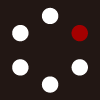
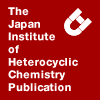
HETEROCYCLES
An International Journal for Reviews and Communications in Heterocyclic ChemistryWeb Edition ISSN: 1881-0942
Published online by The Japan Institute of Heterocyclic Chemistry
e-Journal
Full Text HTML
Received, 25th June, 2010, Accepted, 15th July, 2010, Published online, 15th July, 2010.
DOI: 10.3987/COM-10-12001
■ A Novel Pentose Synthesis via Palladium(II)-Catalyzed Cyclization of an Unstable Hemiacetal
Ken-ichiro Awasaguchi, Masahiro Miyazawa,* Ikuyo Uoya, Koichi Inoue, Koji Nakamura, Hajime Yokoyama, and Yoshiro Hirai*
Department of Chemistry, Graduate School of Science and Engineering, Toyama University, Gofuku 3190, Toyama 930-8555, Japan
Abstract
PdCl2(PhCN)2 (5 mol%)-catalyzed cyclization of a hemiacetal derived from (E,2S,3R)-2,3-isopropylidenedioxy-6-(tetrahydro-2H-pyran-2-yl)-4-hexenal and methanol gave substituted furanoside in moderate yield, exclusively via 5-exo-mode cyclization, without the need for a reoxidant. New stereogenic centers at C1 and C4 on the tetrahydrofuran ring showed preferential 1R and 4R stereochemistry due to anomeric effect (no-σ*c-o) and A1,2 strain, respectively. This methodology was applied to stereocontrolled synthesis of pentoses: D-ribose and L-lyxose.INTRODUCTION
Substituted tetrahydrofuran moieties are often found in natural products, biologically active compounds and drugs such as antivirals.1 Therefore, the stereocontrolled synthesis of substituted tetrahydrofuran derivatives has received considerable attention. Among reported methods, intramolecular cyclization of π-olefin-palladium complex is a powerful tool for the construction of heterocycles.2 However, because the Pd(II) catalyst is reduced to Pd(0) at the end of the reaction, most of these methods require a reoxidant such as O2/CuCl2 or 1,4-benzoquinone (BQ) to transform Pd(0) to Pd(II) and regenerate the catalyst. Recently, Kawai and co-workers have reported palladium(II)-catalyzed stereospecific synthesis of 2,6-substituted tetrahydropyrans from olefinic alcohol,3 and we have reported stereoselective synthesis of 2,4,6-trisubstituted tetrahydropyrans from olefinic hemiacetal without a reoxidant.4 To the best of our knowledge, however, there have been few reports on the construction of a tetrahydrofuran ring by palladium(II)-catalyzed cyclization without a reoxidant.5 As a continuation of our work, we focus here on tetrahydrofuran. Herein, we present a novel strategy for stereocontrolled tetrahydrofuran ring construction with palladium(II) catalyst via hemiacetal as a nucleophile and its application in syntheses of D-ribose and L-lyxose.6
RESULTS AND DISCUSSION
Our synthetic plan to attain a substituted tetrahydrofuran ring by palladium(II)-catalyzed cyclization is outlined in Scheme 1. The unstable hemiacetal 2 derived from aldehyde 1 and alcohol (R’OH) reacts as a nucleophile with the olefin moiety activated by palladium(II) catalyst to give the substituted furanoside 3.
Our retrosynthetic analysis for D-ribose is illustrated in Scheme 2. We envisioned that the pentose would be obtained by transformation of 4-vinylfuranoside (4), and the tetrahydrofuran ring would be constructed by palladium(II)-catalyzed cyclization of the hemiacetal derived from aldehyde 5. Aldehyde 5 would be readily prepared by side chain elongation of acetate 6, followed by oxidation of the resulting alcohol. Optically active acetate 6 was to be synthesized by asymmetric acetylation of meso-diol 7 using lipase. Finally, meso-diol 7 would be readily available from the simple achiral cis-2-butene-1,4-diol (8).
Our synthesis commenced with the benzoylation of cis-2-butene-1,4-diol (8), followed by dihydroxylation with a catalytic amount of osmium tetroxide and N-methylmorpholine N-oxide at room temperature to afford the diol 9 (Scheme 3). Protection of 9 in the presence of 2,2-dimethoxypropane as its acetonide followed by methanolysis of the resulting dibenzoate afforded the meso-diol 7 as a key intermediate. The meso-diol 7 was subjected to asymmetric acetylation with lipase AK Amano 20 in vinyl acetate to give almost optically pure acetate 6.7 The enantiopurity was confirmed by NMR analysis of both MPA esters of 6 and was determined to be >98% by 1H NMR. Protection of the hydroxyl group of 6 in the presence of tert-butyldimethylsilyl chloride, followed by methanolysis of the resulting TBS ether gave the alcohol 10. Swern oxidation of the alcohol 10 and Horner-Wadsworth-Emmons reaction of the resulting aldehyde afforded the α,β-unsaturated ester 11. Reduction of the ester 11 with DIBALH, followed by protection of the resulting alcohol in the presence of dihydropyran gave 12. Finally, removal of the TBS group in 12 using tetra-n-butylammonium fluoride and oxidation of the resulting alcohol under Ley’s condition in the presence of N-methylmorpholine N-oxide, MS 4A and a catalytic amount of tetra-n-propylammonium perruthenate afforded the aldehyde 5 in 62% yield over 13 steps from the simple achiral olefin 8.
With the requisite aldehyde 5 in hand, we next focused on palladium(II)-catalyzed cyclization (Table 1). On the basis of our previous study, the cyclization was conducted in tetrahydrofuran and PdCl2(PhCN)2 was used as a Pd(II) catalyst.4 When the aldehyde 5 was treated with 5 mol% of PdCl2(PhCN)2 and 5 equivalents of methanol in THF at room temperature, the cyclization proceeded smoothly and the cycloadduct 4 was isolated as two diastereomers in 65% yield (entry 1). The stereochemistry of these diastereomers 4A and 4B was established by means of NOE experiments, and (1R,4R) and (1R,4S)-configurations were assigned, respectively, as shown in Figure 1. Then we investigated the effects of temperature, amounts of alcohol and Pd(II) catalyst, and additive. When the cyclization was run at 0 oC, the reaction was slower and the Pd(II) catalyst was deactivated and precipitated as palladium black before the substrate 5 was completely consumed. In contrast, when the reaction was conducted at 50 oC, the substrate 5 was consumed completely in 1 h. Under these conditions (entries 2 and 3), the cycloadduct 4 was obtained in lower yield. When the cyclization was performed in the presence of 20 equivalents of methanol, the yield of the cycloadduct 4 was not improved (entry 4). Meanwhile, when the reaction was conducted in the presence of 20 mol% of Pd(II) catalyst or 5 mol% of Pd(II) catalyst, 20 mol% of 1,4-benzoquinone (BQ) as reoxidant and 20 equivalents of methanol, the yield and selectivity were much lower (entries 5 and 6, respectively).
Although the selectivity of the cyclization was seemingly low under the conditions of entries 5 and 6, this result was not due to low selectivity of the cyclization, but reflected more rapid decomposition of the cycloadduct 4A having (1R,4R)-configuration, as compared with 4B having (1R,4S)-configuration.
We then focused on the further transformation of 4A and 4B into D-ribose and L-lyxose, respectively (Scheme 4). Ozonolysis of the major cycloadduct 4A followed by in situ reduction with sodium borohydride afforded the alcohol 13A in 72% yield. Protection of the hydroxyl group of 13A in the presence of benzyl bromide and a catalytic amount of tetra-n-hexylammonium iodide gave 14A in 97% yield. Removal of the acetal groups of 14A in the presence of IR-120H, a strong acid ion-exchange resin, in water afforded the triol 15A in 82% yield.8 Finally, deprotection of the benzyl group in 15A by hydrogenolysis with Pd/C in ethanol furnished D-ribose in 99% yield. Similarly, 4B was transformed into L-lyxose in 58% yield over 4 steps.
A plausible mechanism for the Pd(II)-catalyzed cyclization of hemiacetal derived from the aldehyde 5 is illustrated in Scheme 5. First of all, deprotection of the tetrahydropyranyl group with methanol in the presence of the palladium(II) catalyst, which behaves as a Lewis acid, gives the allylic alcohol 16 (Step 1). Then, the hemiacetal intermediate is formed by the reaction of aldehyde and methanol (Step 2). Pd π-complex is formed by coordination of PdCl2Ln with the allylic alcohol, and one of the π-faces of the olefin may be preferentially recognized with the assistance of the adjacent hydroxyl group of the hemiacetal (Step 3). This complex may be present in equilibrium with four complexes (TS1, TS2, TS3 and TS4) owing to the formation of hemiacetal having other stereochemistry and coordination of PdCl2Ln with the other π-face of the olefin. Although the conformations TS1 and TS2 are stabilized by anomeric effect (no-σ∗c-o), the conformations TS3 and TS4 are not. In addition to this, although the conformations TS2 and TS4 are destabilized by A1,2 strain, the conformations TS1 and TS2 are not. Therefore, the cyclization of the hemiacetal derived from aldehyde 4 proceeds preferentially through the preferred conformation TS1. A syn-attack of the hydroxyl group in the hemiacetal on the electrophilic sp2 carbon in TS1 occurs intramolecularly from the same side of the Pd-complex in a 5-exo-trigonal fashion to give a σ-Pd complex, followed by syn-elimination of PdCl(OH)Ln to afford furanoside 4A having (1R,4R)-configuration. In the catalytic cycle, PdCl(OH)Ln may catalyze the reaction by itself or regenerate PdCl2Ln with chloride ion. For this reason, this Pd(II)-catalyzed cyclization proceeds very smoothly in the absence of any reoxidant.
In conclusion, we have developed a novel method for tetrahydrofuran ring construction by means of PdCl2(PhCN)2-catalyzed cyclization of hemiacetal as a nucleophile, without the need for a reoxidant. This cyclization proceeds under mild conditions, affording the substituted furanoside, which was used for the synthesis of pentose. Further application of this methodology to the synthesis of other sugars and natural products is in progress.
EXPERIMENTAL
General Remarks: All moisture sensitive reactions were carried out under an argon atmosphere. Anhydrous solvents were obtained as follows: tetrahydrofuran (THF) and dichloromethane were purchased from Kanto Chemical Co., Ltd.; N,N-dimethylformamide (DMF) and dimethyl sulfoxide (DMSO) was distilled from CaH2. Triethylamine was distilled from KOH. Column chromatography was performed with Silica gel 60N and Fuji BW-820. Analytical thin layer chromatography (TLC) was conducted on precoated TLC plates (silica gel 60F254, Merck) visualized under UV light and stained with either phosphomolybdic acid or p-anisaldehyde. Melting points were determined with Yanaco micro melting point apparatus and were uncorrected. IR spectra were measured with a JASCO Model FT/IR-6100 spectrometer. Mass spectra (MS) and high resolution mass spectra (HRMS) were recorded at a JEOL JMS-700 or JMS-T100TD spectrometer. Elemental analyses were performed on a Yanaco MT-5. Optical rotations ([α]D) were determined with a JASCO P-1020 polarimeter. 1H NMR spectra were recorded at 300 MHz with a JEOL JNM-ECX 300 spectrometer or 600 MHz with a JEOL JNM-ECP 600 using tetramethylsilane (TMS) as the internal standard (0.00 ppm). Chemical shifts were reported in ppm (δ) downfield from TMS. The following abbreviations were used to explain the multiplicities: s = singlet, d = doublet, t = triplet, m = multiplet. 13C NMR spectra were recorded at 75 MHz with a JEOL JNM-ECX 300 spectrometer with chemical shifts reported in ppm (δ).
cis-1,4-Dibenzoyloxy-2-butene
To a solution of diol 8 (10.0 g, 114 mmol) and pyridine (26.9 g, 340 mmol) in CH2Cl2 (200 mL) was added benzoyl chloride (36.7 g, 261 mmol) at 0 oC under an argon atmosphere. The mixture was stirred at the same temperature for 10 min and warmed up to rt. After stirring at the same temperature for 6 h, the reaction was quenched with aqueous HCl and ice cooled water. The resulting mixture was extracted with AcOEt (x 3). The combined organic layers were washed with 5% HCl, water, saturated aqueous NaHCO3 solution and brine, dried over MgSO4, and concentrated in vacuo. The resulting solid was recrystallized from n-hexane to afford the dibenzoate (33.6 g, > 99% yield) as colorless plates. mp 59.0-60.0 oC; 1H NMR (300 MHz, CDCl3) δ 8.07-8.04 (m, 4H), 7.56 (tt, J = 11.4, 7.4 Hz, 2H), 7.46-7.41 (m, 4H), 5.96 (ddd, J = 5.3, 4.0, 1.1 Hz, 2H), 5.01 (dd, J = 4.0, 1.1 Hz, 4H); 13C NMR (75 MHz, CDCl3) δ 166.3(2C), 133.1(2C), 130.0(2C), 129.7(6C), 128.4(4C), 60.6(2C); IR (KBr, cm-1) 1714, 1267, 708; MS (EI) m/z 175 (12), 149 (7), 105 (100), 77 (28); (DART) m/z 297 ([M+H]+); HRMS-DART calcd. for C18H17O4 ([M+H]+) 297.1127, found 297.1155.; Anal. Calcd. for C18H16O4 : C, 72.96, H, 5.44; Found : C, 72.86, H, 5.53.
(2R*,3S*)-1,4-Dibenzoyloxybutane-2,3-diol (9)
To a solution of the dibenzoate (5.00 g, 16.9 mmol) and N-methylmorpholine N-oxide (50% in water, 4.55 mL, 21.9 mmol) in acetone (60 mL) and water (20 mL) was added OsO4 (0.05 mol/ L in t-BuOH, 1.01mL, 0.051 mmol) at rt. The mixture was stirred at the same temperature for 18 h. The precipitate was filtered, and washed with a minimum quantity of acetone. To the filtrate was added saturated aqueous Na2S2O3 solution (50 mL) at rt and the quenched mixture was stirred until the organic phase was cleared. The resulting mixture was concentrated in vacuo. The precipitate was filtered, and washed with a minimum quantity of acetone and water. The filtrate was extracted with Et2O until the white solid was dissolved. The combined organic layers were washed with 10% HCl (x 2), water, saturated aqueous NaHCO3 solution and brine, dried over MgSO4, and concentrated in vacuo to afford the diol 9 as white solid (5.25 g, 95% yield), which was used for next step without further purification. An aliquot was crystallized from AcOEt to give an analytical pure sample. colorless needles; mp 153.0-154.0 oC; 1H NMR (300 MHz, CDCl3) δ 8.09-8.05 (m, 4H), 7.59 (tt, J = 7.4, 1.6 Hz, 2H), 7.48-7.43 (m, 4H), 4.65-4.64 (m, 4H), 4.01-3.99 (m, 2H), 2.95 (d, J = 4.5 Hz, 2H); 13C NMR (75 MHz, CDCl3) δ 167.5(2C), 133.4(2C), 129.8(4C), 129.5(2C), 128.5(4C), 70.7(2C), 66.2(2C); IR (KBr, cm-1) 3473, 1693, 1285, 1129, 710; MS (EI) m/z 269 (7), 227 (5), 195 (13), 177 (9), 165 (63), 123 (29), 105 (100), 75 (79); (DART) m/z 331 ([M+H]+); HRMS-DART calcd. for C18H19O6 ([M+H]+) 331.1182, found 331.1185.; Anal. Calcd. for C18H18O6 : C, 65.45, H, 5.49; Found : C, 65.55, H, 5.57.
(2R*,3S*)-1,4-Dibenzoyloxy-2,3-isopropylidenedioxybutane
To a solution of the diol 9 (400 mg, 1.21 mmol) and p-toluenesulfonic acid (2.1 mg, 0.0121 mmol) in CH2Cl2 (4 mL) was added 2,2-dimethoxypropane (1.48 mL, 12.1 mmol). The reaction mixture was stirred at 40 oC for 3 h. After stirring, the mixture was cooled with ice bath and the reaction was quenched with saturated aqueous NaHCO3 solution. The organic layer was separated and the aqueous phase was extracted with AcOEt (x 2). The combined organic layers were washed with brine, dried over MgSO4, filtered, and concentrated in vacuo to afford the acetonide protected dibenzoate as white solid (440 mg, 98% yield). An aliquot was recrystallized from hexane to give an analytical pure sample. colorless needles; mp 107.0-108.0 oC; 1H NMR (300 MHz, CDCl3) δ 8.08-8.03 (m, 4H), 7.56 (tt, J = 7.4, 1.5 Hz, 2H), 7.47-7.39 (m, 4H), 4.64-4.56 (m, 4H), 4.53-4.45 (m, 2H), 1.52 (s, 3H), 1.42 (s, 3H); 13C NMR (75 MHz, CDCl3) δ 166.2(2C), 133.2(2C), 129.8(4C), 129.7(2C), 128.4(4C), 109.5, 74.8(2C), 62.9(2C), 27.7, 25.4; IR (KBr, cm-1) 1714, 1270, 1116, 1082, 711; MS (EI) m/z 356 (39), 355 (89), 235 (36), 178 (28), 177 (93), 126 (52), 105 (100), 77 (73); (DART) m/z 371 ([M+H]+); HRMS-DART calcd. for C21H23O6 ([M+H]+) 371.1495, found 371.1497.; Anal. Calcd. for C21H22O6 : C, 68.10, H, 5.99; Found : C, 68.04, H, 6.14.
(2R*,3S*)-2,3-Isopropylidenedioxybutane-1,4-diol (7)
A mixture of the acetonide protected dibenzoate (89.7 g, 242 mmol) and K2CO3 (1.67 g, 12.1 mmol) in MeOH (242 mL) was stirred at rt for 15 h. The reaction was quenched with 6N HCl. After stirring for 5 min, the mixture was concentrated in vacuo. The residue was purified by silica gel column chromatography (hexane-AcOEt = 4:1 to AcOEt, gradient) to afford the diol 7 (38.5 g, 98% yield) as a white solid. mp 35.5-37.0 oC; 1H NMR (300 MHz, CDCl3) δ 4.33-4.27 (m, 2H), 3.85-3.74 (m, 4H), 2.46 (t, J = 4.6 Hz, 2H), 1.48 (s, 3H), 1.38 (s, 3H); 13C NMR (75 MHz, CDCl3) δ 108.4, 76.8(2C), 60.9(2C), 27.6, 25.1; IR (KBr, cm-1) 3401, 2988, 2938, 1382, 1218, 1049; MS (EI) m/z 149 (100), 137 (12), 123 (16), 111 (27), 97 (38), 83 (59), 71 (82), 57 (86); (DART) m/z 163 ([M+H]+); HRMS-DART calcd. for C7H15O4 ([M+H]+) 163.0970, found 163.0982.
(2S,3R)-4-Acetoxy-2,3-isopropylidenedioxybutanol (6)
A suspension of the diol 5 (406 mg, 2.50 mmol) and Lipase AK Amano 20 (56 mg) in vinyl acetate (0.51 mL, 5.51 mmol) was stirred at rt for 9.5 h under an argon atmosphere. The mixture was diluted with AcOEt and filtered through a pad of Celite®. The filtrate was concentrated in vacuo. The residue was purified by silica gel column chromatography (hexane-AcOEt = 3:2) to afford the acetate 6 (463 mg, 90% yield, >98% ee) as a colorless oil. The enantiopurity of 6 was confirmed by NMR analysis of both MPA esters. [α]20D 18.3 (c 0.40, CHCl3); 1H NMR (300 MHz, CDCl3) δ 4.39 (td, J = 6.9, 4.9 Hz, 1H), 4.33-4.27 (m, 2H), 4.14 (dd, J = 11.7, 7.2 Hz, 1H), 3.80-3.66 (m ,2H), 2.10 (s, 3H), 1.87 (t, J = 6.2 Hz, 1H), 1.50 (s, 3H), 1.39 (s, 3H); 13C NMR (75 MHz, CDCl3) δ 170.1, 109.0, 76.9, 74.6, 63.0, 60.9, 27.6, 25.1, 20.9; IR (neat, cm-1) 3476, 2988, 2938, 1742, 1373, 1240, 1167; MS (EI) m/z 189 (58), 173 (12), 131 (46), 129 (63), 115 (100), 113 (14), 87 (19), 85 (8), 73 (11), 69 (46), 59 (85); (DART) m/z 205 ([M+H]+); HRMS-DART calcd. for C9H17O5 ([M+H]+) 205.1076, found 205.1069.
(2R, 3S)-4-(tert-Butyldimethylsilyl)oxy-2,3-isopropylidenedioxybutyl acetate
To a solution of the acetate 6 (1.02 g, 4.99 mmol) in N,N-dimethylformamide (5 mL) were added imidazole (815 mg, 12.0 mmol) and t-butyldimethylsilyl chloride (902 mg, 5.99 mmol) at 0 °C under an argon atmosphere. The reaction mixture was stirred at rt for 1.5 h. The reaction mixture was diluted with Et2O, and washed with water. The aqueous layer was extracted with diethyl ether (x 1). The combined organic layers were washed with brine, dried over MgSO4, filtered, and concentrated in vacuo. The crude was purified by silica gel column chromatography (hexane-AcOEt = 9:1) to afford the TBS ether (1.58 g, 99% yield) as a colorless oil. [α]20D 32.7 (c 0.95, CHCl3); 1H NMR (300 MHz, CDCl3) δ 4.47 (dd, J = 11.6, 3.1 Hz, 1H), 4.38 (ddd, J = 8.2, 6.3, 2.9 Hz, 1H), 4.20 (dd, J = 6.3, 6.2 Hz, 1H), 4.10 (dd, J = 11.6, 8.2 Hz, 1H), 3.67 (d, J = 6.2 Hz, 2H), 2.10 (s, 3H), 1.46 (s, 3H), 1.37 (s, 3H), 0.90 (s, 9H), 0.074 (s, 3H), 0.071 (s, 3H); 13C NMR (75 MHz, CDCl3) δ 170.9. 109.0, 76.8, 75.4, 63.6, 61.5, 27.8, 25.8(3C), 25.3, 21.0, 18.2, -5.5(2C); IR (neat, cm-1) 2987, 2955, 2932, 2858, 1746, 1372, 1250, 1091, 838, 778; MS (EI) m/z 303 (64), 245 (15), 243 (14), 203 (92), 143 (100), 117 (96), 115 (67), 75 (85), 73 (77); (DART) m/z 319 ([M+H]+); HRMS-DART calcd. for C15H31O5Si ([M+H]+) 319.1941, found 319.1939.
(2R, 3S)-4-(tert-Butyldimethylsilyl)oxy-2,3-isopropylidenedioxybutanol (10)
To a suspension of the TBS ether (37.6 g, 118 mmol) and K2CO3 (1.63 g, 11.8 mmol) in MeOH (190 mL) was stirred at rt for 3 h. The reaction was quenched with water and the solvent was concentrated in vacuo. The residue was diluted with AcOEt and water. The organic layer was separated and the aqueous phase was extracted with AcOEt (x 2). The combined organic layers were washed with brine, dried over MgSO4, and concentrated in vacuo. The residue was purified by silica gel column chromatography (hexane-AcOEt = 4:1) to afford the alcohol 10 (32.3 g, 99% yield) as a colorless oil. [α] 20D 3.9 (c 0.85, CHCl3); 1H NMR (300 MHz, CDCl3) δ 4.35 (dt, J = 6.8, 5.8 Hz, 1H), 4.23 (ddd, J = 8.9, 5.9, 4.1 Hz, 1H), 3.87-3.72 (m, 3H), 3.68 (dd, J = 10.7, 4.1 Hz, 1H), 3.01 (dd, J = 8.3, 5.8 Hz, 1H), 1.42 (s, 3H), 1.36 (s, 3H), 0.90 (s, 9H), 0.109 (s, 3H), 0.108 (s, 3H); 13C NMR (75 MHz, CDCl3) δ 108.4, 77.4, 76.9, 61.6, 60.8, 27.8, 25.8(3C), 25.2, 18.2, -5.52, -5.58; IR (neat, cm-1) 3490, 2986, 2954, 2931, 2885, 2858, 1254, 1218, 1085, 1057, 837, 778; MS (EI) m/z 261 (16), 161 (71), 131 (96), 117 (89), 75 (100), 73 (78), 59 (51); (DART) m/z 277 ([M+H]+); HRMS-DART calcd. for C13H29O4Si ([M+H]+) 277.1835, found 277.1830.
Ethy (E,4R,5S)-6-(tert-Butyldimethylsilyl)oxy-4,5-isopropylidenedioxy-2-hexenoate (11)
To a solution of dimethyl sulfoxide (10.0 mL, 141 mmol) in CH2Cl2 (200 mL) was added oxalyl chloride (6.1 mL, 70.5 mmol) at -78 oC under an argon atmosphere. After stirring for 20 min, to the reaction mixture was added a solution of the alcohol 10 (13.0 g, 47.0 mmol) in CH2Cl2 (20 mL). The suspension was stirred at the same temperature for 30 min. To the mixture was added Et3N (29.5 mL, 212 mmol) at -78 oC, the suspension was warmed to rt and stirred at the same temperature for 30 min. The reaction mixture was poured into ice cooled water and 10% HCl, and the aqueous phase was extracted with CHCl3 (x 1). The combined organic layers were washed with saturated aqueous NaHCO3 solution and brine, filtered, and concentrated in vacuo to afford aldehyde (12.9 g) as a yellow color oil, which was immediately used for the next reaction without further purification. To a suspension of sodium hydride (60% in oil, 2.63 g, 65.8 mmol) in THF (250 mL) was added triethyl phosphonoacetate (14.1 mL, 70.5 mmol) at 0 oC under an argon atmosphere. The mixture was stirred at the same temperature for 30 min. To the mixture was added a solution of aldehyde (12.9 g, 47 mmol) in THF (30 mL) at -78 oC, the mixture was stirred at the same temperature for 10 min and warmed to rt. After stirring at the same temperature for 10 min, the reaction mixture was diluted with AcOEt and poured into saturated aqueous NH4Cl solution. The aqueous phase was extracted with AcOEt (x 2). The combined organic layers were washed with brine, dried over MgSO4, and concentrated in vacuo. The residue was purified by silica gel column chromatography (hexane-AcOEt = 20:1) to afford the α,β-unsaturated ester 11 (15.2 g, 94% yield in 2 steps) as a colorless oil. [α]20D 7.8 (c 0.74, CHCl3); 1H NMR (300 MHz, CDCl3) δ 6.99 (dd, J = 15.8, 5.2 Hz, 1H), 6.12 (dd, J =15.8, 1.5 Hz, 1H), 4.81 (ddd, J = 6.9, 5.2, 1.5 Hz, 1H), 4.29 (ddd, J = 8.0, 6.9, 4.6 Hz, 1H), 4.20 (q, J = 7.1 Hz, 2H), 3.63 (dd, J = 10.1, 4.6 Hz, 1H), 3.53 (dd, J = 10.1, 8.0 Hz, 1H), 1.49 (s, 3H), 1.38 (s, 3H), 1.29 (t, J = 7.1 Hz, 3H), 0.88 (s, 9H), 0.042 (s, 3H), 0.036 (s, 3H); 13C NMR (75 MHz, CDCl3) δ 166.0, 143.1, 122.3, 109.2, 78.3, 76.6, 61.8, 60.4, 27.6, 25.8(3C), 25.2, 18.2, 14.3, -5.6(2C); IR (neat, cm-1) 2985, 2955, 2931, 2886, 2858, 1724, 1257, 1162, 1097, 839, 778; MS (EI) m/z 344 (M+, 7), 343 (28), 127 (100), 117 (86), 89 (54), 75 (68), 73 (72); (DART) m/z 345 ([M+H]+); HRMS-DART calcd. for C17H33O5Si ([M+H]+) 345.2097, found 345.2105.
(E,4R,5S)-6-(tert-Butyldimethylsilyl)oxy-4,5-isopropylidenedioxy-2-hexenol
To a solution of the ester 11 (1.10 g, 3.20 mmol) in THF (16 mL) was added DIBALH (0.98 M in n-hexane, 6.6 mL, 6.4 mmol) at 0 °C under an argon atmosphere. The mixture was stirred at the same temperature for 1.5 h. The reaction was quenched sequentially with methanol, ice cooled water and 10% HCl. The aqueous phase was extracted with AcOEt (x 3), the combined organic layers were washed with saturated aqueous NaHCO3 solution and brine, dried over MgSO4, filtered and concentrated in vacuo. The residue was purified by silica gel column chromatography (hexane-AcOEt = 3:1) to afford the alcohol (0.98 g, 99% yield) as a colorless oil. [α]20D 8.1 (c 0.72, CHCl3); 1H NMR (300 MHz, CDCl3) δ 5.97 (dt, J = 15.5, 5.2 Hz, 1H), 5.80 (dd, J = 15.5, 7.3 Hz, 1H), 4.67 (dd, J = 7.3, 6.3 Hz, 1H), 4.22-4.16 (m, 3H), 3.61 (d, J = 6.3 Hz, 2H), 1.47 (s, 3H), 1.37 (s, 3H), 1.31 (t, J = 6.2 Hz, 1H), 0.88 (s, 9H), 0.06 (s, 3H), 0.05 (s, 3H); 13C NMR (75 MHz, CDCl3) δ 133.0, 126.7, 108.6, 78.5, 77.9, 63.0, 62.2, 27.9, 25.9(3C), 25.4, 18.3, -5.4(2C); IR (neat, cm-1) 3424, 2954, 2930, 2857, 1253, 1100, 838, 777; MS (EI) m/z 302 (M+, 3), 301(10), 201(13), 187(31), 169(26), 157(19), 117(62), 89(36), 75(100), 73(56), 59(29) ; (DART) m/z 303 ([M+H]+); HRMS-DART calcd. for C15H31O4Si ([M+H]+) 303.1992, found 303.1994.
(E,4R,5S)-6-(tert-Butyldimethylsilyl)oxy-4,5-isopropylidenedioxy-1-(tetrahydropyran-2-yl)oxy-2-hexene (12)
To a solution of the alcohol (8.30 g, 27.4 mmol) in CH2Cl2 (125 mL) were added p-toluenesulfonic acid (240 mg, 1.37 mmol) and 2H-dihydropyran (3.8 mL, 41.2 mmol) at 0 oC under an argon atmosphere. After stirring at the same temperature for 1 h, the reaction mixture was diluted with CHCl3 and the reaction was quenched with saturated aqueous NaHCO3 solution. The aqueous phase was extracted with CHCl3 (x 2), and the combined organic layers were washed with brine, dried over MgSO4, filtered and concentrated in vacuo. The residue was purified by silica gel column chromatography (hexane-AcOEt = 20:1) to afford the THP ether 12 (10.3 g, 97% yield as diastereomer mixtures) as a colorless oil. 1H NMR (300 MHz, CDCl3) δ 5.90 (dt, J = 15.9, 5.1 Hz, 1H), 5.79 (dd, J = 15.9, 6.2 Hz, 1H), 4.68-4.65 (m, 2H), 4.29-4.23 (m, 1H), 4.18 (dd, J = 12.5, 6.2 Hz, 1H), 3.99 (dt, J = 12.5, 5.4 Hz, 1H), 3.89-3.82 (m, 1H), 3.61 (d, J = 5.4 Hz, 2H), 3.54-3.47 (m, 1H), 1.81 - 1.67 (m, 6H), 1.47 (s, 3H), 1.37 (s, 3H), 0.88 (s, 9H), 0.054 (s, 3H), 0.049 (s, 3H); IR (neat, cm-1) 2936, 2857, 1119, 1097, 1079, 1026, 837, 777.
(E,2S,3R)-2,3-Isopropylidenedioxy-6-(tetrahydro-2H-pyran-2-yl)oxy-4-hexenol
To a solution of the THP ether 12 (21.0 g, 54.3 mmol) in THF (200 mL) was added tetra-n-butyl- ammonium fluoride (1.0 M in THF, 81.5 mL, 81.5 mmol) at 5 °C under an argon atmosphere. The reaction mixture was stirred at the same temperature for 10 min, and then warmed to rt. After stirring at the same temperature for 2 h, the mixture was diluted with Et2O, and washed with water. The aqueous phase was extracted with Et2O (x 5). The combined organic layers were washed with brine, dried over MgSO4, filtered and concentrated in vacuo. The residue was purified by silica gel column chromatography (hexane-AcOEt = 7:3 to 1:1, gradient) to afford the alcohol (14.1 g, 95% yield) as a colorless oil. 1H NMR (300 MHz, CDCl3) δ 6.01-5.92 (m, 1H), 5.83-5.74 (m, 1H), 4.70 (t, J = 6.9 Hz, 1H), 4.63 (d, J = 2.1 Hz, 1H), 4.23 (dt, J = 13.0, 6.0 Hz, 2H), 4.05 (dt, J = 13.4, 6.5 Hz, 1H), 3.89-3.82 (m, 1H), 3.60-3.57 (m, 2H), 3.54-3.47 (m, 1H), 2.05-1.53 (m, 7H), 1.51 (s, 3H), 1.39 (s, 3H); IR (neat, cm-1) 3419, 2943, 2873, 1714, 1372, 1218, 1123, 1074, 1035, 975, 756; MS (DART) m/z 273 ([M+H]+); HRMS-DART calcd. for C14H25O5 ([M+H]+) 273.1702, found 273.1702.
(E,2S,3R)-2,3-Isopropylidenedioxy-6-(tetrahydro-2H-pyran-2-yl)-4-hexenal (5)
To a suspension of the alcohol (1.05 g, 3.86 mmol) and MS 4A (2.00 g) in CH2Cl2 (50 mL) was added N-methylmorphorine N-oxide (905 mg, 7.71 mmol), then the mixture was stirred for 1 h at rt. To the mixture was added tetra-n-propylammnomium perruthenate (67 mg, 1.93 mmol) at 5 oC, and the mixture was stirred at the same temperature for 40 min. The mixture was diluted with CH2Cl2, filtered through a pad of Celite® and the pad was washed with CH2Cl2. The filtrate was washed with saturated aqueous Na2S2O3 solution, 1N HCl and brine, dried over MgSO4 and concentrated in vacuo. The residue was purified by silica gel column chromatography (hexane-AcOEt = 1:1) to afford the aldehyde 5 (960 mg, 92% yield) as a yellow oil. 1H NMR (300 MHz, CDCl3) δ 9.57 (d, J = 3.1 Hz, 1H), 6.01 (dt, J = 15.3, 5.2 Hz, 1H), 5.67 (dddd, J = 15.3, 7.4, 2.9, 1.5 Hz, 1H), 4.89 (t, J = 7.2 Hz, 1H), 4.62-4.59 (m, 1H), 4.41 (dd, J = 7.4, 2.9 Hz, 1H), 4.30-3.78 (m, 3H), 3.55-3.45 (m, 1H), 1.88-1.35 (m, 6H), 1.63 (s, 3H), 1.44 (s, 3H); IR (neat, cm-1) 2984, 2940, 2871, 1736, 1382, 1218, 1119, 1068, 1025, 973, 870; MS (DART) m/z 271
([M+H]+); HRMS-DART calcd. for C14H23O5 ([M+H]+) 271.1546, found 273.1545.
(3R,4R)-3,4-Isopropylidenedioxy-5-methoxy-2-vinyltetrahydrofuran (4)
To a solution of the aldehyde 5 (200 mg, 0.740 mmol) and MeOH (119 mg, 3.70 mmol) in THF (37 mL) was added PdCl2(PhCN)2 (14 mg, 0.037 mmol) at rt under an argon atmosphere, then the mixture was stirred at the same temperature for 2 h. The reaction mixture was filtered through a pad of Florisil®, the pad was washed with AcOEt, and the filtrate was concentrated in vacuo. The residue was purified by silica gel column chromatography (hexane-AcOEt = 10:1) to afford the olefin 4 (104 mg, 70% yield) as a colorless oil. (2R,3R,4R,5R)-4A;colorless oil; [α]20D -52.6 (c 0.84, CHCl3); 1H NMR (300 MHz, CDCl3) δ 5.88 (ddd, J = 17.9, 10.0, 8.0 Hz, 1H), 5.27 (ddd, J =17.9, 1.3, 1.2 Hz, 1H), 5.16 (ddd, J =10.0, 1.3, 1.2 Hz, 1H), 4.99 (s, 1H), 4.631 (d, J = 8.0 Hz, 1H), 4.626 (s, 2H), 3.35 (s, 3H), 1.50 (s, 3H), 1.32 (s, 3H); 13C NMR (75 MHz, CDCl3) δ 137.6, 117.3, 112.3, 109.3, 88.4, 85.5, 84.6, 54.6, 26.5, 25.0; IR (neat, cm-1) 2988, 2938, 2833, 1373, 1210, 1105, 1057, 1031, 868; MS (EI) m/z 280 (7), 279 (16), 243 (7), 225 (5), 205 (10), 185 (18), 169 (26), 167 (29), 165 (24), 149 (100), 111 (31); (DART) m/z 201 ([M+H]+); HRMS-DART calcd. for C10H17O4 ([M+H]+) 201.1127, found 201.1129. (2S,3R,4R,5R)-4B; colorless oil; [α]20D -24.6 (c 0.83, CHCl3); 1H NMR (300 MHz, CDCl3) δ 6.00 (ddd, J = 17.5, 10.3, 7.3 Hz, 1H), 5.45-5.38 (m, 1H), 5.37-5.32 (m, 1H), 4.92 (s, 1H), 4.68 (dd, J = 5.8, 3.7 Hz, 1H), 4.58 (d, J = 5.8 Hz, 1H), 4.39 (dd, J = 7.3, 3.7 Hz, 1H), 3.35 (s, 3H), 1.47 (s, 3H), 1.32 (s, 3H); 13C NMR (75 MHz, CDCl3) δ 132.3, 119.1, 112.6, 107.2, 85.3, 81.5, 81.1, 54.7, 26.1, 24.9; IR (neat, cm-1) 2989, 2937, 1373, 1211, 1104, 1021, 758; MS (EI) m/z : 279 (21), 243 (3), 239 (2), 205 (3), 185 (27), 167 (29), 149 (100), 125 (13), 111 (14); (DART) m/z 201 ([M+H]+); HRMS-DART calcd. for C10H17O4 ([M+H]+) 201.1127, found 201.1123.
((2R,3R,4R,5R)-(3,4-Isopropylidenedioxy-5-methoxy-2-tetrahydrofuranyl))methanol (13A)
A gas of O3 was bubbled into a solution of the olefin 4A (300 mg, 1.50 mmol) in MeOH (10 mL) at -78 oC for 30 min, then O2 was bubbled into the reaction mixture until the blue color solution was cleared. To the reaction mixture was added NaBH4 (85 mg, 2.25 mmol) at 0 oC and the mixture was stirred at rt for 22 h. The mixture was concentrated in vacuo, the residue was diluted with AcOEt and quenched with 1N HCl. The aqueous phase was extracted with AcOEt. The combined organic layers were washed with saturated aqueous NaHCO3 solution and brine, dried over MgSO4, and concentrated in vacuo. The residue was purified by silica gel column chromatography (hexane-AcOEt = 3:1) to afford the alcohol 13A (220 mg, 72% yield) as a colorless oil. [α]20D -72.2 (c 1.02, CHCl3); 1H NMR (300 MHz, CDCl3) δ 4.98 (s, 1H), 4.84 (d, J = 5.8 Hz, 1H), 4.59 (d, J = 5.8 Hz, 1H), 4.44 (dd, J = 3.1, 2.7 Hz, 1H), 3.70 (ddd, J = 14.3, 2.7, 2.7 Hz, 1H), 3.61 (ddd, J = 14.3, 10.3, 3.1 Hz, 1H), 3.44 (s, 3H), 3.23 (dd, J = 10.3, 2.7 Hz, 1H), 1.49 (s, 3H), 1.32 (s, 3H); 13C NMR (75 MHz, CDCl3) δ 112.1, 109.9, 88.3, 85.8, 81.4, 63.9, 55.5, 26.3, 24.6; IR (neat, cm-1) 3447, 2988, 2941, 1383, 1374, 1211, 1093, 1044, 871; MS (EI) m/z 259 (2), 203 (2), 201 (4), 189 (91), 173 (100), 157 (55), 129 (29), 115 (36), 113 (82); (DART) m/z 205 ([M+H]+); HRMS-DART calcd. for C9H17O5 ([M+H]+) 205.1076, found 205.1080.
((2S,3R,4R,5R)-(3,4-Isopropylidenedioxy-5-methoxy-2-tetrahydrofuranyl))methanol (13B)
A gas of O3 was bubbled into a solution of the olefin 4B (150 mg, 0.75 mmol) in MeOH (8 mL) at -78 oC for 30 min, then O2 was bubbled into a reaction mixture until the blue color solution was cleared. To the reaction mixture was added NaBH4 (43 mg, 1.12 mmol) at 0 oC and the mixture was warmed to rt. After stirring at the same temperature for 21 h, the mixture was concentrated in vacuo. The residue was diluted with AcOEt and 1N HCl. The aqueous phase was extracted with AcOEt (x 3). The combined organic layers were washed with saturated aqueous NaHCO3 solution and brine, dried over MgSO4 and concentrated in vacuo. The crude oil was purified by silica gel column chromatography (hexane-AcOEt = 4:1 to 2:1, gradient) to afford the alcohol 13B (153 mg, < 85% yield) as an inseparable mixture which was colorless oil. 13C NMR (75 MHz, CDCl3) δ 112.7, 107.0, 85.2, 80.4, 79.2, 61.1, 54.7, 25.9, 24.5.
(2R,3R,4R,5R)-2-Benzyloxymethyl-3,4-isopropylidenedioxy-5-methoxytetrahydrofuran (14A)
To a solution of the alcohol 13A (1.00 g, 4.90 mmol) in THF (50 mL) was added NaH (235 mg, 5.39 mmol) at 5 °C, then the mixture was stirred at rt for 1 h. To a suspension were added a solution of benzyl bromide (1.17 g, 6.86 mmol) and tetra-n-hexylammonium iodide (236 mg, 0.49 mmol) at 5 °C, then the mixture was stirred at rt for 4.5 h. The reaction mixture was cooled with ice bath and the reaction was quenched with saturated aqueous NH4Cl solution. The aqueous phase was extracted with AcOEt (x 2). The combined organic layers were washed with brine, dried over MgSO4, filtered and concentrated in vacuo. The crude oil was purified by silica gel column chromatography (hexane-AcOEt = 15:1) to afford the benzyl ether 14A (1.40 g, 97% yield) as a yellow oil. [α]20D -52.2 (c 1.94, CHCl3); 1H NMR (300 MHz, CDCl3) δ 7.36-7.28 (m, 5H), 4.96 (s, 1H), 4.68 (dd, J = 5.8, 1.0 Hz, 1H), 4.57 (d, J = 5.8 Hz, 1H), 4.56 (s, 2H), 4.38 (ddd, J = 8.8, 7.8, 1.0 Hz, 1H), 3.53 (dd, J = 8.8, 6.5 Hz, 1H), 3.46 (dd, J = 7.8, 1.0 Hz, 1H), 3.29 (s, 3H), 1.49 (s, 3H), 1.32 (s, 3H); 13C NMR (75 MHz, CDCl3) δ 138.0, 128.4(3C), 127.7(2C), 112.4, 109.3, 85.19, 85.18, 82.2, 73.3, 71.1, 54.8, 26.5, 25.0; IR (neat, cm-1) 2988, 2938, 2862, 1455, 1382, 1373, 1211, 1109, 1062, 871, 739, 699; MS (EI) m/z 279 (26), 248 (31), 247 (100), 173 (36), 157 (26), 115 (30), 113 (33), 107 (42); (DART) m/z 295 ([M+H]+); HRMS-DART calcd. for C16H23O5 ([M+H]+) 295.1546, found 295.1548.
(2S,3R,4R,5R)-2-Benzyloxymethyl-3,4-isopropylidenedioxy-5-methoxytetrahydrofuran (14B)
To a solution of the alcohol 13B (180 mg, 0.88 mmol) in THF (8.8 mL) was added NaH (64 mg, 1.59 mmol) at rt, then the mixture was stirred at the same temperature for 30 min. To a suspension were added a THF solution of benzyl bromide (316 mg, 1.85 mmol) and tetra-n-hexylammonium iodide (42 mg, 0.09 mmol) at rt, then the mixture was stirred at the same temperature for 23 h. The reaction was quenched with saturated NH4Cl solution, then the aqueous phase was extracted with AcOEt (x 2). The combined organic layers were washed with brine, dried over MgSO4, filtered and concentrated in vacuo. The crude oil was purified by silica gel column chromatography (hexane-AcOEt = 10:1) to afford the benzyl ether 14B (242 mg, 93% yield) as a yellow oil. [α]20D - 31.9 (c 0.72, CHCl3); 1H NMR (300 MHz, CDCl3) δ 7.38-7.26 (m, 5H), 4.92 (s, 1H), 4.72 (dd, J = 5.8, 3.8 Hz, 1H), 4.67 (d, J = 12.0 Hz, 1H), 4.55 (d, J =12.0 Hz, 1H), 4.55 (d, J = 5.8 Hz,1H), 4.17 (ddd, J = 7.1, 4.2, 3.8 Hz, 1H), 3.83 (dd, J = 10.3, 4.2 Hz, 1H), 3.70 (dd, J = 10.5, 7.1 Hz, 1H), 3.34 (s, 3H), 1.43 (s, 3H), 1.30 (s, 3H); 13C NMR (75 MHz, CDCl3) δ 138.2, 128.4(2C), 127.8(2C), 127.6, 112.6, 107.3, 84.9, 80.0, 79.0, 73.5, 68.3, 54.7, 26.1, 24.9; IR (neat, cm-1) : 2989, 2936, 1455, 1373, 1210, 1102, 874, 739, 698; MS (EI) m/z 279 (10), 178 (18), 145 (9), 107 (20), 92 (23), 91 (100), 87 (22), 77 (15), 55 (15); (DART) m/z 295 ([M+H]+); HRMS-DART calcd. for C16H23O5 ([M+H]+) 295.1546, found 295.1544.
5-O-Benzyl-D-ribose (15A)
To a suspension of the benzyl ether 14A (260 mg, 0.88 mmol) in water (8.8 mL) was added Amberlite IR-120H (2.00 g) at rt, then the mixture was stirred at rt for 28 h. The mixture was saturated with NaCl and AcOEt was added to the mixture. After vigorously stirring for 5 min, the mixture was filtered and the aqueous phase was extracted with AcOEt (x 3). The combined organic layers were washed with brine, dried over MgSO4, filtered and concentrated in vacuo. The crude oil was purified by silica gel column chromatography (hexane-AcOEt = 1:10) to afford the triol 15A (173 mg, 82% yield) as a white solid. [α]20D 38.2 (c 0.86, CHCl3); 1H NMR (300 MHz, CD3OD) δ 7.38-7.23 (m, 5H), 5.23 (d, J = 3.6 Hz, 0.3H), 5.12 (s, 0.7H), 4.58 (s, 1.4H), 4.54 (s, 0.6H), 4.14-4.07 (m, 1H), 4.03-3.93 (m, 1.4H), 3.81 (dd, J = 4.8,1.0 Hz, 0.6H), 3.69 (dd, J = 10.5, 3.6 Hz, 0.6H), 3.63-3.52 (m, 1.4H); 13C NMR (75 MHz, CD3OD) δ major epimer : 139.6, 129.4(2C), 128.9(2C), 128.8, 103.3, 82.8, 77.0, 74.4, 73.3, 72.8; minor epimer : 139.6, 129.4(2C), 128.69, 128.66(2C), 98.0, 83.4, 74.4, 72.43, 72.38, 71.6; IR (KBr, cm-1) 3421, 3309, 2937, 2867, 1456, 1246, 1128, 1091, 1051, 1010, 948, 933, 844, 743, 698; MS (EI) m/z 222 (6), 149 (5), 131 (7), 107 (41), 92 (47), 91 (100), 73 (19), 65 (25); (DART) m/z 241 ([M+H]+); HRMS-DART calcd. for C12H17O5 ([M+H]+) 241.1076, found 241.1167.
5-O-Benzyl-L-lyxose (15B)
To a suspension of the benzyl ether 14B (200 mg, 0.68 mmol) in water (5.1 mL) and 1,4-dioxane (5.1 mL) was added Amberlite IR-120H (2.00 g) at rt, then the mixture was stirred at rt for 106 h. The reaction mixture was filtered, the residue was washed with 1,4-dioxane and the filtrate was concentrated in vacuo. The crude oil was purified by silica gel column chromatography (hexane-AcOEt = 1:9) to afford the triol 15B (121 mg, 74 % yield) as a colorless oil. [α]20D -36.9 (c 0.53, CHCl3); 1H NMR (300 MHz, CD3OD) δ 7.28-7.12 (m, 5H), 5.08 (d, J = 3.2 Hz, 0.65H), 5.01 (d, J = 4.7 Hz, 0.35H), 4.48-4.43 (m, 2H), 4.25-4.16 (m, 1.65H), 3.90 (t, J = 4.8 Hz, 0.35H), 3.81 (dd, J = 4.3, 3.2 Hz, 0.65H), 3.72 (dd, J = 10.0, 4.7 Hz, 0.35H), 3.64 (dd, J = 10.7, 4.3 Hz, 0.65H), 3.63-3.47 (m, 0.7H), 3.53 (dd, J = 10.7, 6.2 Hz, 0.65H) 13C NMR (75 MHz, CD3OD) δ major epimer : 139.6, 129.4(2C), 129.0(2C), 128.7, 103.0, 80.0, 78.6, 74.4, 72.6, 70.5; minor epimer : 139.5, 128.94(2C), 128.91, 128.87, 128.7, 97.6, 80.5, 74.3, 73.5, 72.1, 71.4; IR (neat, cm-1): 3388, 2926, 2870, 2514, 1076, 1027, 742, 699; MS (EI) m/z: 178 (3), 149 (4), 108 (9), 107 (23), 92 (13), 91 (100), 79 (10), 77 (11), 65 (10); (DART) m/z 241 ([M+H]+); HRMS-DART calcd. for C12H17O5 ([M+H]+) 241.1076, found 241.1079.
D-Ribose
To a solution of the triol 15A (50 mg, 0.21 mmol) in EtOH (2.1 mL) was added Pd/C (5 mg), then the reaction vessel was evacuated and back-filled with H2 (x 5). After stirring at rt for 51 h, the mixture was filtered through a pad of Celite® and the pad was washed with MeOH. The filtrate was concentrated in vacuo. The crude material was purified by silica gel column chromatography (MeOH) to afford D-ribose (31 mg, >99 % yield) as a colorless amorphous solid. [α]20D -23.5 (c 1.50, MeOH); 1H NMR (300 MHz, CD3OD) δ 4.91 (d, J = 4.8 Hz, 0.8H), 4.76 (d, J = 2.1 Hz, 0.2H), 3.91 (dd, J = 3.1, 2.7 Hz, 0.8H), 3.88-3.52 (m, 2.4H), 3.83 (dd, J = 8.4, 2.7 Hz, 0.8H), 3.49 (d, J = 3.1 Hz, 0.2H), 3.46-3.43 (m, 0.8H); 13C NMR (75 MHz, CD3OD) δ major epimer : 96.0, 73.3, 70.2, 69.2, 64.6; minor epimer : 95.3, 72.1, 71.3, 69.0, 64.6; MS (EI) m/z: 149 (1), 119 (5), 101 (5), 86 (18), 73 (100), 71 (21), 61 (19), 60 (58), 57 (15).
L-Lyxose
To a suspension of the triol 15B (36 mg, 0.15 mmol) in EtOH (2.5 mL) was added Pd/C (5 mg), then the reaction vessel was evacuated and back-filled with H2 (x 5). After stirring for 19.5 h at rt, the mixture was filtered over a pad of Celite® and the pad was washed with EtOH. The filtrate was concentrated in vacuo. The crude material was purified by silica gel column chromatography (MeOH) to afford L-lyxose (23 mg, >99 % yield) as a colorless amorphous solid. [α]20D 8.6 (c 1.13, MeOH); 1H NMR (300 MHz, CD3OD) δ 4.83 (d, J = 3.4 Hz, 0.8H), 4.71 (br s, 0.2H), 3.88 (dd, J = 11.9, 3.6 Hz, 0.2H), 3.72-3.47(m, 5.6H), 3.14 (dd, J = 11.7, 6.9 Hz, 0.2H); 13C NMR (75 MHz, CD3OD) δ major epimer : 96.0, 72.5, 72.2, 69.2, 64.3; minor epimer : 96.0, 74.4, 70.1, 69.3, 64.1; MS (EI) m/z: 149 (1), 119 (6), 101 (6), 91 (7), 86 (8), 73 (100), 71 (21), 61 (28), 60 (63), 57 (16).
ACKNOWLEDGEMENTS
This study was financially supported by a Grant-in-Aid for Scientific Research from the Ministry of Education, Culture, Sports, Science, and Technology of Japan.
References
1. C. Mathe and G. Gosselin, Antiviral Research, 2006, 71, 276. CrossRef
2. G. Zeni and R. C. Larock, Chem. Rev., 2004, 104, 2285; CrossRef E. M. Beccalli, G. Broggini, M. Martinelli, and S. Scottocornola, Chem. Rev., 2007, 107, 5318. CrossRef
3. N. Kawai, J. M. Lagrange, M. Ohmi, and J. Uenishi, J. Org. Chem., 2006, 71, 4530. CrossRef
4. M. Miyazawa, Y. Hirose, M. Narantsetseg, H. Yokoyama, S. Yamaguchi, and Y. Hirai, Tetrahedron Lett., 2004, 45, 2883. CrossRef
5. A. Tenaglia and F. Kammerer, Synlett, 1996, 576; CrossRef J. Uenishi, Y. S. Vikhe, and N. Kawai, Chem. Asian J., 2008, 3, 473. CrossRef
6. Total synthesis of 2-deoxy-D-ribose using Pd(II) catalyst, as a related articule will be published in this journal, M. Miyazawa, K. Awasaguchi, I. Uoya, H. Yokoyama, and Y. Hirai, Heterocycles, 2010, 81, 1891. CrossRef
7. K. Mori and H. Kiyota, Liebigs Ann. Chem., 1992, 989. CrossRef
8. H. Takahashi, Y. Iwai, Y. Hitomi, and S. Ikegami, Org. Lett., 2002, 4, 2401 CrossRef