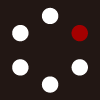
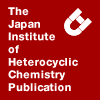
HETEROCYCLES
An International Journal for Reviews and Communications in Heterocyclic ChemistryWeb Edition ISSN: 1881-0942
Published online by The Japan Institute of Heterocyclic Chemistry
e-Journal
Full Text HTML
Received, 8th September, 2010, Accepted, 30th September, 2010, Published online, 1st October, 2010.
DOI: 10.3987/COM-10-12064
■ Novel Application of Electrooxidative Method for the Cyclization of N-Benzyl-2-(hydroxymethyl)- and N-Benzyl-2-(2-hydroxyethyl)piperidines
Mitsuhiro Okimoto,* Takashi Yoshida, Masayuki Hoshi, and Kousuke Ohashi
Department of Biotechnology and Environmental Chemistry, Kitami Institute of Technology, Koen-cho 165, Kitami, Hokkaido 090-8507, Japan
Abstract
Several novel 1,3-oxazinane and oxazolidine derivatives were obtained from the corresponding N-benzyl-2-(2-hydroxyethyl)- and N-benzyl-2-(hydroxymethyl)piperidines via electrochemical oxidation. The reactions were carried out in methanol under basic conditions. The yields of the cyclic products were significantly improved using catalytic amounts of iodide ions, which presumably act as effective electron carriers in the two-electron oxidation process.It is well known that electrooxidation provides a useful and an attractive methodology for the transformation of a variety of organic compounds into their oxidative forms.1 Such electrooxidation reactions are carried out under very mild reaction conditions without the use of hazardous oxidants. Although the oxidative strength of such reactions is relatively weaker than that of metal oxidants, the electrooxidative method often provides specific and/or unique reaction products that are difficult to obtain using typical chemical reagents.2 Previously we reported the electrooxidation of tetrahydroisoquinolyl alcohols A in the presence of KI in methanol under basic conditions (Scheme 1), in which, the hydoxy group attacked only the benzylic carbon to yield cyclic compound B, without any formation of cyclic compound C.3 Based upon the results, we reasoned that N-benzylpiperidines, which possess a hydroxy alkyl group (1), would undergo electrooxidative cyclization to afford the corresponding phenyloxazolidine (2, n = 1) or phenyl-1, 3-oxazinane (2, n = 2) derivatives (Scheme 2). In this study, we report the successful formation of cyclic compounds 2 (n = 1, 2) via electrochemical oxidation using 1 (n = 1, 2) as the starting substrates. Although there are some reports describing their syntheses and/or conformational analyses,4 the preparation of these compounds via electrooxidation has not been reported
to the best of our knowledge. Initially, the optimal reaction conditions for the electrooxidation were determined using N-benzyl-2-(2-hydroxyethyl)piperidine (1e) as the model substrate. The various combinations of the supporting electrolytes (mediators and bases), along with the corresponding yields of the resulting cyclized compounds 2e, are listed in Table 1. When NaOMe or KI was used alone, the yield of 2e was merely 35% (entry 1) and 40% (entry 2), respectively. Remarkably, the combination of KI (0.25 equivalents) and NaOMe (0.63 equivalents) increased the yield of 2e to 57% (entry 3) – furthermore, increasing the amount of NaOMe to 1.25 equivalents (entry 4) improved the yield to nearly 70%. The use of a strong base such as t-BuOK also resulted in a comparable yield of 68% (entry 9). In contrast, replacing NaOMe with a weaker base such as NaOH (entry 7) or NaOAc (entry 8) decreased the yields of 2e. Substitution of KI (entry 4) with KBr (entry 10) or KCl (entry 11) resulted in lower yields of 2e (37% and 31%, respectively). These results indicate that the yield of 2e is strongly affected by not only the catalytic amounts of KI, but by the nature of the base and the mediators. During the initial stages of the electrooxidation reaction, the yield of 2e was proportional to the amount of current passed – specifically, 34% after 3.0 Fmol-1, then 67% after 6.0 Fmol-1. However, as the reaction progressed, the efficiency of the reaction gradually decreased to 66% after 8.0 Fmol-1.
Accordingly, the consumption of 1e required significant amounts of electrical current. Subsequently, we applied our electrooxidation procedure using substituted N-benzyl-2-(2-hydroxyethyl)piperidines 1f-1h and N-benzyl-2-(hydroxymethyl)piperidines 1a-1d to afford 1,3-oxazinanes 2f-2h and oxazolidines 2a-2d, respectively (Table 2).5 During the reaction, the composition of the anolyte was monitored using GC analysis. Although the consumption of methanol derivatives 1a-1d were faster than that of ethanol derivatives 1e-1h, the yields of the corresponding products 2a-2d were lower than that of 2e-2h, which can be attributed to the difference in the structural strain between the five- and six-membered ring of the cyclized products. In both cases, substituent effects of the benzene ring, specifically in the para-position, were not observed, in terms of the yields of 2. For the low-yielding reactions, considerable amount of a tar-like material remained in the distillation flask and/or on the top of the column during chromatography purifications. Although the details of the reaction mechanism remain unclear, a proposed reaction pathway is illustrated in Scheme 3. At the beginning of the reaction, the iodide ion (I-) readily
undergoes a two-electron oxidation step on the anode to form an iodonium ion (I+), which would abstract one electron from the nitrogen atom of the substrate followed by deprotonation resulting in a stable benzylic radical 1e’’. Radical 1e’’ would immediately lose an electron to form cation 1e’’’, which would undergo an intramolecular nucleophilic attack by the oxygen atom of the hydroxy group to give the cyclic product. Accordingly, the presence of a strong base such as NaOMe would aid the deprotonation step during the course of the reaction. In a separate study, N-(2-phenyl)ethyl-2-(2-hydroxyethyl)piperidine was subjected to electrooxidation under similar conditions – unfortunately, the reaction was not specific and afforded multiple products, as shown by GC analysis. In this case, the substrate lacked a benzylic carbon that is directly adjacent to the nitrogen atom for the formation of a conjugate system. In conclusion, we have successfully carried out the electrochemical oxidations of N-benzyl-2- (2-hydroxyethyl)- and N-benzyl-2-(hydroxymethyl)piperidines. Although the yields were moderate, the reactions afforded novel products, and provided new insight into the peculiar electrooxidative behavior of N-benzyl-2-(hydroxyalkyl)piperidines. Moreover, the electrooxidation reactions could be carried out under very mild reaction conditions without the use of hazardous oxidants. Further investigations into the stereo-specificity of the reactions are currently underway in our laboratories.
References
1. R. F. Nelson, ‘Techniques of Electroorganic Synthesis’, ed. by N. L. Weinberg, Wiley, New York, 1974; S. Torii, ‘Electroorganic Synthesis’, Kodansha Ltd., Tokyo, 1985; M. Okimoto and Y. Takahashi, Curr. Org. Synth., 2004, 1, 233. CrossRef
2. M. Okimoto and T. Chiba, J. Org. Chem., 1988, 53, 218; CrossRef M. Okimoto, K. Numata, Y. Takahashi, M. Hoshi, K. Tomozawa, and T. Shigemoto, Synlett, 2005, 2507; CrossRef M. Okimoto, T. Yoshida, M. Hoshi, K. Hattori, M. Komata, K. Numata, and K. Tomozawa, Synlett, 2006, 1753; CrossRef S. Andreades and E. W. Zahnow, J. Am. Chem. Soc., 1969, 91, 4181; CrossRef S. Torii, T. Inokuchi, and N. Takahashi, J. Org. Chem., 1978, 43, 5020; CrossRef M. Okimoto, T. Yoshida, M. Hoshi, K. Hattori, M. Komata, K. Tomozawa, and T. Chiba, Heterocycles, 2008, 75, 35; CrossRef L. L. Miller and A. K. Hoffmann, J. Am. Chem. Soc., 1967, 89, 593; CrossRef D. K. Johnson and R. E. W. Jansson, J. Electrochem. Soc., 1981, 128, 1885. CrossRef
3. M. Okimoto, T. Yoshida, M. Hoshi, K. Hattori, M. Komata, K. Numata, and K. Tomozawa, Heterocycles, 2006, 68, 2563. CrossRef
4. B. Fernández, L. Carballeira, and M. A. Ríos, J. Mol. Struct., 1991, 53; CrossRef T. A. Crabb and R. F. Newton, Tetrahedron, 1968, 24, 4423; CrossRef T. Taguchi and S. Kasuga, Chem. Pharm. Bull., 1965, 13, 241; C. H. Tilford and M. G. Van Campen, Jr., J. Am. Chem. Soc., 1954, 76, 2431; CrossRef L. H. Goodson and H. Christopher, J. Am. Chem. Soc., 1950, 72, 358; CrossRef U. Azzena, L. Pilo, and E. Piras, Tetrahedron Lett., 2001, 42, 129; CrossRef U. Azzena, J. Chem, Soc., Perkin Trans. 1, 2002, 360; CrossRef A. G. Cook, C. A. Schering, P. A. Campbell, and S. S. Hayes, Tetrahedron Lett., 2005. 46. 5451. CrossRef
5. EXPELIMENTAL: N-Benzyl-2-(2-hydroxyethyl)- and N-benzyl-2-(hydroxymethyl)piperidines wereprepared by typical benzylation of 2-(hydroxymethyl)- or 2-(2-hydroxyethyl)piperidine, respectively, using benzyl chloride by refluxing in EtOH for 3h (83 - 93%). Preparative-scale electrooxidations were carried out in a tall 50 mL beaker equipped with a fine frit cup as the cathode compartment with a nickel coil cathode, along with a cylindrical platinum net anode (50 mesh). Typical procedures: a solution of N-benzyl-2-(2-hydroxyethyl)piperidine (8 mmol) in MeOH (40 mL) containing KI (2 mmol) and NaOMe (10 mmol) was electro-oxidized under a constant current (0.3 A) with magnetically stirring at ca. 5 °C. While monitoring the reaction using GC analysis, electric current was passed until almost all of the substrate was consumed. Upon passage of the electrolytic current (6.2 F mol-1), the reaction mixture was concentrated in vacuo (ca. 10 mL) at approximately 50 °C. The resulting residue was extracted using Et2O (3×40 mL), in the absence of water. The resulting cloudy ether solution was dried over sodium sulfate overnight, filtered, then concentrated in vacuo to afford the crude product. The cyclized products were purified by distillation under reduced pressure and/or by silica gel column chromatography using Et2O/n-hexane as the eluent. The boiling and/or melting points, IR, NMR, and MS data of the products are listed below.
2a bp 151-153 ˚C/15 mmHg. Silica gel TLC: Rf = 0.68 (Et2O : n-hexane = 1:1). IR (neat): 2937, 2871, 1456, 1377, 1099, 1078, 1024, 755, 699 cm-1. 1H NMR (CDCl3):δ= 1.18-1.14 (m, 1H), 1.44-1.66 (m, 3H), 1.72-1.86 (m, 2H), 1.98-2.06 (m, 1H), 2.44-2.54 (m, 1H), 2.64-2.74 (m, 1H), 3.60-3.74 (m, 1H), 4.04-4.16 (m, 1H), 4.59 (s, 1H), 7.18-7.38 (m, 3H, Arom), 7.38-7.48 (m, 2H, Arom). 13C NMR (CDCl3):δ= 23.52 (CH2), 24.75 (CH2), 26.59 (CH2), 47.14 (CH2), 62.77 (CH), 71.23 (CH2), 96.62 (CH), 128.00 (CH), 128.18 (CH), 128.84 (CH), 139.18 (C). MS m/z (%): 203(36) [M+], 202(73), 173(34), 172(60), 126(100) [M+-C6H5], 105(39), 98(24), 91(51), 77(29), 65(13). HRMS: m/z calcd. for C13H17NO: 203.1310; found: 203.1324 [M+].
2b mp 48-49 ˚C. Silica gel TLC: Rf = 0.67 (Et2O : n-hexane = 1:1). IR (KBr): 2944, 1326, 1196, 1149, 1124, 1090 (s), 1017, 935, 821, 804 cm-1. 1H NMR (CDCl3):δ = 1.18-1.42 (m, 2H), 1.45-1.46 (m, 1H), 1.57-1.65 (m, 1H), 1.78-1.86 (m, 2H), 1.96-2.06 (m, 1H), 2.34 (s, 3H, CH3), 2.43-2.52 (m, 1H), 2.66-2.73 (m, 1H), 3.62-6.68 (m, 1H), 4.01-4.07 (m, 1H), 4.56 (s, 1H), 7.15 (d, J = 8Hz, 2H, Arom), 7.34 (d, J = 8Hz, 2H, Arom). 13C NMR (CDCl3):δ= 21.26 (CH3), 23.56 (CH2), 24.78 (CH2), 26.99 (CH2), 41.18 (CH2), 62.79 (CH), 71.17 (CH2), 96.54 (CH), 127.94 (CH), 128.91 (CH), 136.23 (C), 138.58 (C). MS m/z (%): 217(49) [M+], 216(99), 187(34), 186(63), 127(26), 126(100) [M+-C6H4CH3], 119(34), 98(25), 91(37), 41(28). HRMS: m/z calcd. for C14H19NO: 217.1467; found: 217.1457 [M+].
2c mp 30-31 ˚C. Silica gel TLC: Rf = 0.54 (Et2O : n-hexane = 1:1). IR (KBr): 2943 (s), 1613, 1515, 1300, 1248 (s), 1169, 1092, 1026 (s), 825, 807 cm-1. 1H NMR (CDCl3):δ= 1.22-1.42 (m, 2H), 1.44-1.56 (m, 1H), 1.58-1.65 (m, 1H), 1.78-1.87 (m, 2H), 1.95-2.05 (m, 1H), 2.43-2.51 (m, 1H), 2.64-2.72 (m, 1H), 3.61-3.67 (m, 1H), 3.79 (s, 3H, CH3O), 4.00-4.06 (m, 1H), 4.55 (s, 1H), 6.88 (d, J = 8Hz, 2H, Arom), 7.38 (d, J = 8Hz, 2H, Arom). 13C NMR (CDCl3):δ= 23.57 (CH2), 24.80 (CH2), 27.03 (CH2), 47.17 (CH2), 55.23 (CH3O), 67.75 (CH), 71.08 (CH2), 96.34 (CH), 113.61 (CH), 129.25 (CH), 131.30 (C), 160.12 (C). MS m/z (%): 233(26) [M+], 232(100), 203(14), 202(32), 135(28), 126(56) [M+-C6H4OCH3], 121(15), 119(5), 96(5), 79(9), 41(7). HRMS: m/z calcd. for C14H19NO2: 233.1416; found : 233.1421 [M+].
2d mp 36-37 ˚C. Silica gel TLC: Rf = 0.66 (Et2O : n-hexane = 1:1). IR (KBr): 2939 (s), 2804, 1492, 1363, 1089 (s), 1023, 1015, 841, 823, 807 cm-1. 1H NMR (CDCl3):δ= 1.18-1.40 (m, 2H), 1.42-1.55 (m, 1H), 1.58-1.72 (m, 1H), 1.75-1.91 (m, 2H), 1.97-2.08 (m, 1H), 2.42-2.54 (m, 1H), 2.62-2.70 (m, 1H), 3.62-3.70 (m, 1H), 4.02-4.08 (m, 1H), 4.56 (s, 1H), 7.32 (d, J = 8Hz, 2H, Arom), 7.39 (d, J = 8Hz, 2H, Arom). 13C NMR (CDCl3):δ= 23.49 (CH2), 24.74( CH2), 26.96 (CH2), 47.09 (CH2), 62.74 (CH), 71.33 (CH2), 95.82 (CH), 128.43 (CH), 129.44 (CH), 134.59 (C), 137.87 (C). MS m/z (%): 239(20) [M+, 37C], 238(46), 237(56) [M+, 35C], 236(85), 208(43), 207(46), 206(61), 139(43), 127(43), 126(100) [M+-C6H4Cl], 41(38). HRMS: m/z calcd. for C13H16NOCl: 237.0920; found: 237.0952 [M+].
2e bp 113-115 ˚C/2 mmHg, mp 53-55 ˚C. Silica gel TLC: Rf = 0.53 (Et2O : n-hexane = 1:1). IR (KBr): 2934, 2844, 1453, 1369, 1130, 1118, 1083, 755, 670 cm-1. 1H NMR (CDCl3):δ= 1.22-1.34 (m, 1H), 1.38-1.52 (m, 4H), 1.58-1.76 (m, 3H), 1.82-1.96 (m, 1H), 2.20-2.30 (m, 1H), 2.34-2.44 (m, 1H), 3.60-3.74 (m, 1H), 4.06-4.16 (m, 1H), 4.34 (s, 1H), 7.22-7.38 (m, 3H, Arom), 7.39-7.52 (m, 2H, Arom). 13C NMR (CDCl3):δ= 24.10 (CH2), 25.53 (CH2), 32.42 (CH2), 32.74 (CH2), 49.03 (CH2), 61.41 (CH), 67.82 (CH2), 97.39 (CH), 127.88 (CH), 128.25 (CH), 128.52 (CH), 139.86 (C). MS m/z (%): 217(4) [M+], 173(83), 172(43), 140(42) [M+-C6H5], 105(15), 96(20), 92(28), 91(100), 82(18), 77(13), 65(20). HRMS: m/z calcd. for C14H19NO: 217.1467; found: 217.1446 [M+].
2f mp 66-67 ˚C. Silica gel TLC: Rf = 0.49 (Et2O : n-hexane = 1:1). IR (KBr): 2934 (s), 2922, 1368, 1255, 1118 (s), 1096, 1081, 1043, 817 cm-1. 1H NMR (CDCl3):δ= 1.21-1.34 (m, 1H), 1.35-1.54 (m, 4H), 1.56-1.75 (m, 3H), 1.82-1.95(m, 1H), 2.18-2.28 (m, 1H), 2.33 (s, 3H, CH3), 2.80-3.45 (m, 1H), 3.65-3.74 (m, 1H), 4.07-4.12 (m, 1H), 4.30 (s, 1H), 7.14 (d, J = 8Hz, 2H, Arom), 7.34 (d, J = 8Hz, 2H, Arom). 13C NMR (CDCl3):δ= 21.23 (CH3), 24.14 (CH2), 25.55 (CH2), 32.48 (CH2), 32.76 (CH2), 49.12 (CH2), 61.54 (CH), 67.83 (CH2), 97.33 (CH), 127.77 (CH), 128.96 (CH), 136.94 (C), 138.22 (C). MS m/z (%): 231(23) [M+], 230(32), 187(64), 140(79) [M+-C6H4CH3], 120(42), 112(35), 105(100), 91(31), 83(34), 55(35). HRMS: m/z calcd. for C15H21NO: 231.1623; found: 231.1657 [M+].
2g mp 88-89 ˚C. Silica gel TLC: Rf = 0.30 (Et2O : n-hexane = 1:1). IR (KBr): 2921 (s), 1615, 1517, 1245 (s), 1175, 1115, 1079 (s), 1030, 831, 821 cm-1. 1H NMR (CDCl3):δ= 1.18-1.33 (m, 1H), 1.37-1.52 (m, 4H), 1.58-1.76 (m, 4H), 1.80-1.95 (m, 1H), 2.17-2.27 (m, 1H), 2.37-2.44 (m, 1H), 3.65-3.74 (m, 1H), 3.78 (s, 3H, CH3O), 4.28 (s, 1H), 6.86 (d, J = 8Hz, 2H, Arom), 7.37 (d, J = 8Hz, 2H, Arom). 13C NMR (CDCl3):δ= 24.18 (CH2), 25.59 (CH2), 32.57 (CH2), 32.80 (CH2), 49.18 (CH2), 55.23 (CH3O), 61.58 (CH), 67.80 (CH2), 97.02 (CH), 113.63 (CH), 129.00 (CH), 132.25 (C), 159.74 (C). MS m/z (%): 247(2) [M+], 246(3), 203(18), 140(7) [M+-C6H4OCH3], 136(9), 135(6), 122(10), 121(100), 92(4), 77(5), 76(6). HRMS: m/z calcd. for C15H21NO2: 247.1572; found: 247.1580 [M+].
2h mp 70-71 ˚C. Silica gel TLC: Rf = 0.54(Et2O : n-hexane = 1:1). IR (KBr): 2924 (s), 2847, 2788, 1367, 1226, 1119, 1083 (s), 1043, 1011, 820 cm-1. 1H NMR (CDCl3):δ= 1.18-1.32 (m, 1H), 1.33-1.53 (m, 4H), 1.56-1.76 (m, 3H), 1.80-1.94 (m, 1H), 2.20-2.30 (m, 1H), 2.32-2.40 (m, 1H), 3.64-3.74 (m, 1H), 4.06-4.13 (m, 1H), 4.32 (s, 1H), 7.31 (d, J = 8Hz, 2H, Arom), 7.40 (d, J = 8Hz, 2H, Arom). 13C NMR (CDCl3):δ= 24.00(CH2), 25.49 (CH2), 32.23 (CH2), 32.69 (CH2), 48.89 (CH2), 61.26 (CH), 67.81 (CH2), 96.42 (CH), 128.45 (CH), 129.30 (CH), 134.17 (C), 138.49 (C). MS m/z (%): 253(7) [M+, 37C], 251(20) [M+, 35C], 250(25), 207(44), 141(42), 140(100) [M+-C6H4Cl], 127(36), 125(66), 112(38), 83(36), 55(37). HRMS: m/z calcd. for C14H18NOCl: 251.1077; found: 251.1102 [M+].