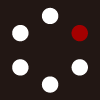
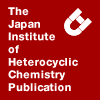
HETEROCYCLES
An International Journal for Reviews and Communications in Heterocyclic ChemistryWeb Edition ISSN: 1881-0942
Published online by The Japan Institute of Heterocyclic Chemistry
e-Journal
Full Text HTML
Received, 5th January, 2011, Accepted, 21st February, 2011, Published online, 22nd February, 2011.
DOI: 10.3987/COM-11-12135
■ Synthesis and Emission Properties of 1,6-Methano[10]annulene-3,4-dicarboximides
Shigeyasu Kuroda,* Naoko Tamura, Ryuta Miyatake, Naoko Matsumoto, Yoshikazu Horino, Daisuke Miyawaki, and Mitsunori Oda*
Department of Environmental Applied Chemistry, Faculty of Engineering, Toyama University, Gofuku 3190, Toyama 930-8555, Japan
Abstract
A mixture of 1,6-methano[10]annulene-3,4-dicarboximide (1e) and 1,6-methano[10]annulene-3,4-dicarboxylic anhydride (3) was obtained through a two-step sequence involving cyanation and subsequent hydrolysis from ethyl 4-bromo-1,6-methano[10]annulene-3-carboxylate (5). Alkylations of 1e provided 1f and 1g, and the nucleophilic substitution of p-fluoronitrobenzene with 1e yielded 1i. Copper-catalyzed arylations of 1e with arylhalides gave 1a and 1h. Reactions of 3 with anilines afforded 1a and 1h. Emission properties of the imides obtained are also reported.INTRODUCTION
Spectroscopic properties and photochemistry of 2,3-naphtalimides have long received much attention, especially their luminescent behavior,1 photocycloadditions2 and photoreductions.3 Quite recently Heagy
et al. reported that dual fluorescent 2,3-naphtalimides could be used in ratiometric DNA detection and white organic light-emitting devices.4 During the course of our continuing research on 1,6- methano[10]annulenes,5 we have been interested in physical and chemical properties of 1,6-methano[10]annulene-3,4-dicarboximides 1, which are structurally related to 2,3-naphtalimides. Recently Zuo et al. reported synthesis of 1a–d from diethyl 1,6-methano[10]annulene-3,4-dicarboxylate (2) as shown in Scheme 1.6 Their papers prompt us to report our independent synthetic efforts for the imides7 and also emission properties thereof.8
RESULTS AND DISCUSSION
Our synthesis started with ethyl 4-bromo-1,6-methano[10]annulene-3-carboxylate (5), which was prepared in three steps from 1,3,5-cycloheptatriene-1,6-dicarbaldehyde (4)9 by the Neidlein’s method.10 Cyanation of 5 with CuCN in DMF11 gave 6 in 91% yield. Hydrolysis of 6 with NaOH in refluxing dioxane and subsequent acidification provided a mixture of 3 and 1e in 51 and 29% yields, respectively (Scheme 2).
Reactions of 3 with aniline and p-anisidine in refluxing xylene afforded 1a and 1h in 32 and 49% yields, respectively. These results confirm the data reported by Zuo et al.6 On the other hand, alkylations of 1e with methyl- and ethyliodides under basic conditions gave 1f and 1g in 90 and 88% yields, respectively, and the nucleophilic substitution of p-fluoronitrobenzene with 1e in DMF provided 1i in 94% yield. Copper-catalyzed couplings12 of 1e with arylhalides were also examined. Iodobenzene and p-bromoanisole react with 1e in the presence of copper(I) oxide in refluxing quinoline to give 1a and 1h in 63 and 91% yields, respectively, which are better than those from 3.
Structures of all new compounds were characterized by spectroscopic and elemental or high-resolution mass spectrometric analyses. Hydrogens at the methylene bridge of the carboximides in their 1H NMR spectra are deshielded by the [10]annulene ring to appear at a range between δ 0.2 and –0.2. Their IR spectra show typical carbonyl stretching bands of a carboximide group at 1750–1760 and 1680–1690 cm–1. The carboximides exhibited four absorption bands in UV-Vis spectra (Figure 1). Among them, the bands around 290 nm are very strong. All carboximides except 1h exhibited emission upon excitation at the longest-wavelength absorptions. Figure 2 shows emission spectra of 1e in methanol for example. Their photophysical data are shown in Table 1. Kossanyi et al. reported that the quantum yield of N-phenyl-2,3-
naphtalimide (2 x 10–2%) was far smaller than those of the corresponding N-methyl derivative (24%) and the unsubstituted compound (26%).1 On the other hand, the quantum yield of 1a (8.5%) is comparable to those of 1f (9.7%) and 1g (9.6%) as shown in Table 1. Besides, it should be noted that the emission spectra of the carboximides 1 do not show dual emission as seen in 2,3-naphtalimides. These observations clearly reveal different emission properties of 1,6-methano[10]annulene-3,4-dicarboximides from those of 2,3-naphtalimides. Further studies on photochemical reactivity of the carboximides are now in progress.
CONCLUSION
The title 1,6-methano[10]annulene-3,4-dicarboximides 1 were synthesized by the independent synthetic method involving cyanation and subsequent functional transformations starting from ethyl 4-bromo-1,6-methano[10]annulene-3-carboxylate (5). Their emission spectra showed different properties from those of structurally related 2,3-naphtalimides.
EXPERIMENTAL
Melting points were measured on a Yanaco MP-3. IR spectra were recorded on a Perkin-Elmer Spectrum RX I spectrometer. UV-Vis spectra were measured on a Shimadzu UV-1600 spectrometer. Emission spectra were recorded in a degassed solution at rt on a Shimadzu RF-5300PC spectrometer. 1H and 13C-NMR were recorded with tetramethylsilane as internal standard on a JEOL α400 NMR instrument. Mass spectra were measured on a JMS-700 mass spectrometer. Column chromatography was done with Kieselgel 60. DMF and quinoline were purified by distillation from CaH2. Ethyl 4-bromo-1,6-methano[10]annulene-3-carboxylate was prepared from 1,3,5-cycloheptatriene-1,6- dicarbaldehyde9 according to a literature procedure.10 Emission quantum yields were determined by comparison of a total emission area with that of anthracene (Φ = 0.27, upon excitation at 356 nm in ethanol). The refractive indices, n = 1.33 (methanol), n = 1.37 (ethanol), and n = 1.35 (acetonitrile), were used in correction.
Synthesis of ethyl 4-cyano-1,6-methano[10]annulene-3-carboxylate (6)
A suspension of 4.15 g (14.2 mM) of 5 and 5.08 g (56.7 mM) of cuprous cyanide in 40 mL of DMF was refluxed on an oil bath for 3 h under nitrogen atmosphere. After the reaction mixture was cooled to room temperature, solids formed were removed by filtration and the filtrate was evacuated under reduced pressure. The residue was purified by column chromatography. Elution with 20%AcOEt-hexane gave 3.15 g of 6 as a yellow oil (93% yield). 1H NMR (CDCl3) δ = 8.33 (s, 1H), 7.90 (s, 1H), 7.40–7.65 (m, 4H), 4.49 (q, J = 7.2 Hz, 2H), 1.47 (t, J = 7.2 Hz, 3H), 0.04 (dt, J = 9.4, 0.1, 1H), –0.18 (dt, J = 9.4, 0.1 Hz, 1H) ppm; 13C NMR (CDCl3) δ = 166.9, 137.2, 134.2, 129.8, 129.3, 129.2, 128.5, 128.5, 120.1, 115.1, 114.2, 107.8, 62.3, 34.3, 14.1 ppm; IR (KBr) νmax = 3035w, 2983w, 2217m, 1719s, 1100s, 1050s, 870w, 830s, 773s cm-1; MS m/z (rel int) 239 (M+, 67), 211 (10), 194 (78), 167 (49), 166 (100), 140 (48), 139 (34); UV-Vis (CH3OH) λmax = 271 (logε = 4.88), 403 (3.04) nm. HRMS m/z Calcd for C15H13NO2 (M+) 239.0946, found 239.0924.
Hydrolysis of 6; Synthesis of 1,6-methano[10]annulene-3,4-dicarboximide (1e) and 1,6-methano[10]annulene-3,4-dicarboxylic anhydride (3)
A 15% NaOH aqueous solution (30 mL) was added nto a solution of 3.15 g (13.2 mM) of 6 in 25 mL of dioxane. This mixture was refluxed on an oil bath for 2 h. The resulted reaction mixture was poured in 100 mL of a saturated NaCl solution and was extracted with AcOEt (50 mL x 3). The combined organic layer was dried over MgSO4. The solvent was evaporated to give yellow solids, which were collected and washed with ether to give 0.81 g (29% yield) of 1e. The water layer was acidified with 3M HCl and the crystals formed were collected by filtration to give 1.41 g (51% yield) of 3.
1e: Light yellow microcrystals, mp 248–250 ºC. 1H NMR (CDCl3) δ = 8.27 (s, 2H), 7.74 (brt, 1H), 7.62 (m, 2H), 7.35 (m, 2H), 0.10 (d, J = 10.0 Hz, 1H), –0.20 (d, J = 10.0 Hz, 1H) ppm; 13C NMR (CDCl3) δ = 169.7, 130.7, 130.2, 129.3, 128.9, 119.4, 35.3 ppm; IR (KBr) νmax = 3528m, 3047m, 2955m, 1757vs, 1690vs, 1521s, 1331s, 1165s, 781s, 745s, 634m, 610w, 585m cm–1; UV-Vis (CH3OH) λmax = 242 (logε = 4.39), 285 (4.77), 324 (3.93), 419 (3.23) nm; MS m/z (rel int) 212 (M++1, 10), 211 (M+, 67), 210 (10), 168 (28), 167 (17), 165 (12), 141 (12), 140 (100), 139 (70). Anal. Calcd for C13H9NO2: C, 73.92; H, 4.29; N, 6.56%. Found C, 73.58; H, 4.44; N, 6.56%.
3: Creamy white prisms, mp 166–168 ˚C (lit.,6 165–166 ˚C). 1H-NMR (CDCl3) δ = 8.41 (s, 2H), 7.73 (m, 2H), 7.42 (m, 2H), –0.08 (d, J = 10.0 Hz, 1H), –0.14 (d, J = 10.0 Hz, 1H) ppm; 13C-NMR(CDCl3) δ = 165.5, 132.4, 131.1, 130.0, 126.5, 120.9, 34.5 ppm; MS m/z (rel int) 212 (M+, 35), 168 (32), 140 (100), 113 (12), 89 (15), 70 (19), 63 (23).
Synthesis of N-methyl-, N-ethyl-, and N-p-nitrophenyl-1,6-methano[10]annulene-3,4-dicarboximides (1f, 1g, and 1i)
To a solution of 70.1 mg (0.332 mM) of 1e in DMF was added 15.9 mg (0.390 mM) of 60% NaH in mineral oil. This mixture was stirred at rt under nitrogen atmosphere for 1h. Then, to this solution was added 31.0 µL (0.498 mM) of iodomethane. The resulted mixture was stirred at rt for further 2h, and then was poured into water. The solids formed were collected by filtration, washed with aqueous ethanol, and dried to give 67.0 mg (90% yield) of 1f. An analytical sample was obtained by recrystallization from hexane-dichloromehane. By the similar method with iodoethane (rt, 3 h) and p-fluoronitrobenzene (120 ˚C, 2 h), 1g and 1i were obtained in 88 and 94% yields, respectively.
1f: Yellow microcrystals, mp 168–170 ºC. 1H NMR (CDCl3) δ = 8.25 (s, 2H), 7.57 (m, 2H), 7.33 (m, 2H), 3.20 (s, 3H), 0.18 (d, J = 9.6 Hz, 1H), –0.22 (d, J = 9.6 Hz, 1H) ppm; 13C NMR (CDCl3) δ = 170.2, 130.3, 129.8, 129.1, 128.5, 119.3, 35.6, 24.3 ppm. IR (KBr) νmax = 3037w, 2956w, 2927w, 1750s, 1687vs, 1436s, 1384m, 1256w, 757s, 745s, 648m, 610w, 582m cm–1; UV-Vis (CH3OH) λmax = 246 (logε = 4.35), 286 (4.69), 325 (3.81), 420 (3.11) nm; MS m/z (rel int) 225 (M+, 100), 224 (50), 197 (25), 181 (69), 168 (100), 166 (30), 141 (72). HRMS m/z Calcd for C14H11NO2 (M+) 225.0790, found 225.0770.
1g: Yellow microcrystals, mp 163–165 ºC. 1H NMR (CDCl3) δ = 8.25 (s, 2H), 7.58 (m, 2H), 7.32 (m, 2H), 3.77 (q, J = 7.2 Hz, 2H), 1.28 (t, J = 7.2 Hz, 3H), 0.19 (d, J = 10.0 Hz, 1H), –0.22 (d, J = 10.0 Hz, 1H) ppm; 13C NMR (CDCl3) δ = 170.0, 130.2, 129.8, 129.0, 128.6, 119.3, 35.5, 33.2, 13.8 ppm; IR (KBr) νmax = 3036w, 2981w, 2947w, 1752s, 1689vs, 1402s, 1380m, 761m cm–1; UV-Vis (CH3OH) λmax = 247 (logε = 4.42), 286 (4.77), 326 (3.87), 420 (3.14) nm; MS m/z (rel int) 239 (M+, 36), 224 (82), 168 (40), 167 (9), 140 (100), 139 (65), 115 (13). Anal. Calcd for C15H13NO2: C, 75.30; H, 5.48; N, 5.85%, found C, 75.43; H, 5.45; N, 5.87%.
1i: Yellow solids, mp 268–270 ºC. 1H NMR (CDCl3) δ = 8.41 (s, 2H), 8.37 (d, J = 8.6 Hz, 2H), 7.82 (d, J = 8.6 Hz, 2H), 7.67 (m, 2H), 7.39 (m, 2H), 0.19 (d, J = 9.8 Hz, 1H), –0.10 (d, J = 9.8 Hz, 1H) ppm; 13C NMR (CDCl3) δ = 168.3, 146.3, 137.9, 131.4, 130.3, 129.6, 127.5, 126.3, 124.3, 120.0, 35.4 ppm; IR (KBr) νmax = 3079w, 1759s, 1703vs, 1518m 1493m, 1371s, 1337s, 847m, 756m, 745m cm–1; UV-Vis (CH3CN) λmax = 251 (logε = 4.27), 295 (4.73), 332sh (4.21), 421 (3.07) nm; MS m/z (rel int) 332 (M+, 66), 302 (10), 288 (17), 286 (10), 242 (23), 168 (43), 140 (100), 139 (72). HRMS m/z Calcd for C19H12N2O4 (M+) 332.0797, found, 332.0773.
Synthesis of N-phenyl- and N-p-methoxyphenyl-1,6-methano[10]annulene-3,4-dicarboximides (1a and 1h) from 3
A solution of 85.0 mg (0.401 mM) of 3 and 41.1 mg (0.441 mM) of aniline in 5 mL of xylene was refluxed on an oil bath for 5 h under nitrogen atmosphere. The solvent was removed under reduced pressure and the residue was purified by column chromatography (CHCl3/hexane = 1/4) to give 37.1 mg (32% yield) of 1a. Compound 1h was obtained similarly with 3 and p-anisidine (49% yield).
1a: Yellow microcrystals, mp 158–159 ºC (lit.,6 170–171 ˚C).13 1H NMR (CDCl3) δ = 8.37 (s, 2H), 7.64 (m, 2H), 7.50 (m, 3H), 7.47 (m, 2H), 7.36 (m, 2H), 0.21 (d, J = 9.8 Hz, 1H), –0.14 (d, J = 9.8 Hz, 1H) ppm; 13C NMR (CDCl3) δ = 169.1, 130.9, 130.1, 129.2, 129.1, 129.0, 128.1, 128.0, 126.5, 119.6, 35.5 ppm; UV-Vis (CH3OH) λmax = 253 (logε = 3.27), 290 (4.71), 327 (2.91), 422 (3.12) nm.
1h: Yellow microcrystals, mp 217–218 ºC. 1H NMR (CDCl3) δ = 8.35 (s, 2H), 7.62 (m, 2H), 7.37 (d, J = 8.8 Hz, 2H), 7.35 (m, 2H), 7.02 (d, J = 8.8 Hz, 2H), 3.85 (s, 3H), 0.21 (d, J = 9.8 Hz, 1H), –0.16 (d, J = 9.8 Hz, 1H) ppm; 13C NMR (CDCl3) δ = 169.3, 159.1, 130.8, 130.0, 129.2, 128.2, 127.8, 124.7, 119.6, 114.4, 55.5, 35.5 ppm; IR (KBr) νmax = 3034w, 1756s, 1705vs, 1508s, 1387s, 1246s, 1154m, 1028m, 827m, 774m, 751m cm–1; UV-Vis (CH3OH) λmax = 229 (logε = 4.33), 289 (4.77), 338 (3.83), 421 (3.11) nm; MS m/z (rel int) 317 (M+, 100), 302 (31), 168 (22), 140 (56), 139 (33). HRMS m/z Calcd for C20H15NO3 (M+) 317.1052, found 317.1049.
Synthesis of N-phenyl- and N-p-methoxyphenyl-1,6-methano[10]annulene-3,4-dicarboximides (1a and 1h) from 1e
A suspension of 106 mg (0.500 mM) of 1e, 112 mg (0.550 mM) of iodobenzene, and 36 mg (0.25 mM) of copper(I) oxide in 5 mL of quinoline was refluxed on an oil bath for 5 h under nitrogen atmosphere. Solids were removed by filtration. The solvent was removed under reduced pressure and the residue was purified by column chromatography (CHCl3/hexane = 1/4) to give 90.4 mg (63% yield) of 1a. By the similar way with p-bromoanisole and 1e, 1h was obtained in 91% yield.
ACKNOWLEDGEMENTS
This research was carried out in part under financial support from Kyowa Hakko Chemical Co. Inc (for S. K). A financial support from the Faculty of Science at Shinshu University (for M.O.) is also acknowledged. We also thank Ms Kanako Tsumuraya and Kimiko Kanayama at University of Toyama for their preliminary research assistance for preparing compounds 1e and 3.
References
1. P. Valat, V. Wintgens, J. Kossanyi, L. Biczók, A. Demeter, and T. Bérces, J. Am. Chem. Soc., 1992, 114, 946; CrossRef A. Demeter, T. Bérces, L. Biczók, V. Wintgens, P. Valat, and J. Kossanyi, J. Phys. Chem., 1996, 100, 2001; CrossRef J. C. Netto-Ferreira, V. Wintgens, L. F. V. Ferreira, A. R. Garcia, L. M. Ilharco, and M. J. Lemos, J. Photochem. Photobio. A: Chem., 2000, 132, 209; CrossRef P. Valat, V. Wintgens, J. Kossanyi, L. Biczók, A. Demeter, and T. Bérces, Helv. Chim. Acta, 2001, 84, 2813; CrossRef G. H. B. Hoa, J. Kossanyi, A. Demeter, L. Biczók, and T. Bérces, Photochem. Photobiol. Sci., 2004, 3, 473. CrossRef
2. Y. Kubo, M. Suto, A. Arai, P. H. Mazzocchi, L. Klingler, D. Shook, and C. Somich, J. Org. Chem., 1986, 51, 4404. CrossRef
3. A. Demeter, L. Biczók, T. Bérces, V. Wintgens, P. Valat, and J. Kossanyi, J. Phys. Chem., 1993, 97, 3217. CrossRef
4. P. Nandhikonda and M. D. Heagy, Org. Lett., 2010, 12, 4796. CrossRef
5. S. Kuroda, T. Kajioka, A. Fukuta, N. C. Thanh, Y. Zhang, R. Miyatake, M. Mouri, S. Zuo, and M. Oda, Mini-Rev. Org. Chem., 2007, 4, 31; CrossRef Y. Zhang, N. Terazawa, Y. Horino, A. Takai, Y. Tsuji, R. Miyatake, M. Oda, and S. Kuroda, Heterocycles, 2009, 77, 241. CrossRef
6. F. Jin, J.-J. Liu, H. Chen, and S. Zuo, Hecheng Huaxue, 2009, 17, 606; F. Jin, J. Liu, H. Chen, and S. Zuo, Beijing Huagong Daxue Xuebao, Ziran Kexueban, 2010, 37, 28.
7. A part of the results in this paper was presented in the 2004th Hokuriku Regional Meeting of Kansai Division of the Chemical Society of Japan; K. Tsumuraya, N. Tamura, M. Nagai, R. Miyatake, M. Kyogoku, and S. Kuroda, 2004, p104 (F-08).
8. Any comment on emission properties of the imides was not reported in ref 6.
9. E. Vogel, R. Feldman, and H. Düwel, Tetrahedron Lett., 1970, 11, 1941; CrossRef E. Vogel, H. M. Deger, J. Somdroek, J. Palm, A. Wagner, and J. Lex, Angew. Chem. Int. Ed. Engl., 1980, 19, 41; CrossRef E. Vogel, Germann Patent 2851790 (1980); E. Vogel, Pure Appl. Chem., 1982, 54, 1015. CrossRef
10. R. Neidlein and G. Schröder, Chem. Ber., 1992, 125, 2225. CrossRef
11. C. R. Ellefson, L. Swenton, R. H. Bile, Jr., and P. M. Green, Tetrahedron, 1976, 32, 1081. CrossRef
12. M. Sato, S. Ebine, and S. Akabori, Synthesis, 1981, 472.
13. Although there is a little difference between melting points of ours and Zuo’s, our 1H and 13C NMR data are identical with those reported by Zuo et el.