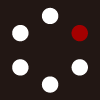
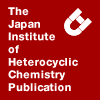
HETEROCYCLES
An International Journal for Reviews and Communications in Heterocyclic ChemistryWeb Edition ISSN: 1881-0942
Published online by The Japan Institute of Heterocyclic Chemistry
e-Journal
Full Text HTML
Received, 12th July, 2010, Accepted, 24th August, 2010, Published online, 25th August, 2010.
DOI: 10.3987/COM-10-12016
■ Diastereoselective Reduction of Chiral N-Tosyl-2-benzoyl-1,3-oxazine Derived from (1R)-(+)-Camphor
Kwang-Youn Ko* and Hoseop Yun
Department of Chemistry, Ajou University, 5 Wonchun-Dong, Youngtong-Gu, Suwon 443-749, Korea
Abstract
The stereochemistry of reduction of chiral N-tosyl-2-benzoyl-1,3-oxazine prepared by condensation of 1,3-amino alcohol derived from (1R)-(+)-camphor with phenylglyoxal was investigated using various reducing agents. Based on X-ray crystallography, 2-benzoyl group in 1,3-oxazine ring was situated in the axial position. High diastereoselectivity observed in the hydride reduction can be explained by a chelate model where N-tosyl oxygen atom takes part in chelation rather than ring oxygen atom.The diastereoselective addition of nucleophilic reagents to ketones having a chiral auxiliary is a useful method for obtaining optically active alcohols.1 Chiral auxiliaries used for this purpose include enantiomerically pure amino alcohols derived from norephedrine,2 (+)-pulegone3 or amino acids,4 3-hydroxythiols derived from (+)-pulegone,5 (-)-myrtenal6 or (1R)-(+)-camphor,7 and 1,3-diols.8 We previously reported highly diastereoselective reduction of chiral N-tosyl-1,3-oxazines 1 derived from D-glucose.9 In this case, the high diastereoselectivity observed in the reduction with chelating reducing agents such as L-Selectride® (lithium tri-sec-butylborohydride) has been explained by invoking a Cram’s chelate model, where the ring oxygen and the carbonyl oxygen take part in the chelation with metal cation. On the contrary, non-chelating agents such as diisobutylaluminum hydride (DIBAL-H) and n-Bu4NBH4 have been suggested to react according to a Felkin-Anh model.9
(1R)-(+)-Camphor has been used as a starting material for the preparation of various chiral auxiliaries or chiral catalysts.10 Chiral auxiliaries derived from camphor are known to have good crystallinity, giving some advantages in purification of product. However, 3-amino alcohols 2 which can be easily derived from camphor have not yet been used as chiral auxiliary for asymmetric reduction. As an extension of our previous work,9 we wish to report a highly diastereoselective reduction of camphor-based oxazine and determination of absolute configuration of newly formed stereocenter by single crystal X-ray diffraction.
N-Tosylamino alcohol 3, a camphor-derived chiral auxiliary used for the present study was prepared according to Scheme 1. Thus, ketopinic acid derived from (+)-camphor was converted to known amino alcohol 2.11 Subsequent tosylation with p-TsCl gave 3 as a crystalline solid. Finally, condensation with phenylglyoxal in acidic condition gave a mixture of two phenyl ketones 4 in a ratio of 4 : 1, as judged by 1H-NMR spectrum. Major product could be isolated in a pure form by recrystallization from EtOH.
Then, we studied reduction of phenyl ketone 4a using several reducing agents, as shown in Table 1 (Scheme 2).
Diastereoselectivity could be easily determined by 1H-NMR spectroscopy. For example, in entry 1, C-2 proton of the major product appeared as a doublet at δ 5.40 (J = 3.7 Hz) and that of the minor one appeared as a doublet at δ 5.54 (J = 9.1 Hz).
Either alcohol (R)-5 or (S)-5 (see below for the determination of the absolute configuration) was obtained as the major product, depending on the nature of reducing agents and solvents. Generally, all the chelating reducing agents used in the present case gave the (R)-5 as the major product (entries 1~7). Nonchelating agents such as DIBAL-H and n-Bu4NBH4 also gave the (R)-isomer albeit in rather poor selectivity, except for the entry 8b. High selectivity (>98%) favoring the (R)-alcohol was observed when LiAlH4, L-Selectride® or LiAlH(O-t-Bu)3 was used as reducing agents (entries 3b, 4a, 5b).
Next, we undertook the cleavage of oxazines 5 to determine the absolute configuration of the newly formed carbinol carbon and thereby the approaching preference of the nucleophile to the carbonyl faces: alcohol 5 obtained from L-Selectride® reduction (entry 4b) was converted to diol 6, [α]D20 -35.5 (c 1.01, EtOH) using acidic hydrolysis followed by NaBH3CN reduction of the resulting 2-hydroxyaldehyde intermediate (Scheme 3).12
Since (R)-6 is known to be levorotatory ([α]D20 -39.7 (c 4.33, EtOH)),13 we can conclude that the carbinol carbon has (R)-configuration. The formation of (R)-isomer can be explained by invoking a similar chelate model as in the case of ketone 1 where ring oxygen atom takes part in chelation, assuming that benzoyl group is situated equatorially.9 However, chemical shift for C-2 proton of major phenyl ketone 4 appeared downfield relative to that in the minor one (6.52 vs. 4.94 ppm), which may imply that benzoyl group is situated in an axial position rather than in an equatorial one.14 To unambiguously determine the absolute configuration of C-2 on 4a and carbinol carbon of 5, we resorted to single crystal X-ray crystallography of 4a and 5.15 Figure 1 and 2 show an ORTEP drawing of ketone 4a16 and the major product 517 of LiAlH4 reduction, respectively. Figure 1 clearly shows the axial orientation of the benzoyl group in 4a,18 as suspected based on the 1H NMR data. The oxazine ring has a chair conformation, which is in contrast to the boat conformation of the similar N-tosyl-2-vinyl-1,3-oxazine ring.19 Figure 2 shows the (R)-configuration of carbinol carbon, which agrees with the optical rotation data.
We can consider three conformations I~III that may result in the (R)-configuration, as shown in Scheme 4.1
Felkin-Anh polar model I where N-tosyl group is located perpendicular to the carbonyl group, appears to be unfavorable due to the steric reason. Another Felkin-Anh model II and the chelate model III can predict the correct stereochemistry. Model II is in accord with the X-ray structure of ketone 4, which shows that the carbonyl group, situated inside the oxazine ring is nearly perpendicular to the α-C-O bond.20 The chelate model III where the sulfonyl oxygen, not the ring oxygen as in model IV takes part in chelation can also explain the formation of (R)-alcohol.21
To see the effect of crown ether on the chelation, we performed the reduction in the presence of crown ethers.9 Little decrease in selectivity in the presence of crown ether was observed in the LiAlH4 and L-Selectride® reductions (entries 3a, 3b, 4b). This fact may be explained by assuming that Felkin-Anh model II is operating or chelation in model III is rather strong as not to be disturbed by crown ether. However, decrease in selectivity in THF which is a better solvating solvent than ether (entry 3a vs. 3b, 5a vs. 5b, 6a vs. 6b, and 7a vs. 7b) may support that the chelate model is more important. In contrast, in the case of LiEt3BH and NaEt3BH reduction the presence of crown ether dramatically affected the degree and/or direction of selectivity. For example, the diastereoselectivity of LiEt3BH reduction in toluene changed from 70% de to 30% in the presence of 12-crown-4 (entry 6c). Surprisingly, the use of 15-crown-5, which is known to complex with sodium cation more efficiently than lithium cation resulted in the opposite stereochemistry (entry 6c).22 Similar trend was also observed in the NaEt3BH reduction (entry 7). Compared with the LiEt3BH reduction the selectivity in the NaEt3BH reduction was better, decreasing in a larger extent in the presence of crown ether. This result is expected in that sodium chelate is less tight than lithium chelate, thus being disturbed more easily by crown ether. However, the reason for the better selectivity of less tight sodium chelate is not clear. In DIBAL-H reduction, the stereochemistry of reduction was moderate, except for the reduction in ether solvent, which gave the opposite stereochemistry to LiAlH4 reduction. This formation of (S)-carbinol can be explained by invoking the dipolar model V where the ring oxygen atom is anti to the carbonyl group. However, the reason why ether solvent behaves differently in the DIBAL-H reduction remains to be answered.
In summary, axial phenyl ketone 4a derived from a new camphor-based chiral auxiliary 3 was found to be reduced with chelating reducing agents in high diastereoselectivity. In the present case, the stereochemistry of this reduction could be explained by invoking a chelate model III where tosyl oxygen takes part in chelation. Participation of tosyl oxygen atom in chelation is in sharp contrast with the case of ketone 1 where ring oxygen atom takes part in chelation. This study shows that ketones 1 and 4a which are similar in structures can react with reducing agents in different stereochemical sense.
EXPERIMENTAL
IR spectra were recorded with a Nicolet Magna FT-IR spectrophotometer 550. NMR spectra were recorded on Varian Gemini 400 spectrometer in CDCl3 with tetramethylsilane (TMS) as internal standard. Melting points were determined on a Buchi and are uncorrected. Optical rotations were measured on a Jasco DIP-370 digital polarimeter. Elemental analyses were performed by Eager 200 CHNS CE Instrument. Liquid Column chromatography was performed over silica gel (Merck, silica gel 60, 70-230 mesh). Analytical TLC was performed on precoated Merck glass plates (silica gel 60 F254) and compounds were visualized under UV light or by dipping in an ethanol solution of phosphomolybdic acid, followed by heating. Dry THF was distilled over sodium benzophenone ketyl under nitrogen atmosphere.
N-Tosyl amino alcohol 3
To a well stirred mixture of amine 2 (1.80 g, 10.6 mmol) in EtOAc (35 mL) and water (20 mL) was added K2CO3 ( 1.47 g, 10.6 mmol) followed by p-TsCl ( 2.20 g, 11. 7 mmol) at 0 oC. The reaction mixture was stirred at 0 oC for 30 min and then at rt for 10 h. Then, the organic layer was separated and the aqueous layer was extracted with EtOAc (2 x 20 mL). The combined organic extracts were washed successively with brine (2 x 10 mL), dried over anhydrous Na2SO4 and concentrated in vacuo to give a crude yellowish product (3.30 g). Trituration in EtOAc (10 mL) gave 3 (2.00 g, 60%) as a white solid. 3: mp 195-197 oC; [α]D20 -4.3 (c 1.00, CHCl3); IR (KBr) νmax 3445 (OH), 3225, 2956, 1475, 1321, 1160; 1H-NMR (400 MHz, CDCl3): δ 7.77 (2H, d, J = 8.3 Hz), 7.32 (2H, d, J = 8.0 Hz), 5.29 (1H, s, J = 8.0 Hz, NH), 4.04, 3.46 (2H, ABq, J = 12 Hz), 3.22 (1H, dd, J = 10, 4.6 Hz), 2.44 (s, 3H), 1.68-1.52 (5H, m), 1.35-1.20 (2H, m), 1.00 (3H, s), 0.88 (3H, s); 13C-NMR (100 MHz, CDCl3): δ 143.5, 136.6, 129.8, 129.2, 61.7, 59.2, 53.3, 47.0, 46.0, 39.5, 32.0, 26.4, 21.5, 21.0, 20.6; Anal. Calcd for C11H25NO3S: C, 63.12; H, 7.81; N, 4.33; S, 9.91. Found: C, 62.51; H, 7.90; N, 4,29; S, 9.54.
Phenyl ketone 4a
A solution of 3 (970 mg, 3.00 mmol) and phenylglyoxal hydrate (790 mg, 5.89 mmol) in benzene (50 mL) was refluxed for 20 h in the presence of p-TsOH (75 mg). The reaction mixture was diluted with EtOAc (50 mL) and water (20 mL). The organic layer was separated and washed successively with brine (2 x 10 mL), dried over anhydrous Na2SO4 and concentrated in vacuo to give a crude residue, which was purified by recrystallization from EtOH to afford 4a (660 mg, 50%). 4a: mp 181-182 oC; [α]D20 -65.1 (c 0.98, CHCl3); IR (KBr) νmax 3447 (OH), 3225, 1640 (C=O), 1329, 1160, 715; 1H-NMR (400 MHz, CDCl3): δ 8.14 (2H, d, J = 8.1 Hz), 7.73 (2H, d, J = 8.1 Hz), 7.65-7.45 (3H, m), 7.31 (2H, s), 6.52 (1H, s), 4.16 (1H, dd, J = 8.1, 4.6 Hz), 3.78, 3.38 (2H, ABq, J = 13 Hz), 2.44 (s, 3H), 2.25-2.13 (1H, m), 1.78-1.62 (4H, m), 1.40-1.09 (2H, m), 0.98 (3H, s), 0.83 (3H, s); 13C-NMR (100 MHz, CDCl3): δ 195.2, 143.2, 139.6, 134.1, 133.8, 129.3, 129.2, 128.6, 127.3, 84.9, 63.5, 59.4, 47.2, 46.5, 45.5, 35.6, 30.9, 26.6, 21.6, 21.4, 20.2; Anal. Calcd for C25H29NO4S: C, 68.23; H, 6.66; N, 3.19; S, 7.29. Found: C, 68.16; H, 6.68; N, 3.16; S, 6.91.
Reduction of phenyl ketone 4a
An example: A solution of 4a (0.50 g, 1.18 mmol) in dry ether (20 mL) was treated with a solution (2.0 mL) of 1 M LiAlH4 in ether under nitrogen atmosphere at -70 oC. After stirring for 1 h, the reaction mixture was quenched with NH4Cl aq. and extracted with EtOAc (20 mL). The organic layer was separated and washed successively with brine (2 x 10 mL), dried over anhydrous Na2SO4 and concentrated in vacuo to give a crude residue 5 (0.45 g, 90%), whose 1H-NMR spectrum showed that the diastereoselective excess was 98%. Recrystallization from EtOH gave 5 as a single diastereomer. 5: mp 172-173 oC; [α]D20 -93.1 (c 1.02, CHCl3); IR (KBr) νmax 3487 (OH), 1160, 1057, 975; 1H-NMR (400 MHz, CDCl3): δ 7.71 (2H, d, J = 8.3 Hz), 7.51-7.23 (7H, m), 5.66 (1H, d, J = 3.7 Hz), 5.40 (1H, d, J = 3.7 Hz), 4.40, 3.61 (2H, ABq, J = 12 Hz), 4.15 (1H, dd, J = 8.1, 5.1 Hz), 2.40 (s, 3H), 2.08-1.98 (2H, m), 1.70-1.08 (5H, m), 0.77 (3H, s), 0.76 (3H, s); 13C-NMR (100 MHz, CDCl3): δ 143.2, 140.2, 140.1, 129.4, 128.3, 127.6, 127.0, 125.9, 86.0, 79.0, 62.8, 60.3, 46.9 46.3, 45.2, 35.8, 31.7, 26.5, 21.5, 20.7, 20.3.
1-Phenyl-1,2-ethanediol (6)
A solution of 5 (0.40 g, 0.91 mmol, derived from L-Selectride® reduction (entry 4b)) in MeOH (50 mL) was treated with 5% HCl aq. (10 mL) and the whole mixture was refluxed for 3 h. After adjusting pH to 4~5 by adding Na2CO3, the reaction mixture was treated with NaBH3CN (60 mg, 0.95 mmol) and refluxed for 30 min. Concentration in vacuo gave a yellow solid, which upon column chromatography (hexanes:EtOAc = 2:1) yielded 6 (0.10 g, 79%) and 3 (0.25 g, 85%). Kugelrohr distillation (145~150 oC, 0.1 mmHg) of crude 6 gave a pure known diol 6. 6: [α]D20 -35.5 (c 1.01, EtOH) [lit.13 for (R)-isomer [α]D20 -39.7 (c 4.33, EtOH)]; 1H-NMR (400 MHz, CDCl3): δ 7.36 (5H, s), 4.80 (1H, X part of ABX, HOCH2CH-, J = 8.0, 3.5 Hz), 3.74, 3.65 (2H, AB part of ABX, HOCH2CH-, J = 11.4, 8.0, 3.5 Hz), 3.11-2.20 (2H, s).
ACKNOWLEDGEMENTS
The authors are grateful to Ajou University for use of its X-ray facilities. One of the authors (K-Y. Ko) thanks Ajou University for providing a sabbatical leave during the year 2009.
References
1. (a) A. Mengel and O. Reiser, Chem. Rev., 1999, 99, 1191; CrossRef (b) J. Roberstson, W. P. Unsworth, and S. G. Lamont, Tetrahedron, 2010, 66, 2363. CrossRef
2. (a) L. Colombo, M. Di Giacomo, G. Brusotti, and E. Milano, Tetrahedron Lett., 1995, 36, 2863; CrossRef (b) T. Harder, T. Lohl, M. Bolte, K. Wagner, and D. Hoppe, Tetrahedron Lett., 1994, 35, 7365. CrossRef
3. E. L. Eliel and X.-C. He, J. Org. Chem., 1990, 55, 2114. CrossRef
4. (a) S. Fukuzawa, M. Miura, and H. Matsuzawa, Tetrahedron Lett., 2001, 42, 4167; CrossRef (b) P. G. H. Uiterweerd, M. van der Sluis, B. Kaptein, B. de Lange, R. M. Kellogg, and Q. B. Broxtermanb, Tetrahedron: Asymmetry, 2003, 14, 3479. CrossRef
5. K.-Y. Ko, W. J. Frazee, and E. L. Eliel, Tetrahedron, 1984, 40, 1333. CrossRef
6. L. Chacón-García, S. Lagunas-Rivera, S. Pérez-Estrada, M. E. Vargas-Díaz, P. Joseph-Nathan, J. Tamariz, and L. Gerardo Zepeda, Tetrahedron Lett., 2004, 45, 2141. CrossRef
7. K.-Y. Ko and K.-I. Kim, Bull. Korean Chem. Soc., 1998, 19, 911.
8. (a) W. F. Bailey, D. P. Reed, D. R. Clark, and G. N. Kapur, Org. Lett., 2001, 3, 1865; CrossRef (b) K.-Y. Ko and J.-Y. Park, Bull. Korean Chem. Soc., 2002, 23, 665. CrossRef
9. K.-Y. Ko and J.-Y. Park, Tetrahedron Lett., 1997, 38, 407. CrossRef
10. (a) W. Oppolzer, Tetrahedron, 1987, 43, 1969; CrossRef (b) Z.-L, Wu, H.-L, Wu, P.-Y. Wu, and B.-J. Uang, Tetrahedron: Asymmetry, 2009, 20, 1556. CrossRef
11. K. H. Ahn, S. Lee, and A. Lim, J. Org. Chem., 1992, 57, 5065. CrossRef
12. The enantiomeric excess of (R)-6 was found to be ca. 85% by the proton NMR study of its 2-phenyl-1,3-dioxolanes doped with Eu(hfc)3. See: E. L. Eliel and K.-Y. Ko, Tetrahedron Lett., 1983, 24, 3547. CrossRef
13. J. A. Dale and H. S. Mosher, J. Org. Chem., 1970, 35, 4002. CrossRef
14. It is well known that in cyclohexanes, an equatorial proton appears at a slightly lower field than an axial proton due to small diamagnetic anisotropy of a C-C bond. See: ‘Stereochemistry of Organic Compounds: Principles and Applications’, D. Nasipuri, Wiley, 1991. The equatorial nature of 1 (R = Me) was determined by 1H NMR spectrum, where for the equatorial and axial 1, the proton between oxygen and nitrogen resonates at δ 5.34 and 6.70, respectively.
15. Crude alcohol obtained from LiAlH4 reduction was purified by recrystallization from EtOH, yielding adequate crystals for X-ray crystallography.
16. K.-Y. Ko and H. Yun, Acta Cryst., 2007, E63, o4712.
17. Further details of the crystal structure investigations may be obtained from the Cambridge Crystallographic Data Centre (CCDC, 12 Union Road, Cambridge CB2 1EZ (UK); tel.: (+44)1223-336-408, fax: (+44)1223-336-033, e-mail: deposit@ccdc.cam.ac.uk) on quoting the depository number CCDC 672055.
18. The steric repulsion between gem-dimethyl group and phenyl group as well as the anomeric effect of phenyl group may contribute to the formation of axial anomer. See: ‘Introduction to Stereochemistry & Conformational Analysis’, E. Juaristi, Wiley-Interscience, 1991.
19. J. Lacoste, C. Soucy, F. D. Rochon, and L. Breau, Tetrahedron Lett., 1998, 39, 9121. CrossRef
20. In Felkin-Anh model, antiperiplanar effect requires that best acceptor σ* orbital should be aligned parallel to π and π* orbitals of carbonyl. It is known that σ* C-O bond is a better acceptor than σ* C-N bond. See: I. V. Alabugin and T. A. Zeidan, J. Am. Chem. Soc., 2002, 124, 3175. CrossRef
21. As shown in Figure 1, the sulfonyl oxygen atoms are disposed into the direction of carbonyl group.
22. L. A. Paquette, ‘Encyclopedia of Reagents for Organic Synthesis’, Vol. 2, John Wiley & Sons, Chichester, 1995, pp. 1401-1407.