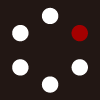
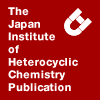
HETEROCYCLES
An International Journal for Reviews and Communications in Heterocyclic ChemistryWeb Edition ISSN: 1881-0942
Published online by The Japan Institute of Heterocyclic Chemistry
e-Journal
Full Text HTML
Received, 28th June, 2010, Accepted, 30th August, 2010, Published online, 13th September, 2010.
DOI: 10.3987/COM-10-12002
■ UNIQUE PHENYL ETHER TYPE BIFLAVONOIDS, ZIZYFLAVOSIDES A AND B WITH BIOMIMETIC SYNTHESIS FOR ZIZYFLAVOSIDE A
Mahmoud Mostafa,* Fumihide Takano, and Tomihisa Ohta*
Department of Pharmacognosy and Chemistry of Natural Products, Graduate School of Natural Science and Technology, Kanazawa University, Kakuma, Kanazawa, Ishikawa 920-1192, Japan
Abstract
During our investigation of Zizyphus spina-christi (L.) Willd. leaves for anti-inflammatory activity, eight compounds were characterized. Two are new phenyl ether type biflavonoids, which were assigned as zizyflavosides A (1) and B (2). The structures of 1 and 2 were determined by spectroscopic analyses including 1D, 2D NMR and MS spectroscopic and chemical data. The other isolated compounds were known flavonoids kaempferol-3-O-rutinoside (5), quercetin-3-O-rutinoside (6), 3',5'-di-C-β-D-glucosylphloretine (7), quercetin-3-O-[β-D-xylosyl-(1→2)-α-L-rhamnoside]-4'-O-α-L-rhamnoside (8), epiafzelechin (9) and quercetin-3-O-[β-D-xylosyl-(1→2)-α-L-rhamnoside] (10). Zizyflavoside A was synthesized from quercetin-3-O-rutinoside and kaempferol-3-O-rutinoside using laccase as an enzyme and this biomimetic synthesis could be indication to its biosynthetic pathway.INTRODUCTION
Zizyphus spina-christi L. Willd. (Rhamnaceae) is a subtropical plant known in Egypt as ‘Nabq’ or ‘Sidr’.1,2 This plant widely spread in the Mediterranean region, Africa and Asia and used for various medicinal purposes.3-7 In Arabian folk medicine the leaves are used to heal wounds, treat some skin diseases, some inflammatory conditions, sores, against ringworm, fever, gonorrhea and ulcers.8 The phytochemical composition in the literature reported the presence of betulinc and ceanolic acids, cyclopeptide alkaloids, triterpenoid saponins and flavonoids.9-14
Eight constituents were isolated and characterized from Zizyphus spina-christi during our research for anti-inflammatory constituents. Two are new phenyl ether type biflavonoids, for which 1 and 2 have been assigned with spectroscopic and chemical data (Figure 1). The biomimetic synthesis of 1 was done.
RESULTS AND DISCUSSION
ZIZYFLAVOSIDE A
Zizyflavoside A (1), isolated as a brownish amorphous powder, gave a deep yellow color upon exposure to NH4OH vapor and a deep blue color with aqueous FeCl3 solution, suggesting an aromatic nature. The pseudo-molecular ion peaks at 1203.3038 [M+H]+ and 1201.2881 [M-H]- were found in the positive HRFAB and the negative HRFAB mass spectra, respectively, along with the 54 carbon signals in 13C NMR, indicated the molecular formula C54H58O31. The 13C NMR spectra, showed 54 carbons signals due to two carbonyls and 14 quaternary aromatic carbons substituted by an oxygen atom, four quaternary and ten tertiary aromatic carbons, in addition to 24 carbons assigned to sugars. The UV data in MeOH showed absorption maxima at λmax 257 (band II), 268 (sh), 297 (sh) and 360 nm (band I) and a broad hydroxyl absorption band at 3050 to 3400 cm-1 and chelated carbonyl bands at 1652 and 1697 cm-1 in the IR spectrum are characteristic of flavonol derivatives.15
The 1H NMR spectrum (in CD3OD) of 1 showed the presence of two quercetin skeletons based on the aromatic proton signals. Four aromatic signals each comprise four sets of meta-coupled doublets at δH 6.20, 6.38, 7.66 and 7.86. Signals at δH 6.20 (2H, d, J = 1.8 Hz) and δH 6.38 (2H, d, J = 1.8 Hz) were assigned to H-6 and 8 of ring A and H-6'' and 8' of ring A', respectively. The two sets of ABX doublets of rings B and B' at δH 7.66 (1H, d, J = 1.8) and δH 7.86 (1H, d, J = 1.8 Hz) were assigned to H-2' and 2''' of ring B and B', respectively. A signal at δH 6.86 (2H, d, J = 8.5 Hz) corresponded to H-5' of ring B and 5''' of ring B', while signals at δH 7.59 (1H, dd, J = 8.5, 1.8 Hz) and 7.62 (1H, dd, J = 8.5, 1.8 Hz) were assigned to H-6' and 6''' of ring B and B', respectively.
The observed proton and carbon resonances due to the sugar moieties were assigned based on the COSY, HMQC and HMBC experiments. In the COSY data, the presence of two rhamnopyranose moieties was suggested by the characteristic two methyl doublets at δH 1.18 (3H, d, J = 6.3) and 1.11 (3H, d, J = 6.3), the two anomeric protons at δH 4.51 (1H, d, J = 1.5) and 4.52 (1H, d, J = 1.5) and the eight other 1H resonances δH 3.84, (1H, d, J = 9.3), 3.80, (1H, d, J = 10.3), 3.59, (2H, br.s), 3.64 (1H, br.s), 3.63 (1H, br.s) 3.28 (2H, d, J = 3.4). Fourteen other 1H resonances were observed, with two anomeric protons at δH 5.11 (1H, d, J = 7.8) and 5.07 (1H, d, J = 7.8), and 12 proton signals at δH 3.58, 3.52, 3.48, 3.46, 3.43, 3.66, 3.65, 3.51 and 3.74 (2H, dd, J = 9.8, 5.4), suggesting the presence of another two other sugar moieties, which were identified as two glucopyranoses from the analysis of the 1H NMR spectroscopic data. From the HMBC, the anomeric proton signals H-1''''' and 1''''''' (δH 4.51, 4.52) of rhamnopyranoses coupled to C-6'''' (δC 67.43) as well as 6'''''' (δC 68.58) of glucopyranoses (Figure 2) were found. Evaluation of spin-spin couplings and chemical shifts (1H NMR, 13C NMR) allowed identification of two rutinoses in compound 1. The positions of the sugar linkages were confirmed by HMBC correlations at 3 and 3', respectively; thus, HMBC of compound 1 showed the anomeric proton signals H-1'''', 1'''''' (δH 5.07, 5.11) of glucopyranoses coupled to C-3 and 3'' (δC 135.64, 135.89) of quercetine moieties. A comparison of molecular weight and 13C NMR spectral data with those of different flavonoid glycosides led to the suggestion that compound 1 could be a dimer of two different, but related, flavonoid glycosides and that, this dimer can only be linked by an ether bond engaging one of the oxygen substituents.
Evidence of linkage position came from complete acetylation, which gave zizyflavoside A octadecaacetate (4, experimental section, C90H94O49). Compound 4 showed no residual IR hydroxyl absorption but did show the presence of 18 acetyl methyls (δ 1.75-2.30) in its 1H NMR and added 18 carbonyls to the 13C-NMR, further confirming complete acetylation. The carbons C4' (δC 150.00) and C4''' (δC 149.81) in zizyflavoside A (1), which was identified by HMBC (Figure 3) correlations, shifted farther upfield (δC 143.53 and δC 143.48) in zizyflavoside A octadecaacetate (4). Additionally, C3' (δC 145.77) and C3''' (δC 145.86) had similar resonances in 1 and 4, leading to an ether linkage between 3' and 3'''. Together, these observations suggest the phenyl ether type biflavonoid with the structure 1 [4',4''',5,5'',7,7'-hexahydroxy-3,3''-O-rutinosyl-3',3'''-biflavonoyl ether].
Although 1 has a symmetrical structure, each flavonoid moieties may take specific conformations in the space. Cage-shaped structure obtained with the aid of spacial software for molecular modeling (data not given) indicated different conformations for both two flavonoid moieties and two rutinose moieties, which had an influence on NMR resonance showing different chemical shifts. The positive FAB mass of 1 had significant peaks at m/z 749, 611, 465 and 303, in addition to retro Diels-Alder peaks (e.g., m/z 271 and m/z 153).16
After acid hydrolysis of zizyflavoside A (1), the sugars were isolated and identified as α-rhamnopyranose and β-glucopyranose by co-TLC with authentic markers. The stereochemistry of the sugars was identified by chiral HPLC of the benzoyl derivatives of acid hydrolysate of 1 (11 and 12).
The aglycone was subsequently identified as the new biflavonoyl ether aglycone 3 (see data in experimental section).
ZIZYFLAVOSIDE B
Zizyflavoside B (2) was isolated as greenish yellow crystals and gave the same chemical reactivity as zizyflavoside A (1), suggesting that compound 2 also had a flavonoid nature. The positive HRFAB
mass showed a quasi-molecular ion peak at m/z 911.1873 [M+H]+ corresponding to the molecular formula C42H38O23; this was corroborated by 13C NMR which showed 42 carbons (Table 2). The UV absorption in MeOH [λmax 257 (band II), 268 (sh), 297 (sh) and 359 nm (band I)]15 as well as the IR spectrum gave similar data to those obtained for 1. The 1H NMR and 13C NMR spectra showed the presence of two anomeric sugar proton signals at δH 5.14 (1H, d, J = 7.8) and δH 5.22 (1H, d, J = 7.8). The 13C NMR and 1H-1H-COSY allowed identification of two β-glucopyranosyls, and the remaining 13C NMR signals were similar to those of 1. Complete analysis of HMBC, HMQC and COSY suggested that 2 may be biflavonoyl ether dimer. The positive FAB mass showed significant peaks at m/z 587, 465 and 303.16
The structure was confirmed by acid hydrolysis of zizyflavoside B which afforded the same aglycone 3 of zizyflavoside A (1) and β-D-glucopyranose identified by co-TLC and chiral HPLC, as mentioned in the experimental section. As a result zizyflavoside B (2) was identified as [4',4''',5,5'',7,7'-hexahydroxy-3,3''-O-β-D-glucosyl-3',3'''-biflavonoyl ether].
BIOMIMETIC SYNTHESIS OF ZIZYFLAVOSIDE A
Oxidative coupling of phenols is involved in some biological reactions, for example, in formation of lignin or melanin, which are catalyzed by oxidoreductase enzymes such as peroxidase, oxidase, or oxygenase. Enzymatic oxidation of polyphenols is very important in biochemistry because the subsequent coupling reactions are involved in some biosynthetic pathways such as tannin and melanin formation. In the oxidative coupling of catechin by polyphenol oxidase, dimers compounds were formed. Laccase, one of the oxidase enzymes that oxidize substrates with a trinuclear copper active site. This enzyme plays an important role in the polymerization of lignin-related substrates in nature. The symmetrical structure of the phenyl ether type biflavonoid suggests the possibility of two biosynthetic processes. One is dehydrative ether formation of the same flavonoids, and the other is oxidative coupling of two different flavonoids.
We postulate that 1 and 2 could be formed through oxidative coupling reaction with enzyme such as laccase of the two different flavonoid glycosides. As the result, zizyflavoside A was synthesized from quercetin-3-O-rutinoside and kaempferol-3-O-rutinoside using the laccase with 8% yield. This enzymatic reaction indicated the biosynthesis of 1 takes place of radical pathway. The reaction mechanisms of the oxidative coupling selectivity of laccase have to date been studied by many researchers so far,17,18 based on those, we have proposed the following possible reaction mechanism for the C–O coupling selectivity as follows, coupling of free phenoxy radicals resulting from one-electron-oxidation of both quercetin-3-O-rutinoside and kaempferol-3-O-rutinoside (Scheme 1).
CONCLUSION
Eight flavonoids were isolated and characterized from Zizyphus spina-christi. Two were new phenyl ether type biflavonoids for which zizflavosides A (1) and B (2) had been assigned with spectroscopic and chemical data. The biomimetic synthesis of zizflavoside A (1) using laccase was done and this enzymatic reaction indicated the biosynthesis of 1 takes place of the radical pathways.
EXPERIMENTAL
GENERAL PROCEDURES
Melting points determined on Yanagimoto micro melting point apparatus and uncorrected. Optical rotations determined with a Horiba SEPA-3000 high-sensitivity polarimeter. UV spectra measured on a Shimadzu UV-1600 UV–Vis spectrometer, whether the IR spectra recorded using KBr disks on a Shimadzu IR-460 IR spectrophotometer. NMR spectra obtained using a JEOL GSX-500 spectrometer.
Chemical shifts referenced to the residual solvent peaks CD3OD (δH 3.30 and δC 49.8) and CDCl3 (δH 7.26 and δC 77.0). Mass spectra measured on a JEOL SX-102 mass spectrometer. Sephadex LH-20 (25–100 μm, Sigma), Silica gel (63–210 μm, Kanto Chemical) and ODS (63–212 μm, Wako) were used for open column chromatography (cc). Further purification performed by HPLC equipped with a reversed-phase column (column: Sun- FireTM Prep C18 5 µm; 10×250 mm; Waters). TLC utilized silica gel 60 F254 (Merck) and RP-18 F254S (Merck).
PLANT AND ENZYME MATERIALS
For the present study, 1.7 kg of leaves of Zizyphus spina-christi (L.) Willd. were collected in April, 2006 from plants identified by Professor Mo'men Mostafa Zareh, (Department of Botany, Assuit University, Egypt) and cultivated in the small village Azbt Abohomodi, Qena, Egypt. A voucher specimen was kept at the Herbarium of Al-Azhar University Faculty of Pharmacy, Assuit branch, Egypt, and at the laboratory of Pharmacognosy and Chemistry of Natural Products (T-205), Graduate School of Natural Science and Technology, Kanazawa University, Japan. Laccase enzyme (EC 1.10.3.2) from Pycnoporus cinnabarinus was purchased from (JenaBios GmbH, Jena/Germany).
EXTRACTION AND ISOLATION
The MeOH extract of the air-dried fine powder of leaves (1.7 kg) dissolved in 50% aqueous MeOH and successfully fractionated with n-hexane, CHCl3 and EtOAc. The dried aqueous MeOH extract (100 g) was subjected to CC on silica gel and eluted with a mixture of CHCl3/MeOH of increasing polarity (9:1, 8:2, 7:3, 6:4, 5:5, 4:6, 3:7 and 2:8). The fraction eluted with CHCl3/ MeOH (6:4) purified by HPLC (ODS, H2O/MeOH 6:4) to afforded kaempferol-3-O-rutinoside (5, 3 mg) and quercetin-3-O-rutinoside (6, 25 mg), while the fraction eluted by CHCl3/MeOH (8:2) was subjected to Sephadex LH 20 with H2O/MeOH of increasing polarity as the eluent ( 9:1, 8:2, 7:3, 6:4, 5:5, 4:6, 3:7 and 2:8). The sub fraction eluted with H2O/MeOH (4:6) was purified by HPLC octadecyl silane (ODS; H2O/MeOH 6:4) to provide 3',5'-di-C-β-D-glucosylphloretine (7, 10 mg), zizyflavoside A (1, 20 mg) and quercetin-3-O-[β-D-xylosyl-(1→2)-α-L-rhamnoside]-4'-O-α-L-rhamnoside (8, 7 mg). The EtOAc fraction (100 g) was subjected to reverse phase CC on ODS with aqueous MeOH (9:1, 8:2, 7:3, 6:4, 5:5, 4:6, 3:7 and 2:8) as the eluent. The fraction eluted with H2O/MeOH (8:2) was purified by HPLC (ODS, H2O/MeOH 8:2) to provide epiafzelechin (9, 10 mg). The fraction eluted with H2O/MeOH (6:4) was purified by HPLC to provide zizyflavoside B (2, 14 mg) along with quercetin-3-O-[β-D-xylosyl-(1→2)-α-L-rhamnoside] (10, 10 mg). The known compounds (5-10) were identified by comparison of their spectral data with those reported in the literatures.12-14,19,20
Zizyflavoside A (1, C54H58O31). Brownish yellow amorphous powder. [α]D30 -80.43° (c 1.8, MeOH); UV (MeOH) λmax nm (log ε) 207 (8.3), 257 (8.0), 268 (sh) (7.9), 297 (sh) (7.6) and 360 (7.9) nm; IR (KBr) vmax 3733, 3647, 3566, 3321, 2387, 2314, 1749, 1616, 1506, 1218, 1047 cm-1; 1H NMR (500 MHz, MeOH-d4, 25 °C, TMS) and 13C NMR (125 MHz, MeOH-d4) shown in Table 1; FABMS m/z (rel. int.) 749 (2) [M+H-146-146-162]+, 611 (3) [M+H-146-162-285]+, 465 (5) [M+H-593-146]+, 303 (88) [M+H-146-162-593 ]+; HRFABMS m/z 1203.3038 [M+H]+ (calcd for C54H59O31, 1203.3040) and m/z 1201.2881 [M-H]- (calcd for C54H57O31, 1201.2883).
Zizyflavoside B (2, C42H38O23). Greenish yellow crystals, mp 205-206 °C. [α]D30 -70.9° (c 0.33, MeOH); UV (MeOH) λmax nm (log ε) 208 (5.8), 257 (5.5), 268 (5.4) (sh), 297 (5.1) (sh) and 359 (5.4) nm; IR (KBr disk) vmax 3733, 3647, 3566, 3278, 3219, 2360, 1749, 1616, 1506, 1218, 1047 cm-1; 1H NMR (500 MHz, MeOH-d4, 25 °C, TMS) and 13C NMR (125 MHz, MeOH-d4) shown in Table 2; FABMS m/z (rel. int.) 587 (2) [M+H-162-162]+, 465 (5) [M+H-162-284]+, 303 (88) [M+H-162-162-284]+; HRFABMS m/z 911.1873[M+1]+ (calcd for C42H39O23, 911.1881).
ACETYLATION OF ZIZYFLAVOSIDE A
Zizyflavoside A (5.9 mg) was dissolved in a mixture of Ac2O (0.75 mL) and dry pyridine (0.75 mL) in a 5 mL round-bottom flask. The well-sealed flask was left in an oven at 60 °C for 4.5 hours, and the solution was then evaporated to dryness under vacuum. The resultant brown residue was purified by CC on a Sephadex LH-20 support with MeOH as the eluent to give zizyflavoside A octadecaacetate (4, 9.5 mg, yield 98.7%) as a yellowish powder.
Zizyflavoside A octadecaacetate (4, C90H94O49). Brownish green amorphous powder. IR (KBr disk) vmax cm-1 2324, 1749, 1616, 1545, 1218, 1047; 1H NMR (500 MHz, MeOH-d4) δ 7.92 (2H, d, J = 8.5, 1.8 Hz, H-6', 6'''), 7.87 (2H, d, J = 1.8 Hz, H-2', 2'''), 7.29 (1H, d, J = 8.5 Hz, H-5'), 7.27 (1H, d, J = 8.5 Hz, H-5'''), 6.87 (1H, d, J = 1.8 Hz, H-8), 6.70 (1H, d, J = 1.8 Hz, H-8'), 6.69 (1H, d, J = 1.8 Hz, H-6), 6.42 (1H, d, J = 1.8 Hz, H-6''), 5.53 (1H, d, J = 7.9 Hz, glucose 1, H-1''''), 5.29 (1H, d, J = 7.9 Hz, glucose 2, H-1''''''), 4.44 (2H, d, J = 1.8 Hz, rhamnose1 and 2, H-1''''' and H-1'''''''), 5.24-3.25 (20H of sugar residue), 2.30-1.75 (54H of 18 CH3, OCOCH3), 0.88 (3H, d, J= 6.1, CH3 of rhamnose 1), 0.78 (3H, d, J = 6.1, CH3 of rhamnose 2); 13C NMR (125 MHz, MeOH-d4) δ158.16, 158.10 (C-2, 2''), 137.81, 137.68 (C-3, 3''), 173.70, 173.68 (C-4, 4'') 151.96, 151.38 (C-5, 5''), 110.61, 110.54 (C-6, 6''), 158.16, 158.10 (C-7, 7''), 101.25, 101.20 (C-8, 8'), 155.98, 155.95 (C-9, 9'), 115.08, 115.04 (C-10, 10'), 130.29 (C-1'), 129.60 (C-1'''), 125.96, 125.93 (C-2', 2'''), 145.77, 145.75 (C-3', 3'''), 143.53, 143.48 (C-4', 4'''), 128.62, 128.44 (C-5', 5'''), 128.28 (C-6'), 126.11 (C-6'''), 101.15, 99.09 (2 anomeric glucose carbons), 99.05, 98.94 (2 anomeric rhamnose carbons), 74.14, 74.08, 73.20, 73.17, 73.04, 72.20, 72.15, 71.88, 71.79, 70.88, 70.85, 70.70, 70.67, 70.21, 69.20, 69.15, 67.65, 67, 58, 67.43, 67.38 (20 carbons of sugar residue), 172.17, 172.15, 171.99, 171.94, 171.84, 171.78, 171.65, 171.48, 171.42, 171.23, 170.91, 170.88, 169.88, 169.86, 169,78, 169.69, 169.55, 169.51 (18C=O of OCOCH3), 21.38-20.47 (18CH3 of OCOCH3), 17.56, 17.36 (2CH3 of two rhamnoses); FABMS m/z (ret. int.) 1914 [M-H-43]-, 1795 [M-H-43-2x60]ˉ.
ACID HYDROLYSIS OF ZIZYFLAVOSIDES A AND B
Each of the phenyl ether type biflavonoids 1 and 2 (each 5 mg) was refluxed in 4 N HC1 (5 mL) for 2 hours. The resulting aglycones were extracted with EtOAc and purified to give the same aglycone 3. TLC [silica-gel, CHCl3-MeOH (9:1)] aglycone 3 Rf 0.36 and quercetine Rf 0.41. The aqueous layer was concentrated and checked by TLC with authentic markers [silica gel, CHCl3-MeOH-H2O (65:40:10)] D-glucose Rf 0.40 and L-rhamnose Rf 0.62.
Aglycone (3, C30H18O13). Yellowish green amorphous powder. IR (KBr disk) vmax 3400, 3210, 1769, 1620, 1218, 1047 cm-1; 1H NMR (500 MHz, MeOH-d4) δ 7.72 (2H, d, J = 1.8 Hz, H-2', 2'''), 7.62 (2H, dd, J = 8.5, 1.8 Hz, H-6', 6'''), 6.87 (2H, d, J = 8.5 Hz, H-5', 5'''), 6.38 (2H, d, J = 1.8 Hz, H-8, 8'), 6.17 (2H, d, J = 1.8 Hz, H-6,6''); 13C NMR (125 MHz, MeOH-d4) δ146.2 (C-2, 2''), 135.3 (C-3, 3''), 177.3 (C-4, 4'') 162.5 (C-5, 5''), 99.2 (C-6, 6''), 165.5 (C-7, 7'), 94.4 (C-8, 8'), 159.2 (C-9, 9'), 104.5 (C-10, 10'), 121.6 (C-1'), 124.1(C-1'''), 116.0 (C-2', 2'''), 146.2 (C-3', 3'''), 148.7 (C-4'), 150.1 (C-4'''), 116.2 (C-5', 5'''), 128.7 (C-6'), 130.9 (C-6'''); FABMS m/z (rel. int.) 585 (5.1) [M-H]ˉ, 301 (9.5) [M-H-284]ˉ; HRFABMS m/z 585.06691 [M-H]ˉ (calcd for C30H17O13, 585.06693).
L-Rhamnopyranose tetrabenzoate and D-glucopyranose tetrabenzoate
Benzoyl chloride (0.5 mL) was added to each ice-cooled solution of either D-glucopyranose (25.0 mg) or L-rhamonopyranose (18.0 mg) in dry pyridine (1.0 mL). And each mixture stirred at room temperature for 15 h. MeOH (1.0 mL) added dropwise to the reaction mixture, stirred for 30 min, and then diluted with EtOAc and aqueous Na2CO3, and the layers separated. Each organic layer washed with brine, and the combined aqueous layers for each extracted with EtOAc. Each combined organic extracts dried over MgSO4, and concentrated. The corresponding dark brown oil residue individually purified by silica gel cc (eluting with hexane/EtOAc 5:1) to give either 11 (55 mg) or 12 (45 mg) as a colorless oil and white flakes respectively.
D-Glucopyranose pentabenzoate (11). 1H NMR (500 MHz, CDCl3) 4.41 (1H, dd, J = 13.1, 5.2 Hz), 4.54 (1H, s,), 4.56 (1H, t, J = 2.4 Hz), 5.61 (1H, dd, J = 10.1, 4.0 Hz), 5.79 (1H, t, J = 10.1 Hz), 6.25 (1H, t, J = 9.8 Hz), 6.78 (1H, d, J = 4.3 Hz), 7.23–8.09 (25H, m).
L-Rhamnopyranose tetrabenzoate (12). 1H NMR (500 MHz, CDCl3) δ 1.44 (3H, d, J = 6.4 Hz), 4.37 (1H, dd, J = 6.4, 9.8 Hz), 5.81 (1H, t, J = 9.8 Hz), 5.88 (1H, dd, J = 1.9, 3.4 Hz), 6.00 (1H, dd, J = 3.4, 10.3 Hz), 6.57 (1H, d, J = 1.5 Hz), 7.27–8.21 (20H, m); 13C NMR (125 MHz, CDCl3) δ17.7, 69.3, 69.7, 69.8, 71.1, 91.3, 128.3, 128.4, 128.6, 128.7, 128.8, 128.8, 129.0, 129.7, 129.7, 130.0, 130.1, 130.1, 133.3, 133.4, 133.6, 133.9, 164.0, 165.3, 165.6, 165.7.
DETERMINATION OF STEREOCHEMISTRY OF THE SECONDAY SUGAR UNIT IN 1 AND 2 BY CHIRAL HPLC
Benzoyl chloride (0.1 mL) added to the ice-cooled solution of each sugar parts of 1 and 2 in dry pyridine (0.5 mL), and each mixture stirred at room temperature for 35 h. MeOH (0.1 mL) added dropwise to each of the reaction mixtures, stirred for 30 min, and then diluted with EtOAc and aqueous Na2CO3, and the layers were separated. Each organic layer washed with brine, and the combined aqueous layers extracted with EtOAc. Each of the combined organic extracts dried over MgSO4, and concentrated. Each dark brown oil residue purified by silica gel cc (eluting with hexane/EtOAc 5:1) to give the corresponding benzoyl derivatives of the sugar. The benzoyl derivatives of each hydrolysis products of 1 or 2 subjected to chiral HPLC analyzes using CHIRALPAK IB (Daisel Chemical Industries, 4.6×250 mm; MeOH, 0.75 mL/min; UV detection at 254 nm). The retention time of each of the benzoyl derivatives of the hydrolysis products of 1 or 2 found to be 6.48 and 9.10 min, respectively. The retention times of synthetic benzoyl derivatives of L-rhamnopyranose (12) and that of D-glucopyranose (11) were 6.48 and 9.10 min, respectively.
SYNTHESIS OF ZIZYFLAVOSIDE A USING LACCASE (EC 1.10.3.2)
In a 30 mL flask, quercetin-3-O-rutinoside (3.99 mg, 0.0065 mmoles) and kaempferol-3-O-rutinoside (3.89 mg, 0.0065 mmoles) were dissolved in a mixture of MeOH (3 mL) and distilled water (1 mL). Laccase solution (3 mL, 0.128 units) was added to the reaction mixture. The flask was incubated for 15 min at 24 ºC, and MeOH was then rapidly evaporated. The remaining mixture containing water was freeze-dried and subjected to HPLC purification to obtain zizyflavoside A (1'), 0.65 mg (8% yield) with other products (two unidentified polymers and another unidentified dimer).
Synthetic zizyflavoside A (1'). Yellowish white amorphous residue. IR (KBr) vmax 3733, 3647, 3566, 3321, 2387, 2314, 1749, 1616, 1506, 1218, 1047 cm-1; 1H NMR (600 MHz, MeOH-d4, 25 °C, TMS) δ 7.85 (1H, d, J = 2.1 Hz, H-2'), 7.57 (1H, d, J = 2.1 Hz, H-2'''), 7.54 (2H, dd, J = 8.5, 2.1 Hz, H-6', 6'''), 6.83 (2H, d, J = 8.5 Hz, H-5', 5'''), 6.33 (2H, d, J = 2.1 Hz, H-8, 8'), 6.12 (2H, d, J = 2.1 Hz, H-6, 6''), 5.15 (2H, d, J = 7.6 Hz) 3.72 (2H, m), 3.65 (1H, brs), 3.54 (1H, d, J = 3.4), 3.51(1H, brs), 3.46 (1H, brs), 3.52 (1H, d, J = 3.0), 3.48 (1H, d, J = 3.0), 3.43 (1H, m), 3.37 (2H, m), 3.32 (1H, brs) (12H, glucoses residue), 4.43 (2H, d, J = 1.4 Hz), 3.66 (1H, brs), 3.65 (1H, brs), 3.63 (1H, d, J = 3.4), 3.58 (2H, brs), 3.52 (1H, d, J = 2.9), 3.26 (1H, t, J = 3.4), 3.25 (10H, rhamnoses residue), 1.11 (3H, d, J = 5.5, CH3 of rhamnose 1), 1.09 (3H, d, J = 5.5, CH3 of rhamnose 1); FABMS m/z (ret. int.) 747 (0.5) [M+H-146-146-162]+, 611 (1) [M+H-146-162-285]+, 465 (0.5) [M+H-593-146]+, 303 (3) [M+H-146-162-593]+; HRFABMS m/z 1203.3058 [M+H]+ (calcd for C54H59O31, 1203.3040).
References
1. V. Takeckholm, ‘Students’ Flora of Egypt, Cairo University, Cairo, Egypt. 1974, p. 345.
2. J. A. Duke, ‘Handbook of Medicinal Herbs’, CRC Press: Florida, 1985, p. 516.
3. D. L Nesseem, C. G. Michel, A. A. Sleem, and T. S. El-Alfy, Pharmazie, 2009, 64, 104.
4. A. O. Abdel-Zaher, S. Y. Salim, M. H. Assaf, and R. H. Abdel-Hady, J. Ethnopharmacol., 2005, 101, 129. CrossRef
5. B. Adzu, S. Amos, M. B. Amizan, and K. Gamaniel, Acta Trop., 2003, 87, 245. CrossRef
6. N. S. Alzoreky and K. Nakahara, Int. J. Food Microbiol., 2003, 80, 223. CrossRef
7. B. Adzu, S. Amos, C. Wambebe, and K. Gamaniel, Fitoterapia, 2001, 72, 4, 344.
8. O. M. Musbah, O. M. Tanira , A. M. Ageel , M. Tariq , A. Mohsin, and A. H. Shah, Pharm. Biol., 1988, 26, 1, 56.
9. M. Ikram, Y. Ogihara, and K. Yamasaki, J. Nat. Prod., 1981, 44, 91. CrossRef
10. F. M. Abdel-galil and M. A. El-Jissry, Phytochemistry, 1991, 30, 1348. CrossRef
11. G. H. Mahran, K. W. Glombitza, Y. W. Mirhom, R. Hartmann, and C. G. Michel, Planta Med., 1996, 62, 163. CrossRef
12. M. A. M. Nawwar, M. S. Ishak, H. N. Michael, and J. Buddrus, Phytochemistry, 1984, 23, 2110. CrossRef
13. A. A. Shahat, L. Pieters, S. Apers, N. M. Nazeif, N. S. Abdel-Azim, D. V. Berghe, and A. J. Vlietinck, Phytother. Res., 2001, 15, 593. CrossRef
14. A. M. Pawlowska, F. Camangi, A. Bader, and A. Braca, Food Chem., 2009, 112, 858. CrossRef
15. T. Mabry, K. P. Markham, and M. B. Thomas, ' The Systematic Identification of Flavonoids, Springer Verlag, New York, 1970, pp. 40-42.
16. J. B. Harborne, 'The Flavonoids Advances in Research since 1986, 1st ed. Chapman and Hall, London, 1994, p. 99.
17. P. J. Baesjou, W. L. Driessen, G. Challa, and J. Reedijk, J. Am. Chem. Soc., 1997, 119, 12590. CrossRef
18. K. Kataoka, R. Sugiyama, S. Hirota, M. Inoue, K. Urata, Y. Minagawa, D. Seo, and T. Sakurai, J. Biol. Chem., 2009, 284, 14405. CrossRef
19. S. B. Wan and T. H. Chan, Tetrahedron, 2004, 60, 8207. CrossRef
20. K. Ogawa, A. Kawasaki, M. Omuro, T. Yoshida, Y. Ikoma, and M. Yano, Phytochemistry, 2001, 57, 737. CrossRef