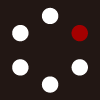
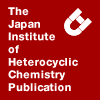
HETEROCYCLES
An International Journal for Reviews and Communications in Heterocyclic ChemistryWeb Edition ISSN: 1881-0942
Published online by The Japan Institute of Heterocyclic Chemistry
e-Journal
Full Text HTML
Received, 28th December, 2010, Accepted, 15th March, 2011, Published online, 16th March, 2011.
DOI: 10.3987/COM-10-12129
■ A Facile Synthesis of 2,5-Disubstituted Indole Derivatives as New Fluorescent Dyes
Hidetaka Hiyoshi, Jung-Hee Do, Xing Feng, Taisuke Matsumoto, and Takehiko Yamato*
Department of Applied Chemistry, Faculty of Science and Engineering, Saga University, Honjo-machi 1, Saga 840-8502, Japan
Abstract
A novel bis-arylated indole derivative, 2,5-bis(2-naphthyl)-1-methyl-1H-indole, was prepared and characterized. This compound shows the highly pure blue emissions both in solution and solid state which were ascribed to the expanded π-conjugation by introduction of two 2-naphthyl groups at the 2 and 5 positions on the indole ring. From X-ray crystallographic results this molecule exhibited the intermolecular CH–π interactions which consisted two dimensional (2D) herringbone structure.INTRODUCTION
Since Tang and Vanslyke investigated an electroluminescent device using organic materials as emitters,1 so many compounds have been researched for new electroluminescenct (EL) materials in last two decade. Among them, carbazole was one of the most attended materials which were depended on their p-doping nature. For example, carbazole derivatives widely used as several color emitters,2–4 hole-transporting materials5–7 and host materials for phosphorescence.8 The reasons why carbazole derivatives widely used in organic light-emitting diode’s (OLED’s) materials were mentioned as followings; i) Good electrochemical stability (reversible oxidation potentials were exhibited in the cyclic voltammetry measurements), ii) Good thermal stability and high glass transition temperature (Tg), iii) Easy to prepare variety of compounds (synthetic methods of carbazole based low molecular weight compounds and polymers have already established).
On the other hand, indole was well-known one of the most versatile heterocycles among biologically active natural products such as alkaloids, pharmaceuticals and marine products. Although indoles also had the p-doping nature, to the best of my knowledge, it had been very little studied until the present time.9–11 Thus there is substantial interest in investigating of the synthesis of substituted indoles and studies on the electronic absorption and fluorescence emission properties of these molecules. We wish to report here synthesis and optical and electrical properties of novel 2,5-bis-aryl-1-methyl-1H-indoles.
RESULTS AND DISCUSSION
When 5-bromo-1-methyl-1H-indolin-2-one 1 was reacted with phosphoric chloride in chlorobenzene, chlorinated product, N-methyl-5-bromo-2-chloroindole 2, was produced in 48% yield. The structure of 2 was elucidated based on the EI-MS, EA, 1H and 13C-NMR spectra and X-ray crystallographic analysis (Scheme 1 and Figure 1).
The EI-MS spectrum of 2 showed the molecular ion peaks 243, 245 and 247 which suggest to include with one chlorine atom and one bromine atom, respectively. The 1H-NMR spectrum in CDCl3 exhibited a doublet at δ 6.40 ppm (J = 0.6 Hz) for a H3 proton of indole ring and three signals for aromatic protons at δ 7.13 ppm (d, J = 8.6 Hz, H7), 7.29 ppm (dd, J = 1.8, 8.6 Hz, H6), and 7.64 ppm (dd, J = 0.6, 1.8 Hz, H4). Single colorless crystals of the 2 suitable for X-ray crystallography were obtained by recrystallization from methanol. The perspective ORTEP drawing of 2 is illustrated in Figure 1, with the atom numbering system.
Compound 2 crystallized in the monoclinic space group P21/a (no. 14) and has one independent molecule (Z = 4) in the equivalent position.
We had seen much attention to optical properties of 5-substituted indole derivatives, therefore, we have chosen 5-bromo-2-chloro-1-methyl-1H-indole 2 as a key intermediate for preparing the light emitting materials. To the best our knowledge, the arylation reaction of 2-chloro-1-methyl-1H-indoles via the Suzuki reaction was only reported using microwave irradiation by Gong and He (Scheme 2).12
Thus, we have attempted to prepare 2,5-bis-aryl-1-methyl-1H-indole derivatives starting from 5-bromo- 2-chloro-1-methyl-1H-indole 2. In fact, arylation of 2 with phenylboronic acid under the normal Suzuki- Miyaura cross-coupling reaction gave 2,5-diphenyl-1-methyl-1H-indole 4a in 58% yield. The results using several arylboronic acids to establish the limitation of the present coupling reaction were shown in Scheme 3.
Similarly, when 2-naphthylboronic acid was reacted with 2, the desired 2,5-disubstituted product, 2,5- bis(2-naphthyl)-1-methyl-1H-indole 4b was produced in 23% yield. On the other hand, when 1-naphthyl- boronic acid was used under the same reaction conditions above, the desired 2,5-disubstituted product 4c was not obtained. Only 5-mono-substituted product, 2-chloro-5-(1-naphthyl)-1-methyl-1H-indole 3c was produced in 75% yield. From this result, it is supposed to be the steric hindrance 1-naphthylboronic and the reactivity of 2-chloro moiety of 5-bromo-2-chloro-1-methyl-1H-indole 2 might suppress the second Suzuki coupling reaction.
Single-crystal X-ray diffraction structures of 2-chloro-5-(1-naphthyl)-1-methyl-1H-indole (3c) and 2,5-bis(2-naphthyl)-1-methyl-1H-indole (4b) recrystallized from hexane are illustrated in Figures 2 and 3, with the atom numbering system. Compounds 3c and 4b both crystallized in the monoclinic space group P21/n (no. 14). Compound 3c has one independent molecule (Z = 2) in the equivalent position. Interestingly, the dihedral angle between indole π-face and naphthalene π-face in 3c was 60.0°. In addition, optimized structure of 3c was calculated using Gaussian 03, B3LYP/6-31G*. The dihedral angle between indole π-face and naphthalene π-face was also calculated to 58.1° which was relatively close to result of the X-ray crystallographic analysis (Figure 2). These findings indicate the restricted expanded π-conjugation between indole ring and naphthyl moiety due to avoid the steric hidrance diaryl linkages. On the other hand, in the case of 4b, the dihedral angle between indole π-face and 2-position of naphthalene π-face was 32.4°.
The electrochemical properties of 4b were investigated by the cyclic voltammetry measurements in CH2Cl2 in the presence of Bu4NPF6 as supporting electrolyte using a glassy-carbon working electrode and Ag/AgCl couple in electrolyte solution as the reference at room temperature. The cyclic voltammogram curve of 4b exhibited a well-defined redox wave. Oxidation potential (E0) associated with generation of the cation radical were evaluated at 0.76 eV. In comparison with that of triphenyl amine which was usually used for OLEDs, the oxidation potential (E0) of 4b was lower than that of triphenylamine one (E0= 0.92 eV). It was supposed that this indole had high donor ability and attracted as key material to design and prepare of the indole-based functional materials.
The optical properties were investigated about 2,5-bis(2-naphthyl)-1-methyl-1H-indole 4b which was only emitted among the present indole derivatives. The absorption and fluorescence spectra of 4b were shown in Figures 4 and 5. The absorption spectrum of 4b exhibited two bands around 267 nm and 306 nm which were assigned to the π → π* transitions (Figure 4). The fluorescence spectrum of 4b was observed in CH2Cl2 in 1 × 10-6 M and solid state. The fluorescence peak in dichloromethane solution appeared mainly around 418 nm with 460 nm as a shoulder peak, and large Storks shift (102 nm) was presented. The relative quantum yield was determined to be 0.44 using quinine sulfate as the reference. The fluorescence spectrum in solid state was also appeared around 406 nm with a shoulder peak (420 nm). The much broader fluorescence spectrum and large Storks shift were characteristic of intermolecular excimer emissions.13,14
Since fluorescence spectra both in dichloromethane solution and solid state of 4b suggested the excimer emissions, we have examined solid state structure. Fortunately, as mentioned previously, we have succeeded single crystal measurement of 4b. As mentioned previously in Figure 1, 5-bromo-2-chloro- 1-methyl-1H-indole 2 which was starting material of 4b was shown intermolecular π-π interaction (π-π stacking) at the central indole ring. This π-π stacking was consisted one dimensional (1D) columnar structure along with a-axis.
On the other hand, Figure 6 showed molecular packing of 4b. Each molecule did not have intermolecular π-π stacking between central indole ring and/or substituted naphthalene ring. Instead of π-π stacking, at the naphthalene rings which were substituted to 2 and 5 positions of central indole ring, intermolecular two dimensional (2D) CH–π interactions which were consisted herringbone structure15–17 were presented. It was supposed that the expanded π-conjugation by introduction of two 2-naphthyl groups at the 2 and 5 positions on the indole ring enhanced the highly pure blue emissions of 4b both in the solution and solid state.
CONCLUSION
We have developed a facile synthesis of novel 2,5-bis-arylated indoles, 2,5-diphenyl-1-methyl-1H-indole 4a and 2,5-bis(2-naphthyl)-1-methyl-1H-indole 4b. Thus, the coupling reaction of 5-bromo-2-chloro- 1-methyl-1H-indole 2 with phenyl- or 2-naphthylboronic acid afforded the desired double coupling products, 2,5-bisaryl-1-methyl-1H-indoles 4 in good yields. Interestingly, the bis-arylated compound, 2,5-bis(2-naphthyl)-1-methyl-1H-indole 4b, was shown the highly pure blue emissions both in the solution and solid state. The highly pure blue emission was ascribed to the expanded π-conjugation by introduction of two 2-naphthyl groups at the 2 and 5 positions on the indole ring. Interestingly, the intermolecular C-H–π interaction of naphthalene ring linked to 2 and 5 positions of central indole ring exhibited in the solid state. 2,5-Bis(2-naphthyl)-1-methyl-1H-indole 4b exhibited reversible oxidation potentials (E0) around 0.76 V which was lower than triphenylamine (E0= 0.92 V) which was usually used for OLEDs. Thus, 2,5-bis(2-naphthyl)-1-methyl-1H-indole 4b is promising candidates for an OLED emitting or hole-transporting materials.
EXPERIMENTAL
All melting points are uncorrected. The 1H-NMR spectra were determined in CDCl3 using a VARIAN Mercury 300 spectrometer. Residual solvent protons were used as the internal standard and the chemical shifts (δ) are given relative to tetramethylsilane (TMS). The coupling constants (J) are reported in hertz (Hz). The elemental analyses were performed at the Elemental Analytical Center, Kyushu University. The electron impact mass spectrometry (EI-MS) spectra were recorded by a JEOL JMS-70 mass spectrometer at 70 eV using a direct inlet system. The fast atom bombardment mass spectrometry (FAB-MS) spectra were recorded by a JEOL JMS-70 mass spectrometer with m-nitrobenzyl alcohol (NBA) as the matrix. The UV-vis spectra were measured by a JASCO V-570 spectrophotometer in a 1.0 cm wide quartz cell (0.01 mM). The fluorescence spectra were measured by a HITACHI F-4500 fluorescence spectrophotometer. The cyclic voltammetry measurements were performed by a BAS model 600 electrochemical analyzer in deaerated dichloromethane solution (0.5 mM) containing tetrabutyl ammonium hexafluoro-phosphate (0.1 M) as the supporting electrolyte at 298 K (100 mV s-1). The glassy carbon working electrode was polished with BAS polishing alumina suspension and rinsed with acetone before use. The counter electrode was a platinum wire. The measured potentials were recorded with respect to an Ag/AgCl (saturated KCl) reference electrode. The analytical TLC was carried out on silica gel coated glass-plate (Merck 60 F254). Column chromatography was carried out on silica gel (KANTO 60 N). The reagents were purchased from commercial suppliers and used without further purification.
Materials: Preparation of 5-bromo-1-methyl-1H-indolin-2-one 1 was previously described.18,19
Preparation of 5-bromo-2-chloro-1-methyl-1H-indole 2. A mixture of 5-bromo-1-methyl-1H- indolin-2-one (1) (2.26 g, 10 mmol) and chlorobenzene (100 mL) was added phosphoric chloride (3.0 g) under a nitrogen atmosphere. The resulting mixture was heated at 100 °C for 4 h. The reaction mixture was cooled to room temperature and evaporated in vacuo. The residue was separated by silica gel column chromatography (KANTO 60 N) eluting with AcOEt/hexane (1:1) to give 5-bromo-2-chloro-1-methyl- 1H-indole (2) (1.07 g, 48%) as a white solid. Recrystallization from hexane gave 2 as colorless prisms; mp 71–72 °C; ν max (KBr)/cm-1: 1669 (C=O); δH (CDCl3) 3.71 (3H, s, Me), 6.40 (1H, d, J = 0.6 Hz, ArH), 7.13 (1H, d, J = 8.6 Hz, ArH), 7.29 (1H, dd, J = 1.8. 8.6 Hz, ArH), 7.64 (1H, dd, J = 0.6, 1.8 Hz, ArH); δC (CDCl3) 30.2, 99.4, 110.9, 113.7, 122.5, 124.7, 127.5, 128.7, 135.0; m/z 243, 245, 247 (M+). Anal. Calcd for C9H7BrClN (244.52): C, 44.21; H, 2.89; N, 5.73. Found: C, 44.40; H, 2.83; N, 5.74%.
Preparation of 2,5-diphenyl-1-methyl-1H-indole 4a. To a mixture of 2 (240 mg, 0.98 mmol), tetrakis(triphenylphosphine)palladium(0) (5 mol%) in toluene (80 mL), and saturated aqueous NaHCO3 (40 mL) was added phenylboronic acid (720 mg, 5.90 mmol) in EtOH (5 mL) under a nitrogen atmosphere. The resulting mixture was heated at refluxing temperature for 4 h. The reaction mixture was poured into water and extracted with AcOEt (100 mL). The organic layer was washed with brine (50 mL), dried over anhydrous MgSO4, and evaporated in vacuo to dryness. The residue was separated by silica gel column chromatography (KANTO 60N) eluting with CHCl3 to give 2,5-diphenyl-1-methyl-1H-indole 4a as a white solid (160 mg, 58%). Recrystallization from hexane gave 4a as colorless prisms; mp 193–194 °C (dec.); 1H-NMR: δ (ppm, CDCl3) 3.79 (3H, s, Me), 6.62 (1H, broad s, ArH), 7.32 (1H, t, J = 9.3Hz, ArH), 7.38–7.56 (9H, m, ArH), 7.68 (2H, d, J = 7.2 Hz, ArH), 7.86 (1H, d, J = 1.5 Hz, ArH); MS (EI, m/z) 283 (M+). Anal. Calcd for C21H17N (283.38): C, 89.01; H, 6.05; N, 4.94. Found: C, 89.08; H, 6.00; N, 5.00%.
Preparation of 2,5-bis(2-naphthyl)-1-methyl-1H-indole 4b. According to a method similar to the preparation of 4b was obtained in 23% yield from 2 as a white solid. Recrystallization from hexane gave 4b as colorless prisms; mp 201–203 °C; 1H-NMR: δ (ppm, CDCl3) 3.87 (3H, s, Me), 6.75 (1H, s, ArH), 8.1–7.2 (17H, m, ArH); MS (EI, m/z) 383 (M+). Anal. Calcd for C29H21N (383.5): C, 90.83; H, 5.52; N, 3.65. Found: C, 90.71; H, 5.57; N, 3.62%.
Preparation of 2-chloro-5-(1-naphthyl)-1-methyl-1H-indole 3c. According to a method similar to the preparation of 3c was obtained in 75% yield from 2 as a white solid. Recrystallization from hexane gave 2 as colorless prisms; mp 133–135 °C; 1H-NMR: δ (ppm, CDCl3) 3.83 (3H, s, Me), 6.51 (1H, s, ArH), 7.2–7.6 (7H, m, ArH), 7.8–8.0 (3H, m, ArH); MS (EI, m/z) 291 and 293 (M+). Anal. Calcd for C19H14ClN (291.78): C, 78.21; H, 4.84; N, 4.8. Found: C, 78.19; H, 4.86; N, 4.78%.
Crystallographic data for 2, 3c, and 4b.
Crystal data for 2・H2O: C9H9NBrClO, M = 262.53, colorless needle, monoclinic, space group P21/a (no. 14), a = 4.598(2) Å, b = 32.818(15) Å, c = 6.347(3) Å, β = 99.2288(16)°, V = 945.4(7) Å3, T = 123 K, Z = 4, Dc = 1.844 g cm-1, F(000) = 520, μ = 45.985 cm-1, crystal dimensions 0.15 × 0.08 × 0.06 mm3, 8965 reflections measured on a Rigaku Saturn CCD diffractometer, of which were independent, data corrected for absorption on the basis of symmetry equivalent and repeated data (min and max transmission factors: 0.646 0.759) and Lp effects, Rint = 0.042, structure solved by direct methods (Sir2002), F2 refinement, R1 = 0.0781 for 8965 data with F2> 2σ(F2), wR2= 0.2007, S = 1.236 for all data, 2140 parameters.
Crystal data for 3c: C19H14ClN, M = 291.78, colorless prism, monoclinic, space group P21/n (no. 14), a = 13.951(8) Å, b = 7.007(5) Å, c = 15.714(8) Å, β = 108.45(5)°, V = 1457(1) Å3, T = 123 K, Z = 4, Dc = 1.330 g cm-1, F(000) = 608, μ = 2.54 cm-1, crystal dimensions 0.30 × 0.20 × 0.15 mm3, 3311 reflections measured on a Rigaku RAXIS RAPID diffractometer, of which were independent, data corrected for absorption on the basis of symmetry equivalent and repeated data (min and max transmission factors: 0.220 0.963) and Lp effects, Rint = 0.000, structure solved by direct methods (Sir97), F2 refinement, R1 = 0.0715 for 3311 data with F2> 2σ(F2), wR2 = 0.1916, S = 1.040 for all data, 3311 parameters.
Crystal data for 4b: C29H21N, M = 383.49, colorless prism, monoclinic, space group P21 (no. 4), a = 7.277(3) Å, b = 7.214(4) Å, c = 18.891(9) Å, β = 94.04(2)°, V = 989.3(8) Å3, T = 123K, Z = 2, Dc = 1.287 g cm-1, F(000) = 404, μ = 0.74 cm-1, crystal dimensions 0.40 × 0.30 × 0.20 mm3, 2419 reflections measured on a Rigaku RAXIS RAPID diffractometer, of which were independent, data corrected for absorption on the basis of symmetry equivalent and repeated data (min and max transmission factors: 0.689 0.985) and Lp effects, Rint = 0.000, structure solved by direct methods (Sir97), F2 refinement, R1 = 0.0546 for 3311 data with F2> 2σ(F2), wR2 = 0.1488, S = 1.031 for all data, 2419 parameters.
Crystallographic data (excluding structure factors) for the structures in this paper have been deposited with the Cambridge Crystallographic Data Centre as supplementary publication numbers CCDC 778128 for 2, CCDC 778129 for 3c, and CCDC 778130 for 4b, respectively. Copies of the data can be obtained, free of charge, on application to CCDC, 12 Union Road, Cambridge CB2 1EZ, UK [fax: 144-1223-336033 or e-mail: deposit@ccdc.cam.ac.uk].
References
1. C. W. Tang and S. A. Vanslyke, Appl. Phys. Lett., 1987, 51, 913. CrossRef
2. K. D’Almeida, J. C. Bernede, S. Marsillac, A. Godoy, and F. R. Diaz, Synthetic Metals, 2001, 122, 127. CrossRef
3. Y. Liu, M. Nishiura, Y. Wang, and Z. Hou, J. Am. Chem. Soc., 2006, 128, 5592. CrossRef
4. R. M. Adhikari, R. Mondal, B. K. Shah, and D. C. Neckers, J. Org. Chem., 2007, 72, 4727. CrossRef
5. Q. Zhang, J. Chen, Y. Cheng, L. Wang, D. Ma, X. Jing, and F. Wang, J. Mater. Chem., 2004, 14, 895. CrossRef
6. S. Lengvinaite, J. V. Grazulevicius, V. Jankauskas, and S. Grigalevicius, Synthetic Metals, 2007, 157, 529. CrossRef
7. J. H. Kim, D. Y. Yoon, J. W. Kim, and J.-J. Kim, Synthetic Metals, 2007, 157, 743. CrossRef
8. A. V. Dijken, J. J. A. M. Bastiaansen, N. M. M. Kiggen, B. M. W. Langeveld, C. Rothe, A. Monkman, I. Bach, P. Stossel, and K. Brunner, J. Am. Chem. Soc., 2004, 126, 7718. CrossRef
9. L. Tung-shen, US patent publication, 6713193.
10. L. Tung-shen, US patent publication, 6815093.
11. T. Tanaka, T. Fukuda, and A. Suzuki, Jpn. Kokai Tokkyo, Koho (1999) JP 11260555A 19990924.
12. Y. Gong and W. He, Org. Lett., 2002, 4, 3803. CrossRef
13. A. S. Jenekhe and L. X. Chen, J. Phys. Chem. B, 2000, 104, 6332. CrossRef
14. C. Huang, C.-G. Zhen, S. P. Su, K. P. Loh, and Z.-K. Chen, Org. Lett., 2005, 7, 391. CrossRef
15. M. D. Curtis, J. Cao, and J. W. Kamp, J. Am. Chem. Soc., 2004, 126, 4318. CrossRef
16. M. M. Payne, S. R. Parkin, J. E. Anthony, C. C. Kuo, and T. N. Jackson, J. Am. Chem. Soc., 2005, 127, 4986. CrossRef
17. H. Ebata, T. Izawa, E. Miyazaki, K. Takimiya, M. Ikeda, H. Kuwabata, and T. Yui, J. Am. Chem. Soc., 2007, 129, 15732. CrossRef
18. H. Hiyoshi, T. Sonoda, and S. Mataka, Heterocycles, 2006, 68, 763. CrossRef
19. H. Hiyoshi, H. Kumagai, H. Ooi, T. Sonoda, and S. Mataka, Heterocycles, 2007, 72, 231. CrossRef