis of 2-aryl substituted tetrahydropyran, tetrahydrofuran, and oxepine derivatives via palladium catalyzed intramolecular Heck reaction was developed (Scheme 87).76
3. 3 CYCLIZATION VIA FUNCTIONALIZATION OF AROMATIC C-H BONDS
It was found that a 1:2 ratio of Pd:ethyl nicotinate was ideal and that inclusion of a substoichiometric amount of NaOAc (20 mol%) provided increased yields. Finally, increasing the temperature to 100 °C led to optimal results, providing benzofuran 310 in 77% yield after 12 h (Scheme 88).77
Under standard reaction conditions (Pd(OAc)2 10 mol%, ethyl nicotinate 20 mol%, benzoquinone 1 equiv, NaOAc 20 mol%, t-AmOH: AcOH 4: 1, 100 oC), ether 311 cyclized to produce a diastereomerically pure product in 60% yield, which was determined to be dihydrobenzofuran 313 by 1H NMR NOE experiments (Scheme 89).77
2-bromophenoxy pyridines were treated with Pd(OAc)2 to afford functionalized benzo[4,5]furo[3,2-c]pyridine 315. Under optimized conditions, both electron-deficient and electron-donating substrates gave good yields of the desired products (Scheme 90).78
Precursor 316, when subjected to intramolecular Heck reaction in the presence of 10 mol% of Pd(OAc)2 as catalyst KOAc as base and tetrabutylammonium bromide as promoter in DMF at about 100 oC, afforded linearly fused bis-cyclized product 317 (Scheme 91).79
4. SYNTHESIS OF HETEROCYCLIC COMPOUNDS CONTAININ SULFUR, SULFUR AND NITROGEN, SULFUR AND OXYGEN
Both the iodo and bromo derivatives 318 afforded cyclic products in good yield when exposed to the Heck reaction conditions (67% and 69%), giving a novel cyclic sulfone 319 (Scheme 92).43
For Heck cyclizations of the α-bromovinylsulfonamides Merten and his coworkers chose two established catalytic systems. Besides standard conditions A, they also applied conditions B, which have recently been utilized for the synthesis of lactams via a tandem Heck-allylic substitution reaction. However, next to formation of the expected α-methylene-γ-sultams, double bond migration by readdition of the palladium hydride species and occasionally also a complementary regioselectivity of carbopalladation (6-endo instead of 5-exo) was noticed under both conditions. Since these undesired features were especially pronounced for substrate 320, Merten and his cowokers investigated the effect of silver and thallium additives on the Heck cyclization of this bromovinylsulfonamide (Scheme 93).80
Substrate 327 was subjected to standard Heck conditions in the hope that a regioselective cyclisation would occur. In the event, using catalytic amounts of either Pd(OAc)2 or Pd(dba)2, starting material 327 was completely consumed (Scheme 94).81
Syntheses of hitherto unreported heterocycles, such as oxathiocine derivatives, in excellent yields, and a doubly cyclized oxathiocine derivative, through a intramolecular Heck reaction via an unusual 8-endo-trig cyclization, were reported (Scheme 95).82
5. CONCLUSION
In this review, we have presented numerous very useful processes for the synthesis of heterocycles, via intramolecular Heck cyclization, reported in recent years. The reactions proceed under relatively mild reaction conditions and tolerate a wide variety of functional groups. Most palladium-based methodologies proceed stereo- and regioselectively in excellent yields.
6. ACKNOWLEDGEMENTS
The authors are thankful to Alzahra university Research Council for partial financial support.
References
1. M. M. Heravi and S. Sadjadi, Tetrahedron, 2009, 65, 7761. CrossRef
2. N. Marion and S. P. Nolan, Acc. Chem. Res., 2008, 41, 1440. CrossRef
3. F.-X. Felpin, T. Ayad, and S. Mitra, Eur. J. Org. Chem., 2006, 2679. CrossRef
4. J. P. Knowles and A. Whiting, Org. Biomol. Chem., 2007, 5, 31. CrossRef
5. D. Astruc, Inorg. Chem., 2007, 46, 1884. CrossRef
6. M. Lamblin, L. Nassar- Hardy, J. C. Hierso, E. Fouquet, and T. T. Felpin, Adv. Synth. Catal., 2010, 352, 33. CrossRef
7. G. Zeni and R. C. Larock, Chem. Rev., 2006, 106, 4644. CrossRef
8. K. C. Majumdar, B. Chattopadhyay, P. K. Maji, S. K. Chattopadhyay, and S. Samanta, Heterocycles, 2010, 81, 795. CrossRef
9. I. P. Beletskaya and A. V. Cheprakov, Chem. Rev., 2000, 100, 3009. CrossRef
10. M. Shibasaki, E. M. Vogl, and T. Ohshima, Adv. Synth. Catal., 2004, 346, 1533. CrossRef
11. R. Grigg, E. L. Millington, and M. Thornton-Pett, Tetrahedron Lett., 2002, 43, 2605. CrossRef
12. S. Caddick and W. Kofie, Tetrahedron Lett., 2002, 43, 9347. CrossRef
13. J. Zhao, X. Yang, X. Jia, S. Luo, and H. Zhai, Tetrahedron, 2003, 59, 9379. CrossRef
14. U. S. Serensen and E. Pombo-Villar, Helv. Chim. Acta, 2004, 87, 82. CrossRef
15. M. Umkehrer, C. Kalinski, J. Kolb, and C. Burdack, Tetrahedron Lett., 2006, 47, 2391. CrossRef
16. L. Ackermann and A. Althammer, Synlett, 2006, 18, 3125. CrossRef
17. P. Liu, L. Huang, Y. Lu, M. Dilmeghani, J. Baum, T. Xiang, J. Adams, A. Tasker, R. Larsen, and M. M. Faul, Tetrahedron Lett., 2007, 48, 2307. CrossRef
18. P. Mangeney and C. Pays, Tetrahedron Lett., 2003, 44, 5719. CrossRef
19. D. L. Comins and K. Higuchi, Beilstein J. Org. Chem., 2007, 3, 42. CrossRef
20. L. E. Kaim, M. Gizzi, and L. Grimaud, Org. Lett., 2008, 10, 3417. CrossRef
21. G. Satyanarayana and M. E. Maier, J. Org. Chem., 2008, 73, 5410. CrossRef
22. F. X. Felpin, O. Ibarguren, L. Nassar-Hardy, and E. Fouquet, J. Org. Chem., 2009, 74, 1349. CrossRef
23. J. Maruyama, H. Yamashita, T. Watanabe, S. Aria, and A. Nishida, Tetrahedron, 2009, 65, 1327. CrossRef
24. W. Phakhodee, P. Ploypradith, P. Sahakitpichan, and S. Ruchirawat, Tetrahedron, 2009, 65, 351. CrossRef
25. M. L. Weinrich and H. P. Beck, Tetrahedron Lett., 2009, 50, 6968. CrossRef
26. P. W. Phuan and M. C. Kozlowski, Tetrehedron Lett., 2001, 42, 3963. CrossRef
27. G. Kim, J. H. Kim, W. J. Kim, and Y. A. Kim, Tetrahedron Lett., 2003, 44, 8207. CrossRef
28. T. L. Smalley and W. Y. Mills, J. Heterocycl. Chem., 2005, 42, 327. CrossRef
29. C. S. Cho and D. B. Patel, J. Mol. Catal. A: Chem., 2006, 260, 105. CrossRef
30. C. S. Cho and J. U. Kim, Tetrahedron Lett., 2007, 48, 3775. CrossRef
31. K. C. Majumdar, B. Chattopadhyay, and A. Taher, Synthesis, 2007, 3647. CrossRef
32. J. Jacobs, B. M. Mbala, B. Kesteleyn, G. Diels, and N. De Kimpe, Tetrahedron, 2008, 64, 6364. CrossRef
33. A. Dhami, M. F. Mahon, M. D. Lloyd, and M. D. Threadgill, Tetrahedron, 2009, 65, 4751. CrossRef
34. K. C. Majumdar, B. Chattopadhyay, and S. Samanta, Tetrahedron Lett., 2009, 50, 3178. CrossRef
35. K. C. Majumdar, S. Samanta, and B. Chattopadhyay, Tetrahedron Lett., 2009, 50, 4866. CrossRef
36. E. M. Beccalli, G. Broggini, A. Marchesini, and E. Rossi, Tetrahedron, 2002, 58, 6673. CrossRef
37. B. Dyck and J. R. McCarthy, Heterocycles, 2004, 62, 191. CrossRef
38. C. Pays and P. Mangeney, Tetrahedron Lett., 2001, 42, 589. CrossRef
39. D. Kiely and P. J. Guiry, Tetrahedron Lett., 2002, 43, 9545. CrossRef
40. L. R. Donaldson, D. Haigh, and A. N. Hulme, Tetrahedron, 2008, 64, 4468. CrossRef
41. P. H. Liang, L. W. Hsin, S. L. Pong, C. H. Hsu, and C. Y. Cheng, J. Chin. Chem. Soc. (Taipei, Taiwan), 2003, 50, 449.
42. P. H. Liang, J. P. Liu, L. W. Hsin, and C. Y. Cheng, Tetrahedron, 2004, 60, 11655. CrossRef
43. L. A. Arnold, W. Luo, and R. K. Guy, Org. Lett., 2004, 6, 3005. CrossRef
44. V. Gracias, J. D. Moor, and S. W. Djuric, Tetrahedron Lett., 2004, 45, 417. CrossRef
45. X. Beebe, V. Gracias, and S. W. Djuric, Tetrahedron Lett., 2006, 47, 3225. CrossRef
46. P. Ribiere, V. Declerck, Y. Nedellec, N. Yadav- Bhatnagar, J. Martinez, and F. Lamaty, Tetrahedron, 2006, 62, 10456. CrossRef
47. M. M. Segorbe, J. Adrio, and J. C. Carretero, Tetrahedron Lett., 2000, 41, 1983. CrossRef
48. L. S. Santos and R. A. Pilli, Synthesis, 2002, 87. CrossRef
49. M. L. Bennasar, E. Zulaica, D. Sole, and S. Alonso, Synlett, 2008, 5, 667. CrossRef
50. S. Nandi and J. K. Ray, Tetrahedron Lett., 2009, 50, 6993. CrossRef
51. K. Kiewel, M. Tallant, and G. A. Sulikowski, Tetrahedron Lett., 2001, 42, 6621. CrossRef
52. L. Joucla, A. Putey, and B. Joseph, Tetrahedron Lett., 2005, 46, 8177. CrossRef
53. E. M. Beccalli, G. Broggini, M. Martinelli, G. Paladino, and C. Zoni, Eur. J. Org. Chem., 2005, 2091. CrossRef
54. J. G. Avila-Zarraga, A. Lujan-Montelongo, A. Covarrubias-Zuniga, and M. Romer-Ortega, Tetrahedron Lett., 2006, 47, 7987. CrossRef
55. A. Putey, L. Joucla, L. Picot, T. Besson, and B. Joseph, Tetrahedron, 2007, 63, 867. CrossRef
56. H. S. Lee, S. H. Kim, T. H. Kim, and J. N. Kim, Tetrahedron Lett., 2008, 49, 1773. CrossRef
57. K. C. Majumdar, B. Chattopadhyay, and S. Nath, Tetrahedron Lett., 2008, 49, 1609. CrossRef
58. E. M. Beccalli, G. Broggini, M. Martinelli, and S. Sottocornola, Synthesis, 2008, 136. CrossRef
59. L. Basolo, E. M. Beccalli, E. Borsini, and G. Broggini, Tetrahedron, 2009, 65, 3486. CrossRef
60. Y. Fukuyama, H. Yuasa, Y. Tonoi, K. Harada, M. Wada, Y. Asakawa, and T. Hashimoto, Tetrahedron, 2001, 57, 9299. CrossRef
61. M. Lautens and Y. Q. Fang, Org. Lett., 2003, 5, 3672. CrossRef
62. X. Xie, B. Chen, J. Lu, J. Han, X. She, and X. Pan, Tetrahedron Lett., 2004, 45, 6235. CrossRef
63. A. T. Omori, K. J. Finn, H. Leisch, R. J. Carroll, and T. Hudlicky, Synlett, 2007, 2859. CrossRef
64. J. Zezula, K. C. Rice, and T. Hudlicky, Synlett, 2007, 2863. CrossRef
65. D. P. Sant’Ana, V. D. Pinho, M. C. L. S. Maior, and P. R. R. Costa, Tetrahedron Lett., 2009, 50, 3753. CrossRef
66. N. Shezad, A. A. Clifford, and C. M. Rayner, Tetrahedron Lett., 2001, 42, 323. CrossRef
67. R. Imbos, A. J. Minnaard, and B. L. Feringa, Dalton Trans., 2003, 2017. CrossRef
68. B. C. G. Soderberg, J. W. Hubbard, S. R. Rector, and S. N. O’Neil, Tetrahedron, 2005, 61, 3637. CrossRef
69. S. R.Woodcock and B. P. Branchaud, Tetrahedron Lett., 2005, 46, 7213. CrossRef
70. M. E. P. Lormann, M. Nieger, and S. Brase, J. Organomet. Chem., 2006, 691, 2159. CrossRef
71. K. C. Majumdar, B. Chattopadhyay, and K. Ray, Tetrahedron Lett., 2007, 48, 7633. CrossRef
72. K. C. Majumdar, B. Chattopadhyay, and B. Sinha, Tetrahedron Lett., 2008, 49, 1319. CrossRef
73. K. C. Majumdar and B. Chattopadhyay, Synlett, 2008, 979. CrossRef
74. S. Apte, B. Radetich, S. Shin, and T. V. Rajanbabu, Org. Lett., 2004, 6, 4053. CrossRef
75. R. Jana, S. Samanta, and J. K. Ray, Tetrahedron Lett., 2008, 49, 851. CrossRef
76. S. Samanta, H. Mohapatra, R. Jana, and J. K. Ray, Tetrahedron Lett., 2008, 49, 7153. CrossRef
77. H. Zhang, E. M. Ferreira, and B. M. Stoltz, Angew. Chem. Int. Ed., 2004, 43, 6144. CrossRef
78. W. S. Yoon, S. J. Lee, S. K. Kang, D. C. Ha, and J. D. Ha, Tetrahedron Lett., 2009, 50, 4492. CrossRef
79. K. C. Majumdar, S. Chakravorty, and N. De, Tetrahedron Lett., 2008, 49, 3419. CrossRef
80. S. Mrten, R. Frohlich, O. Kataeva, and P. Metz, Adv. Synth. Catal., 2005, 347, 754. CrossRef
81. J. E. M. N. Klein, H. Muller-Bunz, Y. Ortin, and P. Evans, Tetrahedron Lett., 2008, 49, 7187. CrossRef
82. K. C. Majumdar, B. Chattopadhyay, and B. Sinha, Synthesis, 2008, 23, 3857. CrossRef