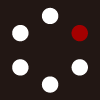
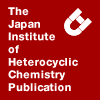
HETEROCYCLES
An International Journal for Reviews and Communications in Heterocyclic ChemistryWeb Edition ISSN: 1881-0942
Published online by The Japan Institute of Heterocyclic Chemistry
e-Journal
Full Text HTML
Received, 16th June, 2010, Accepted, 10th August, 2010, Published online, 10th August, 2010.
DOI: 10.3987/COM-10-11996
■ Substituent Effect of 7-Hydroxy-1-methylquinolinium Derivatives: A Photochemical Approach to Development of New Fluorescent pH Indicator
Naoko Senda, Atsuya Momotake, and Tatsuo Arai*
Department of Chemistry, Graduate School of Pure and Applied Sciences, University of Tsukuba, 1-1-1 Ten-nodai, Tsukuba-shi, Ibaraki 305-0006, Japan
Abstract
Substituent effect of N-methyl-7-hydroxyquinolinium derivatives on photochemical properties in aqueous solution has been investigated. The efficiency of fluorescence emission from excited zwitter ion enhanced significantly by introduction of methyl group(s). pH Dependence of photochemical behavior of the quinolinium derivatives is also investigated.7-Hydroxyquinoline (HQ) is known to form a hydrogen-bonding chain with protic solvents, through which proton or hydrogen atom relay takes place in the excited singlet state.1-3 The solvent assisted proton relay mechanism in this type of molecule has been of interest because the processes may serve as simple models for proton migration in biological systems. However the behavior is slightly complicated, since HQ can take four prototropic species including a normal form (N), an N-protonated cation form (C), a OH-deprotonated anion form (A), and an N-protonated and OH-deprotonated zwitter ion form (Z) (Scheme 1a), and these four species are in equilibrium in neutral aqueous solution.4-6 When the quinolinium nitrogen in HQ is functionalized by treatment with such methyl iodide, the equilibrium can be simplified: 7-hydroxy-1-methylquinolinium iodide (1) can take only two prototropic species, a cation form (C) at acidic pH and a zwitter ion form (Z) at neutral to basic pH (Scheme 1b).7,8 The 7-hydroxyl group of 1 was found to exhibit a strong photoacidity. For example, excited-state protolysis can occur even in the presence of 10 M perchloric acid.7 The electron-withdrawing quinolinium cation part probably amplify the photoacidity.
Quinolinium iodide 1 shows the biological activity. For example, applying a series of isomeric 1-methylhydroxyquinolinium ions to the electroplax, 1 was found to be a stronger receptor inhibitor compared to 1-methylquinolium,9 where 7-hydroxyl group of 1 is expected for the formation of a hydrogen bond to the receptor.10 Compound 1 is also used as a fluorescent probe, by monitoring the fluorescence intensity upon progressing of an acetylcholinesterase-catalyzed hydrolysis of either 7-acetoxy1-methylquinolinium iodides11-13 or 7-dimethylcarbamoxy-1-methylquinolinium iodide.14
Very recently, we have reported the new HQ-based fluorescent dye, Tsukuba-Green (TG), 4-ethoxycarbonylmethyl-7-hydroxy-2-methylquinoline (Figure 1).15 Photosensitivity, product of the molar extinction coefficient and fluorescence quantum yield (ε·ΦF) of TG is 4-5 hold greater than that of the parent compound HQ. During the course of the study we have found that both acylation of 7-OH and N-methylation of TG (TG’-Ac) is essential to penetrate the cell membrane. Since TG’-Ac is non-fluorescent, observation of immediate staining of HEp-2 cells after treatment of TG’-Ac indicates that TG’-Ac passed rapidly through the plasma membrane followed by hydrolysis via intracellular esterase to give quinolinium ion TG’ which was highly fluorescent. In fact, ε·ΦF value of the
quinolinium ion TG’ (ε·ΦF = 4200) is more than 2-fold larger than that of quinolinium ion 1 (ε·ΦF = 2000). This significant enhancement of photosensitivity led us to assume the favorable effect of alkyl substituents on its photosensitivity of 1.
In this paper we wish to report the substituent effect of 7-hydroxy-1-methylquinolinium ions on its photosensitivity. On this aspect, two methyl analogues, 7-hydroxy-1,2-dimethylqunolinium iodide 2 and 7-hydroxy-1,2,4-trimethylquinolinium iodide 3 (Figure 1) are prepared and their photochemical properties are compared to the parent compound 1. In addition pH dependence of photochemical behavior of 1-3 is also investigated.
7-Hydroxy-1-methylquinolinium iodides 1,15 2, 316 were prepared from HQ, 7-hydroxy-2-methylquinoline and 7-hydroxy-2,4-dimethylquinoline,17 respectively, by treatment with methyl iodide. The preparation of the starting materials and photochemical data of 1 have been reported elsewhere.15
Figure 2 shows the steady-state absorption (2a) and fluorescence (2b) spectra of 1-3 in water (1% DMSO). Compare to the absorption band of 1, slight hypsochromic shift and a small shoulder at 360 nm were observed in 2, and these features were prominent in 3. The ε values at the maximum wavelength at pH
7.0 were 10300, 8400, and 8100 M-1cm-1, for 1, 2, and 3, respectivley. The spectral difference is caused by the difference of pKa values at 7-hydroxyl group: pKa values of 1, 2 and 3 were estimated to be 6.09, 6.25, and 6.45, respectively, showing that the pKa values increased by introduction of methyl group(s) (Table 1). At pH 7.0, 1 mostly takes Z form, while population of C form is not negligible for 2 and 3. The formation of C form of 3 is more significant at pH 7.0 due to its higher pKa value than those of 1 and 2, resulted in the smaller ε value at the band for Z form. As in Figure 2b, a single fluorescence band around 500 nm appeared for each compound, ascribed to emission from the excited Z form.6 The fluorescence slightly shifted to shorter wavelength from 517 nm for 1 to 500 and 496 nm for 2 and 3, respectively. In each compound, the fluorescence spectra by excitation at different wavelength were almost identical, and all three compounds showed a single fluorescence decay curve. It is noteworthy that the quantum yield of fluorescence of 2 (Φf = 0.36) and 3 (Φf = 0.45) are much higher than that of 1 (Φf = 0.19). The fluorescence lifetime of 2 (τ = 9.1 ns) and 3 (τ = 10.0 ns) are also longer compared to that for 1 (τ = 7.4 ns). One can calculate the fluorescence rate constant kf and non-radiative rate constant kd from the observed τ and Φf (Table 1). While the value of the fluorescence rate constant kf increased in the order of 1, 2, and 3, the other non-radiative rate constant kd decreased in the same order. These facts indicate that the introduction of methyl group increased the kf value and decreased the kd value in 7-hydroxy-1-methylquinolinium iodides. This should be the reason why Φf value increased in the order of 1, 2, and 3. The photosensitivity, the product of the molar extinction coefficient and fluorescence quantum yield (ε·ΦF) for 2 (ε·ΦF = 3000) and 3 (ε·ΦF = 3700), respectively, is about 1.5-1.9-fold greater than that of 1 (ε·ΦF = 2000) (Table 1).
The absorption spectra of all three compounds changed depending on pH, while the fluorescence spectra are almost identical at vairous pH values. The apparent absorption spectra of 1, 2, and 3 in aqueous bufferd solution at various pH are shown in Figure 3. At pH 7.0 (solid line in Figure 3a-3c), the absorption spectra of each compound correspond with those in Figure 2a. The absorption spectra at pH
2.0 (closed circle) are dominated by C form, and those at pH 12 (opened circle) are due to Z form. The spectra of 2 and 3 at pH = 7.0 are composed of both C and Z forms. The pH dependence of absorption spectra of 2 and 3 are shown in Figure S1. Unlike the pH dependence observed in the absorption, there Figure 3. pH dependence of the absorption spectra of 1 (a), 2 (b), and 3 (c) at pH 2.0 (closed circle), pH 7.0 (solid line), and pH 12.0 (opened circle), respectively. Inset of each figure shows the normalized fluorescence spectra (excited at 350 nm) at pH 2.0 (closed circle), pH 7.0 (solid line), and pH 12.0 (opened circle), respectivelyis practically no change in the fluorescence spectra of each compound (Inset of Figure 3) measured in acidic (pH = 2), neutral (pH = 7), and basic (pH = 12). In addition, the fluorescence quantum yield of all compounds did not depend on pH. Furthermore, the fluorescenc excitation spectra of each compound are very similar to the corresponding absorption spectra, and are also changed with changing pH in similar manner (Figure S2). This indicates that the structure of the emission state does not depend on
pH at least between pH = 2 and pH = 12, because the excitation of these compounds renders the 7-hydroxyl group to be more acidic than that in the ground state. The schematic representation of the structural change of 1 in different pH is shown in Scheme 2.
Since intracellular pH is generally between 6.8-7.4 in the cytosol, these dyes may be a potential candidate for pH indicator for inside live cells. Remarkably, the pKa values of 3 (Table 1) is appropriate for detection of intracellular pH change. In fact, excitation of 3 at 400 nm light displayed a dramatic change in the fluorescence especially between pH 6.0-7.5 (Figure 4) which is close to the cytosolic pH. This change in the fluorescence intensity probably is large enough to detect intracellular pH changes. In addition, the absorption wavelength of 3 is different from similar fluorescein-based fluorescence pH indicators, such as BCECF and SNAFL that are currently used for live cell imaging,18 indicating that 3 could be used for multi-color imaging together with fluorescein-based probes. To load this dye into cells, however, further chemical modification, such as for enhancement of membrane permeability and retention inside cells, would be needed.
In summary, substituent effect of 7-hydroxy-1-methylquinolinium ions has been investigated. The efficiency of fluorescence emission from excited state Z form is enhanced significantly by introduction of methyl group(s). In aqueous solution, the products of the molar extinction coefficient and fluorescence quantum yield (ε·ΦF) for 2 and 3, respectively, are 1.5- and 1.9-fold greater than that of 1. The lifetime of fluorescence increased with increasing the fluorescence quantum yield from 7.4 ns in 1 to 9.1 ns and 10.0 ns in 2 and 3. The pH dependence of the absorption spectra was observed and the pKa values at 7-hydxoxy group increased by the effect of methyl group(s). Spectral features in the fluorescence spectra or fluorescence quantum yield did not show pH dependence (from pH 2 to 12) suggesting the same structure in emission state despite the different ground-state structure at various pH. These results presented in this paper indicate that 2 and 3 are considered to be good candidates as fluorescent chromophores for bioimaging. Further investigation for biological use of these chromophores is underway.
EXPERIMENTAL
Measurement
1H NMR spectra were measured with a JEOL EX-270 (270 MHz for 1H NMR) or a Bruker ARX-400 (400 MHz for 1H NMR) spectrometer using CD3OD as a solvent. UV absorption and fluorescence spectra were recorded on a Shimadzu UV-1600 spectrophotometer and on a Hitachi F-4500 fluorescence spectrometer, respectively. Fluorescence decay measurements were performed using time-correlated single-photon counting.
Materials
Syntheses of 1 and 3 were written in elsewhere.15, 16
7-Hydroxy-1,2-dimethylqunolinium iodide (2): A mixture of 2-methyl-7-hydroxyquiniline (107 mg, 0.67 mmol) and methyl iodide (1.0 mL, 0.70 mmol) was heated in a sealed tube at 65 °C for 23 h. The precipitate formed was collected by filtration, washed thoroughly with dichloromethane to give 94 mg of 2 in 47 % yield; 1H NMR (270 MHz, CD3OD) δ 8.78 (d, J = 8.1 Hz, 1H), 8.17 (d, J = 8.6 Hz, 1H), 7.71 (d, J = 8.4 Hz, 1H), 7.56 (s, 1H), 7.47 (dd, J = 1.9, 8.9 Hz, 1H), 4.34 (s, 3H), 3.04 (s, 3H) 13C NMR (67.5 MHz, CD3OD) δ 165.9, 160.2, 146.0, 143.8, 133.7, 124.4, 122.2, 122.1, 101.9, 39.9, 23.4. Anal. Calcd for C11H12NOI: C, 43.87; H, 4.02; N, 4.65 %. Found: C, 43.68; H, 3.90; N, 4.55 %.
ACKNOWLEDGEMENTS
This work was supported by a Grant-in-Aid for Scientific Research in a Priority Area “New Frontiers in Photochromism (No. 471) and (No. 19550176) from the Ministry of Education, Culture, Sports, Science, and Technology (MEXT), Japan.
References
1. W.-H. Fang, J. Am. Chem. Soc., 1998, 120, 7568. CrossRef
2. C. Tanner, C. Manca, and S. Leutwyler, Science, 2003, 302, 1736. CrossRef
3. H. Yu, O.-H. Kwon, and D.-J. Jang, J. Phys. Chem. A, 2004, 108, 5932. CrossRef
4. S. I. Lee and D. J. Jang, J. Phys. Chem., 1995, 99, 7537. CrossRef
5. T. G. Kim, Y. R. Kim, and D.-J. Jang, J. Phys. Chem. A, 2001, 105, 4328. CrossRef
6. T. G. Kim and M. R. Topp, J. Phys. Chem. A, 2004, 108, 10060. CrossRef
7. E. Bardez, A. Chatelain, B. Larrey, and B. Valeur, J. Phys. Chem., 1994, 98, 2357. CrossRef
8. E. Bardez, A. Fedorov, M. N. Berberan-Santos, and J. M. G. Martinho, J. Phys. Chem. A, 1999, 103, 4131. CrossRef
9. T. R. Podleski and D. Nachmansohn, Proc. Nat. Acd. Sci., 1966, 56, 1034. CrossRef
10. T. R. Podleski, Proc. Nat. Acd. Sci., 1967, 57, 268. CrossRef
11. A. K. Prince, Arch. Biochem. Biophys., 1966, 113, 195. CrossRef
12. A. K. Prince, Biochem. Pharmacol., 1966, 15, 411. CrossRef
13. F. Caturla, J. Enjo, M. C. Bernabeu, and S. L. Serre, Tetrahedron, 2004, 60, 1903. CrossRef
14. T. L. Rosenberry and S. A. Bernhardt, Biochemistry, 1971, 10, 4114. CrossRef
15. N. Senda, Y. Miwa, J. Tanaka, A. Momotake, and T. Arai, Chem. Lett., 2010, 39, 308. CrossRef
16. H. Lempert and R. Robinson, J. Chem. Soc., 1934, 1419. CrossRef
17. O. D. Fedoryak and T. M. Dore, Org. Lett., 2002, 4, 341. CrossRef
18. R. P. Haugland, Handbook of Fluorescent Probes and Research Products (Molecular Probes, Eugene, OR, ed.9, 2002).