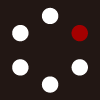
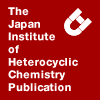
HETEROCYCLES
An International Journal for Reviews and Communications in Heterocyclic ChemistryWeb Edition ISSN: 1881-0942
Published online by The Japan Institute of Heterocyclic Chemistry
e-Journal
Full Text HTML
Received, 12th January, 2011, Accepted, 21st February, 2011, Published online, 1st March, 2011.
DOI: 10.3987/COM-11-12137
■ Synthesis of O2- and N3-(2-Phosphonomethoxy)ethyl Derivatives of 6-Phenyl- and 6-Pyridinyl-5-azacytosine
Miroslav Otmar,* Martin Dracinsky, Marcela Krecmerova, Jan Balzarini, Graciela Andrei, and Robert Snoeck
Gilead Sciences and IOCB Research Centre, Centre for New Antivirals and Antineoplastics, Institute of Organic Chemistry and Biochemistry, Flemingovo nam. 2, 16610 Prague 6, Czech Republic
Abstract
A series of hydrolytically stable O2- and N3-(2-phosphonomethoxy)- ethyl (PME) derivatives of 6-phenyl, pyridin-2, -3 and -4-yl-5-azacytosines was prepared by their alkylation with diisopropyl (2-chloroethoxy)methylphosphonate followed by the deprotection. No antitumor or antiviral activity was found except for 6-(pyridin-4-yl)-1,3,5-triazine-2,4-diamine (13d) which exhibited slight activity against feline herpesvirus in CrFK cell cultures with IC50 = 6.7 µg/mL.INTRODUCTION
5-Azacytosine nucleosides are well-known anticancer agents with an epigenetic mechanism of action.1,2 Two of them, 2’-deoxy-5-azacytidine3-5 (decitabine, Dacogen®, 1; its alpha anomer 2 shows comparable epigenetic activity but with much lower toxicity6-10) and 5-azacytidine10(Vidaza®, 3), were approved for treatment of myelodisplastic syndromes (MDS, Figure 1). Others, i.e. 5-azacytosine arabinoside11 (fazarabine, 4) and 5,6-dihydro-5-azacytidine12,13 (5), are being investigated in clinical trials. On the other hand, 2’-deoxy-5,6-dihydro-5-azacytidine14 (6) exhibits a very strong anti-HIV potency, which enables, especially when its prodrug KP-1461 (7) is applied, the eradication of HIV from laboratory cell cultures.15-17 Compound 6 does not inhibit reverse transcription like other nucleosides or acyclic nucleoside phosphonates, but, after incorporation into proviral DNA, it acts as an ambiguous base, which causes mutations. An accumulation of the errors throughout the viral genome during the replication cycle may lead to a progressive decrease of the fitness of the virus and further to a lethal mutagenesis, which may cause a total collapse of the virus population. Recently, a pronounced antiviral activity has been discovered for the acyclic nucleoside phosphonate HPMP-5-aza-C (8); however, the instability of its 5-azacytosine ring in water solution may substantially compromise the pharmaceutical potential of this compound.18-20 The instability is caused by the electron deficiency in position 6 of the triazine ring, where the imine-resembling carbon could be easily attacked by a nucleophile, e.g. an hydroxyl ion, under the cleavage of the ring, and form an unstable formylcarbamoylguanidine, which spontaneously releases formic acid under the formation of the corresponding carbamoylguanidine.21
To prevent the hydrolytic instability of the 5-azacytosine nucleosides and acyclic nucleoside phosphonates limiting their pharmaceutical potential, we sought a suitable structural modification, which can compensate for the electron deficiency in position 6 through the introduction of an electron-donating substituent. In our previous work, we introduced a C1-C4 alkyl and phenyl substituent into position 6 of HPMP-5-aza-C (8). However, these compounds led to a substantial decrease of the antiviral activity.22 The only exception was the isopropyl ester of the N3-isomer of HPMP-5-aza-6-methyl-C (9), which exhibit moderate anti-RNA-virus activity, whereas the acyclic nucleoside phosphonates of the HPMP structural type are typically active against DNA viruses. Two methods for the preparation of the 6-substituted congeners of HPMP-5-aza-C (8) were used: (1) the condensation of orthoester with guanylurea bearing the HPMP chain and (2) the direct substitution of preformed triazine with the synthon. The direct substitution of 5-azacytosine afforded a mixture of N1-, N3- and O2-derivatives in a 62:14:8 ratio of yields; however, the O2- derivative was not stable enough to survive the subsequent deprotection by trimethylbromosilane. On the other hand, the substitution of 6-methyl-5-azacytosine yielded almost only N3- (46%) with a trace of N1-derivative (6%). The substantial decrease of the substitution rate in the N1-position was ascribed to a steric hindrance caused by the substituent in position 6.
The aim of the present study was to prepare hydrolytically stable acyclic nucleoside phosphonates of 5-azacytidine. To stabilize the triazine ring, we selected aromatic substituents possessing a conjugation effect for position 6: i.e. phenyl, 2-, 3-, and 4-pyridinyl. As the synthetic methodology, we used the direct substitution of the preformed nucleobase, which should provide N3-substituted derivatives resembling compound 9. As the pseudosugar moiety we first chose phosphonomethoxyethyl (PME); the development of other side-chain structural types of these acyclic nucleoside phosphonates is planned.
RESULTS AND DISCUSSION
For the preparations of the 6-aryl-5-azacytosines 10a-d, we used two methods: (1) the reaction of methyl arenecarboxylate 11a-d with carbamoyl guanidine in sodium methoxide solution23 (2) the reaction of pyridinecarbonitriles 12b-d with dicyan diamide in basic conditions,24,25 yielding 2,4-diamino-6-pyridinyl-1,3,5-triazines 13b-d, followed by a treatment with isoamyl nitrite in an acetic acid – water mixture. The diazotation reaction affected selectively only one amino group of triazines 13b-d, affording 6-pyridinyl-5-azacytosines 10b-d. The efficiency of both methods is almost the same. The preformed 6-aryl-5-azacytosines 10a-d were alkylated with diisopropyl (2-chloroethoxy)methylphosphonate (PME-Cl) in DMSO with cesium carbonate as the base at 100 °C, yielding a mixture of O2- and N3-substituted derivatives 14a-d and 15a-d. The yields of the product are rather below-average, amounting to between 10 and 25%; the ratio of the O2- and N3-derivatives varies from 0.7 to 0.9. Our attempts to optimize the reaction conditions were rather unsatisfactory. Prolongation of the reaction time or elevation of the reaction temperature results in a decrease of the yield – significantly for the O2-derivatives until to disappearing and moderately for the N3-derivatives. Addition of the further portion of the alkylating agent and the base did not improve the yield as well. The low overall yields we attributed to the instability of triazine ring itself in the reaction conditions of the base–catalyzed alkylation at elevated temperature. The preferential disappearance of the arisen O2-substituted derivatives, we ascribe to their liability to further react with a nucleophile under cleavage of the enol ether bond. The nucleophile was rather the base itself than the adjacent triazine nitrogen, because no additional formation of the N3-substituted products was observed during the prolongation of reaction time. The higher yields of 6-phenyl derivatives 14a and 15a (21 and 24%) in comparison with 6-pyridyl derivatives 14b-d and 15b-d (10–17%) could be interpreted by a higher electron-donating effect of phenyl, which better stabilizes the triazine ring and the enol ether bond as well. On the other hand, the deprotection of phosphonate diesters 14a-d and 15a-d with bromotrimethylsilane followed by hydrolysis afforded a quantitative yield of phosphonic acids 16a-d and 17a-d. In contrast to the literature22 reporting the total degradation of O2-isomer of HPMP-5-aza-C in the treatment with bromotrimethylsilane, the O2-substituted 6-aryl-5-azacytidine derivatives 16a-d are quite stable under these conditions. Additionally, no decomposition of the resulting compounds 16a-d and 17a-d in aqueous solutions was observed.
The heterocyclic bases, 10b-d and 13b-d and free phosphonic acids, 16a-d and 17a-d were tested for cytostatic activity in CCRF-CEM T-lymphoblastoid cells (human acute lymphoblastic leukemia, ATCC CCL 119), human promyelocytic leukemia HL-60 cells (ATCC CCL 240), human cervix carcinoma HeLa S3 cells (ATCC CCL 2.2), and mouse lymphocytic leukemia L1210 cells (ATCC CCL 219). The antiviral activity of the above-mentioned compounds, except for 10d, 16d, and 17d, was evaluated against various DNA viruses, including poxviruses [i.e. vaccinia virus (VACV)] and herpesviruses [i.e. herpes simplex virus type 1 (HSV-1)] and type 2 (HSV-2), thymidine kinase-deficient HSV-1 (acyclovir-resistant, ACVr), varicella-zoster virus (VZV), human cytomegalovirus (HCMV), and feline herpesvirus], against retroviruses [(i.e. human immunodeficiency virus type 1 (HIV-1) and type 2 (HIV-2)] and Moloney murine sarcoma virus (MSV) (only compounds 13d, 16a,b, 17a,b) and several other RNA viruses, including vesicular stomatitis virus (VSV), Coxsackie B4, respiratory syncytial virus (RSV), parainfluenza virus type 3, reovirus-1, Sindbis virus, Punta Toro virus, feline coronavirus, influenza A virus subtypes H1N1 and H3N2, and influenza B virus. Compounds 10d, 16d, and 17d were tested (considering the earlier negative results in this series) only on a narrower spectrum of viruses – hepatitis C virus, HIV-1 and human respiratory syncytial virus. No antiviral activity was found except for compound 13d, showing activity against the feline herpes virus in Crandell-Rees feline kidney (CrFK) cells at an EC50 = 6.7 µg/mL. However, its antiviral selectivity was rather limited since cell growth was affected at a CC50 = 33 µg/mL, resulting in a selectivity index (ratio CC50/EC50) of 5.
The results showed that alkylation of 6-aryl-5-azacytosines 10a-d with diisopropyl (2-chloroethoxy)methylphosphonate (PME-Cl) proceeded exclusively to positions O2 and N3 and after deprotection afforded the corresponding acyclic nucleoside phosphonates 16a-d and 17a-d, which are, unlike 5-azacytosine nucleosides and nucleoside phosphonates, hydrolytically stable. The O2-derivatives 16a-d could be considered as triazine congeners of the PMEO group of biologically active acyclic nucleoside phosphonates.26 In comparison with HPMP-5-aza-C (8), these structural modifications led to hydrolytically stable compounds, however, they also resulted in a significant loss of antiviral activity.
EXPERIMENTAL
General:
The melting points were determined on a Stuart SMP3 apparatus and are uncorrected. The analytical TLC was performed on silica-gel pre-coated aluminum plates with fluorescent indicator (Merck 5554, 60 F254). The spots were visualized with UV light (254 nm) or by spraying with ninhydrin (1% solution in ethanol) followed by a short heating to 300–400 °C. The column chromatography was conducted on silica gel (Sigma S 0507, 40–63 μm). The mass spectra were measured on a Q-Tof micro (Waters) and the HR MS were taken on a LTQ Orbitrap XL (Thermo Fisher Scientific) spectrometer. The 1H and 13C NMR spectra were recorded on a Bruker Avance 400 (1H at 400 MHz and 13C at 100.6 MHz), Bruker Avance 500 (1H at 500 MHz and 13C at 125.7 MHz), and Bruker Avance 600 (1H at 600.1 MHz and 13C at 150.9 MHz) in CDCl3 (referenced to TMS as an internal standard), in DMSO-d6 (referenced to the solvent signal δ = 2.50 and 39.70 ppm, respectively), or in D2O (referenced to dioxane as an internal standard). The complete assignment was based on the heteronuclear correlation experiments (HSQC and H,C-HMBC). The chemical shifts (δ) are in ppm and the coupling con¬stants (J) in Hz. The UV spectra were taken on a Beckman DU-65 spectrophotometer in methanol solution. The IR spectra were obtained on an FT IR Bruker Equinox IFS 55 spectro¬meter in chloroform or KBr pellets. The elemental analyses were performed on a PE 2400 Series II CHNS/O Analyzer (Perkin Elmer, USA).
General procedures:
6-Pyridinyl-1,3,5-triazine-2,4-diamines (13b-d)
Pyridine carbonitrile 12b-d (10 mmol), 2-cyanoguanidine (10 mmol) and KOH (500 mg) in 2-methoxyethanol (50 mL) were heated to 125 °C for 20 min. The solvent was evaporated and the residue was crystallized from MeOH, affording 13b-d as white crystals in a 90–98% yield. Compound 13d had been prepared in an alternative way previously.24
4-Amino-6-aryl-1,3,5-triazin-2-oles (10a-d)
Method A: Compound 10a had been prepared in this way previously.23 Methyl arenecarboxylate 11a-d (10 mmol), carbamoyl guanidine (10 mmol) and sodium methoxide (10 mmol) were refluxed in methanol for 7 h. The reaction mixture was acidified with acetic acid and evaporated. The residue was crystallized from water and MeOH affording 10a-d as white hygroscopic powder (at >250 °C decomposition without melting) in a yield of 42–48%.
Method B: Isoamyl nitrite (50 mmol) was added to a solution of 6-(pyridinyl)-1,3,5-triazine-2,4-diamine 13b-d (10 mmol) in an acetic acid – water (3:1) mixture at 0 °C, and the mixture was kept at 0 °C for 4 h and then at room temperature for 2 days. The solvent was evaporated, the residue co-evaporated with water then desalted on the Dowex 50 (H+). Crystallization from the water–MeOH mixture afforded compounds 10b-d as white hygroscopic powder (at >250 °C decomposition without melting) in a yield of 55–68%.
Diisopropyl (2-(4-amino-6-aryl-1,3,5-triazin-2-yloxy)ethoxy)methylphosphonates (14a-d)
Diisopropyl (2-(6-amino-2-oxo-4-aryl-1,3,5-triazin-1(2H)-yl)ethoxy)methylphosphonates (15a-d)
4-Amino-6-aryl-1,3,5-triazin-2-ol 10a-d (4 mmol), cesium carbonate (10 mmol), and diisopropyl (2-chloroethoxy)methylphosphonate (PME-Cl, 6 mmol) in DMSO (25mL) were heated to 100 °C for 4 h. The reaction mixture was subsequently applied on the Dowex 50 (H+) column and washed with water and then with aqueous ammonia. The ammonia wash was evaporated. The chromatography on the silica gel column (CHCl3–MeOH (96:4) followed by crystallization (hexanes–EtOAc) afforded the O-substituted product 14a-d (10–21%) and N-substituted product 15a-d, both as white crystals.
(2-(4-Amino-6-aryl-1,3,5-triazin-2-yloxy)ethoxy)methylphosphonic acid ammonium salt (16a-d)
(2-(6-Amino-2-oxo-4-aryl-1,3,5-triazin-1(2H)-yl)ethoxy)methylphosphonic acid ammonium salt (17a-d)
Bromotrimethylsilane (10 mmol) was added to a solution/suspension of diisopropyl phosphonate (1 mmol) in MeCN (5 mL), and the reaction mixture was kept at room temperature for overnight. The volatiles were evaporated, then aqueous ammonia was added and the mixture was evaporated to dryness. The product was purified on a Dowex 1x2 column with a gradient of 0–0.75 M AcOH, the appropriate fractions were collected and evaporated, the traces of acetic acid were removed by co-evaporation with water, then aqueous ammonia was added and the solution was evaporated to dryness. Crystallization from a MeOH–EtOAc mixture gave the ammonium salt of the free acids 16a-d and 17a-d in a yield of 80–88% as a white powder with an indefinite melting point.
6-(Pyridin-2-yl)-1,3,5-triazine-2,4-diamine (13b)
Yield: 90%, white powder. MS (ESI) m/z (%): 189 (100, M+H), 211 (84, M+Na). 1H NMR (DMSO-d6) δ: 8.69 (ddd, 1H, J(6’,5’)=4.7 Hz, J(6’,4’)=1.8 Hz, J(6’,3’)=0.9 Hz, H-6’), 8.20 (dt, 1H, J(3’,4’)=7.9 Hz, J(3’,5’)= J(3’,6’)=1.1 Hz, H-3’), 7.9 (td, 1H, J(4’,3’)= J(4’,5’)=7.8 Hz, J(4’,6’)=1.8 Hz, H-4’), 7.49 (ddd, 1H, J(5’,4’)=7.5 Hz, J(5’,6’)=4.7 Hz, J(5’,3’)=1.2 Hz, H-5’), 7.05 (br s, 2H, NH2), 6.85 (br s, 2H, NH2). 13C NMR (DMSO-d6) δ: 170.48 (C-6), 167.83 (2C, C-2 and C-4), 154.87 (C-2’), 149.48 (C-6’), 136.91 (C-4’), 125.50 (C-5’), 123.33 (C-3’). FTIR (KBr, cm-1) ν: 3464, 3398, 3289, 3168, 1670, 1616, 1577, 1544, 1498, 1449, 1398, 1254, 997, 832, 794. UV (H2O) λmax (ε): pH=2: 275 (20), pH=7: 269 (17.5), pH=12: 269 (13.6). Anal. Calcd for C8H8N6: C, 51.06; H, 4.28; N, 44.66. Found: C, 51.02; H, 4.26; N, 44.69.
6-(Pyridin-3-yl)-1,3,5-triazine-2,4-diamine (13c)
Yield: 97%, white powder. MS (ESI) m/z (%): 189 (100, M+H), 211 (81, M+Na). 1H NMR (DMSO-d6) δ: 9.34 (dd, 1H, J(2’,4’)=2.2 Hz, J(2’,5’)=0.9 Hz, H-2’), 8.86 (dd, 1H, J(6’,5’)=4.8 Hz, J(6’,4’)=1.8 Hz, H-6’), 8.48 (ddd, 1H, J(4’,5’)=8.0 Hz, J(4’,2’)=2.2 Hz, J(4’,6’)=1.8 Hz, H-4’), 7.50 (ddd, 1H, J(5’,4’)=8.0 Hz, J(5’,6’)=4.8 Hz, J(5’,2’)=0.9 Hz, H-5’), 6.86 (br s, 4H, NH2). 13C NMR (DMSO-d6) δ: 168.99 (C-6), 167.48 (C-2 and C-4), 151.88 (C-6’), 149.17 (C-2’), 135.20 (C-4’), 132.66 (C-3’), 123.66 (C-5’). FTIR (KBr, cm-1) ν: 3466, 3399, 3311, 3195, 1631, 1591, 1545, 1496, 1449, 1393, 807. UV (MeOH) λmax (ε): 267 (7.0). Anal. Calcd for C8H8N6: C, 51.06; H, 4.28; N, 44.66. Found: C, 51.13; H, 4.28; N, 44.47.
6-(Pyridin-4-yl)-1,3,5-triazine-2,4-diamine24 (13d)
Yield: 98%, white powder. MS (ESI) m/z (%): 189 (100, M+H). 1H NMR (DMSO-d6) δ: 8.71 (8.71 m, 2H, H-2’), 8.07 (m, 2H, H-3’), 6.95 (br s, 4H, NH2). 13C NMR (DMSO-d6) δ: 168.97 (C-6), 167.67 (C-2 or C-4), 150.29 (C-2’), 144.72 (C-4’), 121.73 (C-3’). FTIR (KBr, cm-1) ν: 3386, 3332, 3157, 1679, 1646, 1628, 1575, 1508, 1496, 1445, 1398, 1064, 809. UV (MeOH) λmax (ε): 271 (7.0). Anal. Calcd for C8H8N6: C, 51.06; H, 4.28; N, 44.66. Found: C, 50.98; H, 4.37; N, 44.89.
4-Amino-6-(pyridin-2-yl)-1,3,5-triazin-2-ol (10b)
Yield: 42% (method A), 55% (method B), white hygroscopic powder. MS (ESI) m/z (%): 190 (100, M+H), 379 (68, 2M+H). HRMS (ESI) calcd. for C8H8N5O (M+H): 190.0723, found: 190.0723. 1H NMR (DMSO-d6) δ: 9.86 (br s, 1H, NH2), 8.84 (ddd, 1H, J(6’,5’)=4.7 Hz, J(6’,4’)=1.7 Hz, J(6’,3’)=0.9 Hz, H-6’), 8.73 (br s, 1H, NH2), 8.32 (dt, 1H, J(3’,4’)=7.9 Hz, J(3’,5’)= J(3’,6’)=1.1 Hz, H-3’), 8.18 (td, 1H, J(4’,3’)= J(4’,5’)=7.8 Hz, J(4’,6’)=1.7 Hz H-4’), 7.81 (ddd, 1H, J(5’,4’)=7.7 Hz, J(5’,6’)=4.8 Hz, J(5’,3’)=1.2 Hz, H-5’). 13C NMR (DMSO-d6) δ: 163.86 (C-6), 160.20 (C-2 or C-4), 149.96 (C-6’), 148.23 (C-2 or C-4), 146.57 (C-2’), 138.93 (C-4’), 129.32 (C-5’), 124.86 (C-3’). FTIR (KBr, cm-1) ν: 3337, 3148, 1694, 1615, 1556, 1469, 1389. UV (H2O) λmax (ε): pH=2: 279 (12.0), pH=7: 275 (9.2), pH=12: 266 (10.0).
4-Amino-6-(pyridin-3-yl)-1,3,5-triazin-2-ol (10c)
Yield: 48% (method A), 68% (method B), white hygroscopic powder. MS (ESI) m/z (%): 190 (100, M+H). HRMS (ESI) calcd. for C8H8N5O (M+H): 190.0723, found: 190.0723. 1H NMR (DMSO-d6) δ: 9.59 (br s, 1H, NH2), 9.27 (dd, 1H, J(2’,4’)=2.3 Hz, J(2’,5’)=0.9 Hz, H-2’), 8.94 (dd, 1H, J(6’,5’)=5.1 Hz, J(3’,5’)= J(6’,4’)=1.6 Hz, H-6’), 8.60 (ddd, 1H, J(4’,5’)=8.1 Hz, J(4’,2’)=2.3 Hz, J(4’,6’)=1.6 Hz, H-4’), 8.44 (br s, 1H, NH2), 7.81 (ddd, 1H, J(5’,4’)=8.2 Hz, J(5’,6’)=5.1 Hz, J(5’,2’)=0.8 Hz, H-5’). 13C NMR (DMSO-d6) δ: 165.63 (C-6), 159.87 (C-2 or C-4), 152.47 (C-6’), 150.30 (C-2 or C-4), 148.10 (C-2’), 138.74 (C-4’), 128.21 (C-3’), 125.03 (C-5’). FTIR (KBr, cm-1) ν: 3314, 3064, 1637, 1554, 1495, 1385, 1295, 800. UV (MeOH): 268 (7.7).
4-Amino-6-(pyridin-4-yl)-1,3,5-triazin-2-ol (10d)
Yield: 47% (method A), 59% (method B), white hygroscopic powder. MS (ESI) m/z (%): 190 (100, M+H). HRMS (ESI) calcd. for C8H8N5O (M+H): 190.0723, found: 190.0723. 1H NMR (DMSO-d6) δ: 8.73 (m, 2H, H-2’), 8.05 (m, 2H, H-3’), 7.46 (bs, 2H, NH2). 13C NMR (DMSO-d6) δ: 169.35 (C-6), 162.25 (C-2 or C-4), 156.69 (C-2 or C-4), 150.01 (C-2’), 142.84 (C-4’), 121.73 (C-3’). FTIR (KBr, cm-1) ν: 3385, 3310, 3128, 2749, 1688, 1643, 1573, 1547, 1463, 1412, 1389, 982, 798. UV (H2O) λmax (ε): pH=2: 271 (7.0), pH=7: 272 (7.2), pH=12: 268 (6.0).
Diisopropyl (2-(4-amino-6-phenyl-1,3,5-triazin-2-yloxy)ethoxy)methylphosphonate (14a)
Yield: 21%, white crystals, mp 97-99 °C. MS (FAB) m/z (%): 411 (31, M+H), 139 (100, base+H). 1H NMR (DMSO-d6) δ: 8.39 (m, 2H, H-2’), 7.53 (m, 1H, H-4’), 7.46 (m, 2H, H-3’), 5.55 (br s, 2H, NH2), 4.76 (dn, 2H, J(H-C-O-P)=7.6 Hz, J(CH,CH3)=6.2 Hz, CH(CH3)2), 4.62 (m, 2H, H-1’’), 3.99 (m, 2H, H-2’’), 3.86 (d, 2H, J(CH,P)=8.2 Hz, OCH2P), 1.33 (d, 6H, J(CH3,CH)=6.2, CH3-iPr), 1.32 (d, 6H, J(CH3,CH)=6.2, CH3-iPr). 13C NMR (DMSO-d6) δ: 173.56 (C-6), 171.24 (C-2), 168.68 (C-4), 135.68 (C-1’), 132.16 (C-4’), 128.61 (2C, C-2’), 128.34 (2C, C-3’), 71.14 (d, J(C-O-P)=6.6 Hz, CH(CH3)2), 71.02 (d, J(2’’,P)=10.9 Hz), 66.14 (d, J(C,P)=167.3 Hz, OCH2P), 66.06 (C-1’’), 24.07 (d, 2C, J(C-C-O-P)=3.4 Hz, (CH3)2CH), 23.95 (d, 2C, J(C-C-O-P)=4.2 Hz, (CH3)2CH). FTIR (CHCl3, cm-1) ν: 3544, 3427, 2985, 1610, 1556, 1543, 1412, 1399, 1342, 997. UV (MeOH) λmax (ε): 250 (20.6). Anal. Calcd for C18H27N4O5P: C, 52.68; H, 6.63; N, 13.65; P, 7.55. Found: C, 52.45; H, 6.62; N, 13.43; P, 7.44.
Diisopropyl (2-(4-Amino-6-(pyridin-2-yl)-1,3,5-triazin-2-yloxy)ethoxy)methylphosphonate (14b)
Yield: 10%, white crystals, mp 116-117 °C. MS (ESI) m/z (%): 412 (26, M+H), 434 (100, M+Na). 1H NMR (DMSO-d6) δ: 8.71 (ddd, 1H, J(6’,5’)=4.7 Hz, J(6’,4’)=1.8 Hz, J(6’,3’)=0.9 Hz,H-6’), 8.28 (dt, 1H, J(3’,4’)=7.9 Hz, J(3’,5’)= J(3’,6’)=1.1 Hz, H-3’), 7.96 (td, 1H, J(4’,3’)= J(4’,5’)=7.8 Hz, J(4’,6’)=1.8 Hz, H-4’), 7.75 (br s, 1H, NH2), 7.57 (br s, 1H, NH2), 7.55 (ddd, 1H, J(5’,4’)=7.6 Hz, J(5’,6’)=4.7 Hz, J(5’,3’)=1.2 Hz, H-5’), 4.58 (dn, 2H, J(HC,OP)=7.8 Hz, J(CH,CH3)=6.2, CH-iPr), 4.50 (m, 2H, H-1’’), 3.86 (m, 2H, H-2’’), 3.83 (d, 2H, J(HC,P)=8.3 Hz, OCH2P), 1.22 (d, 6H, J(CH3,CH)=6.2, CH3-iPr), 1.21 (d, 6H, J(CH3,CH)=6.2, CH3-iPr). 13C NMR (DMSO-d6) δ: 172.00 (C-6), 171.21 (C-2), 169.11 (C-4), 153.73 (C-2’), 149.72 (C-6’), 137.19 (C-4’), 126.17 (C-5’), 123.89 (C-3’), 70.71 (d, J(2’’,P)=12.0 Hz, C-2’’), 70.42 (d, 2C, J(C-O-P)=6.4 Hz, CH-iPr), 65.60 (C-1’’), 65.08 (d, J(C,P)=164.4 Hz, OCH2P), 24.03 (d, 2C, J(C-C-O-P)=3.7 Hz, CH3-iPr), 23.89 (d, 2C, J(C-C-O-P)=4.5 Hz, CH3-iPr). Anal. Calcd for C17H26N5O5P: C, 49.63; H, 6.37; N, 17.02; P, 7.53. Found: C, 49.50; H, 6.36; N, 17.02; P, 7.65.
Diisopropyl (2-(4-Amino-6-(pyridin-3-yl)-1,3,5-triazin-2-yloxy)ethoxy)methylphosphonate (14c)
Yield: 13%, white crystals, mp 104-105 °C. MS (ESI) m/z (%): 412 (39, M+H), 434 (97, M+Na), 845 (100, 2M+Na). 1H NMR (DMSO-d6) δ: 9.39 (dd, 1H, J(2’,4’)=2.2 Hz, J(2’,5’)=0.9 Hz, H-2’), 8.75 (dd, 1H, J(6’,5’)=4.8 Hz, J(6’,4’)=1.8 Hz, H-6’), 8.55 (dt, 1H, J(4’,5’)=8.0 Hz, J(4’,2’)=J(4’,6’)=2.0 Hz, H-4’), 7.74 (br s, 1H, NH2), 7.67 (br s, 1H, NH2), 7.55 (ddd, 1H, J(5’,4’)=8.0 Hz, J(5’,6’)=4.8 Hz, J(5’,2’)=0.9 Hz, H-5’), 4.58 (dn, 2H, J(HC,OP)=7.8 Hz, J(CH,CH3)=6.2, CH-iPr), 4.49 (m, 2H, H-1’’), 3.85 (m, 2H, H-2’’), 3.82 (d, 2H, J(HC,P)=8.4 Hz, CH2P), 1.22 (d, 6H, J(CH3,CH)=6.2, CH3-iPr), 1.22 (d, 6H, J(CH3,CH)=6.2, CH3-iPr). 13C NMR (DMSO-d6) δ: 170.91 (C-2 or C-6), 170.98 (C-2 or C-6), 168.67 (C-4), 152.73 (C-6’), 149.41 (C-2’), 135.64 (C-4’), 131.62 (C-3’), 123.94 (C-5’), 70.67 (d, J(2’’,P)=12.0 Hz, C-2’’), 70.42 (d, 2C, J(C-O-P)=6.4 Hz, CH-iPr), 65.65 (C-1’’), 65.04 (d, J(C,P)=164.0 Hz, CH2P), 24.04 (d, 2C, J(C-C-O-P)=3.7 Hz, CH3-iPr), 23.90 (d, 2C, J(C-C-O-P)=4.5 Hz, CH3-iPr). FTIR (KBr, cm-1) ν: 3315, 3118, 2979, 1669, 1596, 1589, 1548, 1513, 1414, 1405, 1385, 1341, 1316, 1253, 1007, 973. UV (MeOH) λmax (ε): 267 (7.6), 225 (20.5). Anal. Calcd for C17H26N5O5P: C, 49.63; H, 6.37; N, 17.02; P, 7.53. Found: C, 49.59; H, 6.34; N, 17.00; P, 7.80.
Diisopropyl (2-(4-amino-6-(pyridine-4-yl)-1,3,5-triazin-2-yloxy)ethoxy)methylphosphonate (14d)
Yield: 11%, white crystals, mp 103-104 °C. MS (ESI) m/z (%): 412 (100, M+H), 434 (98, M+Na). 1H NMR (DMSO-d6) δ: 8.75 (m, 2H, H-2’), 8.12 (m, 2H, H-3’), 7.75 (1H, bs, NH2), 7.69 (1H, bs, NH2), 4.58 (dn, 2H, J(H-C-O-P)=7.8 Hz, J(CH-CH3=6.2 Hz, P-O-CH), 4.50 (m, 2H, H-1’’), 3.86 (m, 2H, H-2’’), 3.82 (d, 2H, J(H-C-P)=8.3 Hz, 1.21 (d, 6H, J(CH3-CH)=6.2 Hz, CH3), 1.20 (d, 6H, J(CH3-CH)=6.2 Hz, CH3). 13C NMR (DMSO-d6) δ: 171.17 (C-2), 170.75 (C-6), 168.89 (C-4), 150.63 (C-2’), 143.51 (C-4’), 121.89 (C-3’), 70.67 (d, J(2’’-P)=12.0 Hz, C-2’’), 70.48 (d, J(C-O-P)=6.3 Hz, CH(CH3)2), 65.77 (C-1’’), 65.09 (d, J(C-P)=164.9 Hz, CH2P), 24.03 (d, J(C-C-O-P)=3.6 Hz, CH(CH3)2), 23.90 (d, J(C-C-O-P)=4.3 Hz, CH(CH3)2). FTIR (KBr, cm-1) ν: 3332, 2981, 1667, 1645, 1586, 1547, 1423, 1396, 1342, 1241, 1003, 991, 814. UV (MeOH) λmax (ε): 271 (3.8). Anal. Calcd for C17H26N5O5P: C, 49.63; H, 6.37; N, 17.02; P, 7.53. Found: C, 49.82; H, 6.48; N, 16.80; P, 7.39.
Diisopropyl (2-(6-amino-2-oxo-4-phenyl-1,3,5-triazin-1(2H)-yl)ethoxy)methylphosphonate (15a)
Yield: 24%, white crystals, 177-178 °C. MS (FAB) m/z (%): 411 (100, M+H). 1H NMR (DMSO-d6) δ: 8.39 (m, 2H, H-2’), 7.52 (m, 1H, H-4’), 7.44 (m, 2H, H-3’), 4.73 (dn, 2H, J(H-C-O-P)=7.6 Hz, J(CH-CH3)=6.2 Hz, P-OCH), 4.28 (m, 2H, H-1’’), 3.99 (m, 2H, H-2’’), 3.78 (d, 2H, J(H-C-P)=8.4 Hz, H-3’’), 1.33 (d, 6H, J(CH3-CH)=6.2 Hz, CH3), 1.32 (d, 6H, J(CH3-CH)=6.2 Hz, CH3). 13C NMR (DMSO-d6) δ: 171.70 (C-4), 161.56 (C-6), 156.80 (C-2), 135.64 (C-1’), 132.27 (C-4’), 129.13 (2C, C-2’), 128.17 (2C, C-3’), 72.40 (d, J(2’’-P)=10.9 Hz, C-2’’), 71.56 (d, 2C, J( C-O-P)=6.7 Hz, P-O-CH), 66.43 (d, J(C-P)=168.0 Hz, C-3’’), 44.47 (C-1’’), 24.00 (4C, m, CH3). FTIR (CHCl3, cm-1) ν: 3474, 3341, 3222, 3002, 2986, 1684, 1624, 1559,1472, 1401, 1420, 1401, 1246, 1103, 998. UV (MeOH) λmax (ε): 255 (23.6). Anal. Calcd for C18H27N4O5P: C, 52.68; H, 6.63; N, 13.65; P, 7.55. Found: C, 52.45; H, 6.65; N, 13.39; P, 7.41.
Diisopropyl (2-(6-amino-2-oxo-4-(pyridin-2-yl)-1,3,5-triazin-1(2H)-yl)ethoxy)methylphosphonate (15b)
Yield: 11%, white crystals, 168-169 °C. MS (ESI) m/z (%): 412 (37, M+H), 434 (19, M+Na), 845 (100, 2M+Na). 1H NMR (DMSO-d6) δ: 8.68 (ddd, 1H, J(6’,5’)=4.7 Hz, J(6’,4’)=1.8 Hz, J(6’,3’)=0.9 Hz, H-6’), 8.21 (dt, 1H, J(3’,4’)=7.9 Hz, J(3’,5’)=J(3’,6’)= 1.1 Hz, H-3’), 7.92 (td, 1H, J(4’,3’)=J(4’,5’)=7.7 Hz, J(4’,6’)=1.8 Hz, H-4’), 7.52 (ddd, 1H, J(5’,4’)=7.6 Hz, J(5’,6’)=4.7 Hz, J(5’,3’)=1.2 Hz, H-5’), 4.55 (dn, 2H, J(HC,OP)=7.7 Hz, J(CH,CH3)=6.2 Hz, CH-iPr), 4.14 (t, 2H, J(1’’,2‘’)=5.6 Hz, H-1’’), 3.79 (d, 2H, J(HC,P)=8.2 Hz, OCH2P), 3.78 (t, 2H, J(2’’,1’’)=5.6 Hz, H-2’’), 1.19 (d, 6H, J(CH3,CH)=6.2, CH3-iPr), 1.17 (d, 6H, J(CH3,CH)=6.2, CH3-iPr). 13C NMR (DMSO-d6) δ: 169.97 (C-4), 160.89 (C-2 or C-6), 156.27 (C-2 or C-6), 154.09 (C-2’), 149.46 (C-6’), 137.01 (C-4’), 125.98 (C-5’), 123.90 (C-3’), 70.50 (d, 2C, J(C-O-P)=6.4 Hz, CH-iPr), 69.00 (d, J(2’’,P)=11.3 Hz, C-2’’), 65.13 (d, J(C,P)=163.5 Hz, CH2P), 41.48 (C-1’’), 24.01 (d, 2C, J(C-C-O-P)=3.7 Hz, CH3-iPr), 23.89 (d, 2C, J(C-C-O-P)=4.5 Hz, CH3-iPr). FTIR (KBr, cm-1) ν: UV (MeOH) λmax (ε): Anal. Calcd for C17H26N5O5P: C, 49.63; H, 6.37; N, 17.02; P, 7.53. Found: C, 49.44; H, 6.41; N, 16.82; P,7.61.
Diisopropyl (2-(6-amino-2-oxo-4-(pyridin-3-yl)-1,3,5-triazin-1(2H)-yl)ethoxy)methylphosphonate (15c)
Yield: 17%, white crystals, 145-146 °C. MS (ESI) m/z (%): 412 (24, M+H), 434 (100, M+Na), 845 (44, 2M+Na). 1H NMR (DMSO-d6) δ: 9.33 (dd, 1H, J(2’,4’)=2.2 Hz, J(2’,5’)=0.9 Hz, H-2’), 8.72 (dd, 1H, J(6’,5’)=4.8 Hz, J(6’,4’)=1.8 Hz, H-6’), 8.48 (ddd, 1H, J(4’,5’)=8.0 Hz, J(4’,2’)= 2.2 Hz, J(4’,6’)=1.8 Hz, H-4’), 8.47 (bs, 1H, NH2), 7.87 (bs, 1H, NH2), 7.53 (ddd, 1H, J(5’,4’)=8.0 Hz, J(5’,6’)=4.8 Hz, J(5’,2’)=0.9 Hz, H-5’), 4.54 (dn, 2H, J(HC,OP)=7.7 Hz, J(CH,CH3)=6.2 Hz, CH-iPr), 4.13 (t, 2H, J(1’’,2‘’)=5.5 Hz, H-1’’), 3.79 (d, 2H, J(HC,P)=8.1 Hz, CH2P), 3.77 (t, 2H, J(2’’,1’’)=5.6 Hz,H-2’’), 1.19 (d, 6H, J(CH3,CH)=6.2, CH3-iPr), 1.17 (d, 6H, J(CH3,CH)=6.2, CH3-iPr). 13C NMR (DMSO-d6) δ: 168.69 (C-4), 160.45 (C-2 or C-6), 155.87 (C-2 or C-6), 152.56 (C-6’), 149.57 (C-2’), 135.81 (C-4’), 131.91 (C-3’), 123.79 (C-5’), 70.45 (d, 2C, J(C-O-P)=6.4 Hz, CH-iPr), 68.96 (d, J(2’’,P)=11.4 Hz, C-2’’), 65.08 (d, J(C,P)=163.5 Hz, CH2P), 41.48 (C-1’’), 24.01 (d, 2C, J(C-C-O-P)=3.7 Hz, CH3-iPr), 23.88 (d, 2C, J(C-C-O-P)=4.5 Hz, CH3-iPr). FTIR (KBr, cm-1) ν: 3322, 3062, 2982, 1693, 1668, 1638, 1585, 1536, 1495, 1467, 1460, 1386, 1243, 997, 801. UV (MeOH) λmax (ε): 268 (8.4), 232 (14.6). Anal. Calcd for C17H26N5O5P: C, 49.63; H, 6.37; N, 17.02; P, 7.53. Found: C, 49.59; H, 6.36; N, 16.90; P, 7.75.
Diisopropyl (2-(6-amino-2-oxo-4-(pyridin-4-yl)-1,3,5-triazin-1(2H)-yl)ethoxy)methylphosphonate (15d)
Yield: 16%, white crystals, mp 157-158 °C. MS (ESI) m/z (%): 412 (100, M+H), 434 (64, M+Na). 1H NMR (DMSO-d6) δ: 8.73 (m, 2H, H-2’), 8.51 (bs, 1H, NH2), 8.05 (m, 2H, H-3’), 7.91 (bs, 1H, NH2), 4.53 (dn, 2H, J(H-C-O-P)=7.6 Hz, J(CH-CH3)=6.0 Hz, 4.14 (t, 2H, J(1’’,2’’)=5.5 Hz, H-1’’), 3.78 (d, 2H, J(H-C-P)=8.00 Hz, CH2P), 3.77 (d, 2H, J(2’’,1’’)=5.5 Hz, H-2’’), 1.18 (d, 6H, J(CH3-CH)=6.2 Hz, CH3-CH), 1.16 (d, 6H, J(CH3-CH)=6.2 Hz, CH3-CH). 13C NMR (DMSO-d6) δ: 168.61 (C-4), 160.72 (C-2 or C-6), 156.06 (C-2 or C-6), 150.47 (2C, C-2’), 143.99 (C-4’), 122.06 (2C, C-3’), 70.52 (d, J(C-O-P)=6.3 Hz, CH-O-P), 68.95 (d, J(2’’,P)=11.1 Hz, H-2’’), 65.13 (d, J(C-P)=163.7 Hz, CH2P), 41.64 (C-1’’), 24.00 (d, J(C-C-O-P)=3.5 Hz, CH(CH3)2), 23.88 (d, J(C-C-O-P)=4.4 Hz, CH(CH3)2). FTIR (KBr, cm-1) ν: 3338, 3167, 2980, 1699, 1681, 1652, 1576, 1542, 1475, 1445, 1392, 1242, 1005, 986. UV (MeOH) λmax (ε): 275 (6.4). Anal. Calcd for C17H26N5O5P: C, 49.63; H, 6.37; N, 17.02; P, 7.53. Found: C, 49.82; H, 6.48; N, 16.90; P, 7.29.
(2-(4-Amino-6-phenyl-1,3,5-triazin-2-yloxy)ethoxy)methylphosphonic acid ammonium salt (16a)
Yield: 88%. MS (ESI-) m/z (%): 325 (100, M-H). HRMS (ESI) calcd. for C12H14N4O5P (M-H): 325.0707, found: 325.0708. 1H NMR (D2O) δ: 8.00 (m, 2H, H-2’), 7.58 (m, 1H, H-4’), 7.46 (m, 2H, H-3’), 4.46 (m, 2H, H-1’’), 3.93 (m, 2H, H-2’’), 3.74 (m, 2H, CH2P). 13C NMR (D2O) δ: 174.07 (C-6), 171.05 (C-2), 168.60 (C-4), 135.18 (C-1’), 133.25 (C-4’), 129.24 (2C, C-2’ or C-3’), 128.89 (2C, C-2’ or C-3’), 71.10 (d, J(2’’,P)=10.5 Hz, C-2’’), 67.75 (d, J(C-P)=156.1 Hz, CH2P), 67.02 (C-1’’). FTIR (KBr, cm-1) ν: 3461, 3318, 3167, 1642, 1594, 1566, 1529, 1401, 1338, 1163, 1044, 942. UV (H2O) λmax (ε): pH=2: 255 (13.0), pH=7: 251 (13.8), pH=12: 251 (16.5).
(2-(4-Amino-6-(pyridin-2-yl)-1,3,5-triazin-2-yloxy)ethoxy)methylphosphonic acid ammonium salt (16b)
Yield: 85%. MS (ESI-) m/z (%): 326 (74, M-H), 653 (100, 2M-H). MS (ESI) m/z (%): 328 (10, M+H), 350 (65, M+Na), 372 (100, M+2Na-H), 394 (49, M+3Na-2H). HRMS (ESI) calcd. for C11H15N5O5P (M+H): 328.0805, found: 328.0803; calcd. for C11H14N5O5NaP (M+Na): 350.0625, found: 350.0624.1H NMR (D2O) δ: 8.63 (m, 1H, H-6’), 8.20 (bd, 1H, (3’,4’) = 7.8 Hz, H-3’), 7.99 (bt, 1H, J(4’,3’)= J(4’,5’) = 7.7 Hz, H-4’), 7.62 (m, 1H, H-5’), 4.57 (m, 2H, H-1’’), 3.97 (m, 2H, H-2’’), 3.74 (m, 2H, OCH2P). 13C NMR (D2O) δ: 172.20 (C-6), 171.38 (C-2), 168.84 (C-4), 151.71 (C-2’), 149.61 (C-6’), 139.26 (C-4’), 127.86 (C-5’), 125.02 (C-3’), 71.10 (d, J(2’’,P) = 10.7 Hz, C-2’’), 67.68 (d, J(C,P) = 155.9 Hz, OCH2P), 67.30 (C-1’).
(2-(4-Amino-6-(pyridin-3-yl)-1,3,5-triazin-2-yloxy)ethoxy)methylphosphonic acid ammonium salt (16c)
Yield: 85%. MS (ESI-) m/z (%): 326 (100, M-H), 653 (48, 2M-H). MS (ESI) m/z (%): 328 (13, M+H), 350 (72, M+Na), 372 (100, M+2Na-H), 394 (53, M+3Na-2H). HRMS (ESI) calcd. for C11H15N5O5P (M+H): 328.0805, found: 328.0804; calcd. for C11H14N5O5NaP (M+Na): 350.0625, found: 350.0625. 1H NMR (500 MHz, D2O) δ: 8.98 (dd, 1H, J(2’,4’)=2.2 Hz, J(2’,5’)=0.8 Hz, H-2’), 8.57 (dd, 1H, J(6’,5’)=5.0 Hz, J(6’,4’)=1.7 Hz, H-6’), 8.32 (ddd, 1H, J(4’,5’)=8.1 Hz, J(4’,2’)=2.2 Hz, J(4’,6’)=1.7 Hz, H-4’), 7.45 (ddd, 1H, J(5’,4’)=8.1 Hz, J(5’,6’)=2.2 Hz, J(5’,2’)=1.7 Hz, H-5’), 4.48 (m, 2H, H-1’’), 3.94 (m, 2H, H-2’’), 3.64 (d, 2H, J(CH2,P)=8.5 Hz, CH2-P). 13C NMR (125.7 MHz, D2O) δ: 171.63 (C-2 or C-6), 170.93 (C-2 or C-6), 168.43 (C-4), 152.20 (C-6’), 148.87 (C-2’), 137.42 (C-4’), 131.39 (C-3’), 124.70 (C-5’), 70.86 (d, J(2’’,P)=10.5 Hz, C-2’’), 69.0 (d, J(CH2,P)=152.0 Hz, CH2-P), 67.35 (C-1’’). FTIR (KBr, cm-1) ν: 3446, 3344, 3241, 2875, 1636, 1598, 1588, 1560, 1538, 1436, 1416, 1338, 1074, 1055, 965, 810. UV (H2O) λmax (ε): pH=2: 264 (125), pH=7: 265 (390), pH=12: 265 (260).
(2-(4-Amino-6-(pyridin-3-yl)-1,3,5-triazin-2-yloxy)ethoxy)methylphosphonic acid ammonium salt (16d)
Yield: 80%. MS (ESI-) m/z (%): 326 (100, M-H). HRMS (ESI) calcd. for C11H13N5O5P (M-H): 325.0660, found: 325.0660. 1H NMR (D2O) δ: 8.56 (m, 2H, H-2’), 7.90 (m, 2H, H-3’), 4.49 (m, 2H, H-1’’), 3.93 (m, 2H, H-2’), 3.63 (d, 2H, J(H-C-P)=8.5 Hz, CH2P). 13C NMR (D2O) δ: 171.80 (C-6), 171.19 (C-2), 168.70 (C-4), 149.87 (2C, C-2’), 143.79 (C-4’), 122.98 (2C, C-3’), 70.82 (d, J(2’’,P)=10.5 Hz, C-2’’), 69.14 (d, J(C-P)=151.9 Hz, CH2P), 67.47 (C-1’’). FTIR (KBr, cm-1) ν: 3341, 3137, 3034, 1638, 1578, 1555, 1532, 1401, 1341, 1142, 919. UV (H2O) λmax (ε): pH=2: 269 (5.9), pH=7: 271 (4.7), pH=12: 270 (4.8).
2-(6-Amino-2-oxo-4-phenyl-1,3,5-triazin-1(2H)-yl)ethoxy)methylphosphonic acid ammonium salt (17a)
Yield: 82%. MS (ESI-) m/z (%): 325 (100, M-H). HRMS (ESI) calcd. for C12H14N4O5P (M-H): 325.0707, found: 325.0709. 1H NMR (D2O) δ: 8.01 (m, 2H, H-2’), 7.59 (m, 1H, H-4’), 7.47 (m, 2H, H-3’), 4.04 (t, 2H, J(1’’,2’’)=5.1 Hz, H-1’’), 3.86 (t, 2H, J(2’’,1’’)=5.1 Hz, H-2’’), 3.68 (d, 2H, J(H-C-P)=8.7 Hz, CH2P). 13C NMR (D2O) δ: 172.20 (C-4), 161.14 (C-2 or C-6), 158.67 (C-2 or C-6), 134.98 (C-1’), 133.43 (C-4’), 129.19 (2C, C-2’ or C-3’), 129.13 (2C, C-2’ or C-3’), 70.58 (d, J(2’’,P)=11.6 Hz, C-2’’), 68.18 (d, J(C-P)=155.9 Hz, CH2P), 44.46 (C-1’’). FTIR (KBr, cm-1) ν: 3377, 3130, 3034, 1686, 1662, 1636, 1546, 1481, 1427, 1394, 1151, 1011, 942. UV (H2O) λmax (ε): pH=2: 275 (11.0), pH=7: 258 (18.0), pH=12: 258 (10.5).
2-(6-Amino-2-oxo-4-(pyridin-3-yl)-1,3,5-triazin-1(2H)-yl)ethoxy)methylphosphonic acid ammonium salt (17b)
Yield: 80%. MS (ESI-) m/z (%): 326 (100, M-H). MS (ESI) m/z (%): 328 (81, M+H), 350 (63, M+Na), 372 (100, M+2Na-H), 394 (60, M+3Na-2H). HRMS (ESI) calcd. for C11H15N5O5P (M+H): 328.0805, found: 328.0804.1H NMR (D2O) δ: 8.66 (dm, 1H, J(6’,5’) = 4.8 Hz, H-6’), 8.24 (dm, 1H, J(3’,4’) = 7.9 Hz), 8.03 (td, 1H, J(4’,3’) = J(4’,5’) = 7.8 Hz, J(4’,6’) = 1.8 Hz, H-4’), 7.65 (ddm, 1H, J(5’,4’) = 7.6 Hz, J(5’,6’) = 4.9 Hz, H-5’), 4.22 (t, 2H, J(1’’,2’’) = 5.1 Hz, H-1’’), 3.94 (t, 2H, J(2’’,1’’) = 5.1 Hz, H-2’’), 3.68 (d, 2H, J(H,P) = 8.8 Hz, OCH2P). 13C NMR (D2O) δ: 170.49 (C-4), 161.90 (C-2 or C-6), 158.92 (C-2 or C-6), 151.58 (C-2’), 149.62 (C-6’), 139.27 (C-4’), 127.99 (C-5’), 125.27 (C-3’), 70.64 (d, J(2’’,P) = 12.0 Hz, C-2’’), 68.09 (d, J(C,P) = 156.2 Hz, OCH2P), 44.75 (C-1’).
(2-(6-Amino-2-oxo-4-(pyridin-3-yl)-1,3,5-triazin-1(2H)-yl)ethoxy)methylphosphonic acid ammonium salt (17c)
Yield: 85%. MS (ESI-) m/z (%): 326 (100, M-H), 653 (43, 2M-H). MS (ESI) m/z (%): 328 (57, M+H), 350 (94, M+Na), 372 (100, M+2Na-H), 394 (35, M+3Na-2H). HRMS (ESI) calcd. for C11H15N5O5P (M+H): 328.0805, found: 328.0804; calcd. for C11H14N5O5NaP (M+Na): 350.0625, found: 350.0625.1H NMR (500 MHz, D2O) δ: 9.10 (s, 1H, H-2’), 8.66 (bd, 1H, J(6’,5’) = 5.0 Hz, H-6’), 8.44 (dt, 1H, J(4’,5’) = 8.1 Hz, J(4’,2’) = J(4’,6’) = 1.8 Hz, H-4’), 7.54 (dd, 1H, J(5’,4’) = 8.0 Hz, J(5’,6’) = 5.0 Hz, H-5’), 4.15 (t, 2H, J(1’’,2’’) = 5.1 Hz, H-1’’), 3.91 (t, 2H, J(2’’,1’’) = 5.1 Hz, H-2’’), 3.69 (d, 2H, J(H,P) = 8.8 Hz, CH2-P). 13C NMR (125.7 MHz, D2O) δ: 170.38 (C-4), 161.46 (C-2 or C-6), 158.74 (C-2 or C-6), 152.19 (C-6’), 149.11 (C-2’), 138.09 (C-4’), 131.67 (C-3’), 124.78 (C-5’), 70.65 (d, J(2’’,P) = 11.7 Hz, C-2’’), 68.14 (d, J(CH2,P) = 156.1 Hz, CH2-P), 44.61 (C-1’’). FTIR (KBr, cm-1) ν: 3184, 3007, 2887, 1651, 1595, 1551, 1506, 1472, 1381, 1195, 1165, 1060, 1026, 800 cm-1. UV (H2O) λmax (ε): pH=2: 264 (125), 238 (180); pH=7: 268 (250), 238 (300), pH=12: 268 (280), 237 (380).
(2-(6-Amino-2-oxo-4-(pyridin-4-yl)-1,3,5-triazin-1(2H)-yl)ethoxy)methylphosphonic acid ammonium salt (17d)
Yield: 86%. MS (ESI-) m/z (%): 326 (100, M-H). HRMS (ESI) calcd. for C11H13N5O5P (M-H): 325.0660, found: 325.0660. 1H NMR (D2O) δ: 8.65 (m, 2H, H-2’), 7.80 (m, 2H, H-3’), 4.18 (t, 2H, J(1’’,2’’)=5.2 Hz, H-1’’), 3.89 (t, 2H, J(2’’,1’’)=5.2 Hz, H-2’’), 3.54 (d, 2H, J(H-C-P)=8.3 Hz, CH2P). 13C NMR (125.7 MHz, D2O) δ: 170.67 (C-4), 161.81 (C-2 or C-6), 159.02 (C-2 or C-6), 149.96 (2C, C-2’), 144.12 (C-4’), 123.28 (2C, C-3’), 70.27 (d, J(2’’,P)=10.3 Hz, C-2’’), 70.11 (d, J(C-P)=149.7 Hz, CH2P), 45.03 (C-1’’). FTIR (KBr, cm-1) ν: 3397, 3133, 3033, 1665, 1577, 1540, 1399, 1160, 1061, 1046, 904. UV (H2O) λmax (ε): pH=2: 270 (7.7), pH=7: 275 (7.2), pH=12: 275 (7.0).
Antiviral Activity Assays
The compounds were evaluated against the following viruses: the herpes simplex virus type 1 (HSV-1) the KOS strain, the thymidine kinase-deficient (TK-) HSV-1 derived from the KOS strain resistant to ACV (ACVr), the herpes simplex virus type 2 (HSV-2) the Lyons and G strains, the varicella-zoster virus (VZV) the Oka strain, the TK- VZV the 07-1 strain, the human cytomegalovirus (HCMV) the AD-169 and Davis strains, the feline herpesvirus, the vaccinia virus the Lederle strain, the human immunodeficiency virus (HIV) type 1 (IIIB) and type 2 (ROD), the murine Moloney sarcoma virus (MSV), the respiratory syncytial virus (RSV) the Long strain, the vesicular stomatitis virus (VSV), the Coxsackie B4, the Parainfluenza 3, the Reovirus-1, the Sindbis, the Punta Toro, the feline coronavirus, the influenza A virus the H1N1 and the H3N2 subtypes, and the influenza B virus. The antiviral assays, other than HIV and MSV, were based on the inhibition of virus-induced cytopathicity or plaque formation in human embryonic lung (HEL) fibroblasts, African green monkey cells (Vero), human epithelial cells (HeLa), Crandell-Rees feline kidney cells (CRFK), or Madin Darby canine kidney cells (MDCK) following the previously established procedures.27 The confluent cell cultures in 96-well microtiter plates were inoculated with 100 CCID50 of virus (1 CCID50 being the virus dose to infect 50% of the cell cultures) or with twenty plaque forming units (PFU). After a 1–2 h adsorption period, the residual virus was removed, and the cell cultures were incubated in the presence of varying concentrations of the test compounds. Viral cytopathicity or plaque formation (VZV) was recorded as soon as it reached completion in the control virus-infected cell cultures that were not treated with the test compounds. Antiviral activity was expressed as the EC50 or compound concentration required to reduce virus-induced cytopathogenicity or viral plaque formation by 50%. The methodology of the anti-HIV assays was as follows: human CEM (~3 x 105 cells/ml) were infected with 100 CCID50 of HIV-1(IIIB) or HIV-2(ROD)/ml and seeded in 200-µL wells of a microtiter plate containing appropriate dilutions of the test compounds. After four days of incubation at 37 °C, HIV-induced CEM giant cell formation was examined microscopically. To assess the anti-MSV activity, the confluent C3H/3T3 cell cultures were infected with eighty foci-forming units (FFU) of MSV in 48-well microtiter plates. After six days of cultivation, cell transformation was recorded microscopically.
Cytotoxicity Assays
The cytotoxicity measurements were based on the inhibition of cell growth. The HEL cells were seeded at a rate of 5 x 103 cells/well into 96-well microtiter plates and allowed to proliferate for 24 h. Then, a medium containing different concentrations of the test compounds was added. After three days of incubation at 37 °C, the cell number was determined with a Coulter counter. The cytostatic concentration was calculated as the CC50, or the compound concentration required to reduce cell proliferation by 50% relative to the number of cells in the untreated controls. The CC50 values were estimated from graphic plots of the number of cells (percentage of control) as a function of the concentration of the test compounds. Alternatively, the cytotoxicity of the test compounds was expressed as the minimum cytotoxic concentration (MCC) or the compound concentration that caused a microscopically detectable alteration of cell morphology.
ACKNOWLEDGEMENTS
This work is a part of the research project of the Institute Z40550506. It was supported by the Centre for New Antivirals and Antineoplastics 1M0508 funded by the Ministry of Education, Youth and Sports of the Czech Republic, by Gilead Sciences, Inc. (Foster City, U.S.A.), and the “Geconcerteerde Onderzoeksacties” (GOA), Krediet nr. 10/014” of the K.U.Leuven. The authors thank Dr. Helena Mertliková-Kaiserova and her group from this Institute for the antitumor assays of final compounds, Dr. Pavel Fiedler for the IR spectra, the staff of the mass spectrometry (Dr. Josef Cvacka, Head) and the analytical departments (Dr. Stanislava Matejkova, Head) of the Institute. The excellent technical assistance of Mr. David Marak is gratefully acknowledged. We also thank Anita Camps, Steven Carmans, Frieda De Meyer, Leentje Persoons, Leen Ingels, Lizette van Berckelaer, Wim Van Dam and Lies Van den Heurck for their excellent technical assistance with the antiviral assays.
References
1. C. B. Yoo and P. A. Jones, Nature Reviews Drug Discovery, 2006, 5, 37. CrossRef
2. P. W. Hollenbach, A. N. Nguyen, H. Brady, M. Williams, Y. H. Ning, N. Richard, L. Krushel, S. L. Aukerman, C. Heise, and K. J. MacBeth, PLOS ONE – 2010, 5, Article No. e9001. CrossRef
3. F. Sorm and A. Piskala, Czech Appl. 139 542, Neth. Appl. 6 414 959 (Chem. Abstr., 1966, 64, 2154).
4. J. Pliml and F. Sorm, Coll. Czech. Chem. Commun., 1964, 29, 2576.
5. A. Piskala and F. Sorm, In Nucleic Acids Chemistry; ed. by L. B. Townsend and R. S. Tipson; Wiley: New York , 1978; Part 1, pp. 435–441.
6. M. Fojtova, A. Piskala, I. Votruba, M. Otmar, E. Bartova, and A. Kovarik, Pharmacol. Res., 2007, 1, 16. CrossRef
7. J. Vesely and A. Piskala, Cancer Res., 1984, 44, 5765.
8. A. Holy, M. Otmar, A. Piskala, I. Votruba, A. Kovarik, M. Fojtova, and E. Bartova, WO 2007065231 A2 2006000130.
9. A. Holy, M. Otmar, and A. Piskala, WO 2008101448 A2 20080828.
10. A. Piskala and F. Sorm, Coll. Czech. Chem. Commun., 1964, 29, 2060.
11. J. A. Beisler, M. M. Abbasi, and J. S. Driscoll, J. Med. Chem., 1979, 22, 1230. CrossRef
12. J. A. Beisler, M. M. Abbasi, J. A. Kelley, and J. S. Driscoll, J. Med. Chem., 1977, 20, 806. CrossRef
13. N. J. Vogelzang, J. E. Herndon, C. Cirrincione, D. C. Harmon, K. H. Antman, J. M. Corson, Y. Suzuki, M. L. Citron, and M. R. Green, Cancer, 1997, 79, 2237. CrossRef
14. A. Piskala, B. Cesneková, and J. Vesely, Nucleic Acids Research, Symposium Ser., 1987, 18, 57.
15. K. S. Harris, W. Brabant, S. Styrchak, A. Gall, and R. Daifuku, Antiviral. Res., 2005, 67, 1. CrossRef
16. E. Murakami, A. Basavapathruni, W. D. Bradley, and K. S. Anderson, Antiviral. Res., 2005, 67, 10. CrossRef
17. J. D. Graci and C. E. Cameron, Future Virol., 2008, 3, 553. CrossRef
18. M. Krecmerova, A. Holy, A. Piskala, M. Masojidkova, J. Balzarini, G. Andrei, R. Snoeck, L. Naesens, J. Neyts, and E. De Clercq, J. Med. Chem., 2007, 50, 1069. CrossRef
19. M. Krecmerova, A. Holy, R. Pohl, M. Masojidkova, G. Andrei, L. Naesens, J. Neyts, J. Balzarini, and E. De Clercq, J. Med. Chem., 2007, 50, 5765. CrossRef
20. A. Holy, M. Krecmerova, A. Piskala, G. Andrei, R. Snoeck, E. De Clercq, J. Neyts, and L. Naesens, WO 2008083634 A1 20080717.
21. M. Dracinsky, M. Krecmerova, and A. Holy, Bioorg. Med. Chem., 2008, 16, 6778. CrossRef
22. M. Krecmerova, M. Masojidkova, and A. Holy, Bioorg. Med. Chem., 2010, 18, 387. CrossRef
23. N. B. Hanna, M. Masojidkova, P. Fiedler, and A. Piskala, Coll. Czech. Chem. Commun., 1997, 63, 222. CrossRef
24. A. Diaz-Ortiz, J. Elguero, C. Foces-Foces, A. de la Hoz, A. Moreno, M. del Carmen Mateo, A. Sanchez-Migallon, and G. Valiente, New J. Chem., 2004, 28, 952. CrossRef
25. J. K. Simons and M. R. Saxton, Organic Syntheses, Coll. Vol. 4, p.78 (1963); Vol. 33, p.13 (1953).
26. A. Holy, I. Votruba, M. Masojidkova, G. Andrei, R. Snoeck, L. Naesens, E. De Clercq, and J. Balzarini, J. Med. Chem., 2002, 45, 1918. CrossRef
27. A. Holy, H. Dvorakova, J. Jindrich, M. Masojidková, M. Budesinsky, J. Balzarini, G. Andrei, and E. De Clercq, J. Med. Chem., 1996, 39, 4073. CrossRef