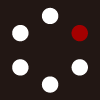
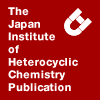
HETEROCYCLES
An International Journal for Reviews and Communications in Heterocyclic ChemistryWeb Edition ISSN: 1881-0942
Published online by The Japan Institute of Heterocyclic Chemistry
e-Journal
Full Text HTML
Received, 25th January, 2011, Accepted, 21st February, 2011, Published online, 28th February, 2011.
DOI: 10.3987/COM-11-12154
■ Preparation and Reaction of Quinolinyl (or Pyridinyl)phosphonium Salts with Base and Pivalaldehyde
Minami Shimada, Osamu Sugimoto,* Akihiro Sato, and Ken-ichi Tanji
Laboratory of Organic Chemistry, School of Food and Nutritional Sciences, University of Shizuoka, 52-1 Yada, Shizuoka 422-8526, Japan
Abstract
α- and γ-Heteroaryltriphenylphosphonium iodides were prepared by reaction of the corresponding heteroaryl iodides with triphenylphosphine. Reaction of β-heteroaryl iodides with triphenylphosphine in the presence of a palladium catalyst gave β-heteroaryltriphenylphosphonium iodides. Elimination of the heteroaryl group was achieved by treating the heteroaryltriphenylphosphonium iodides with a base. Further, the heteroaryl group was trapped with pivalaldehyde to introduce a pivaloyl substituent onto the heteroaromatic ring.INTRODUCTION
There are many reactions that take advantage of the high affinity between phosphorus and oxygen. Alkenes are prepared via reaction of carbonyl compounds with phosphonium ylides (Wittig reaction)1 or phosphonate carbanions (Horner-Wadsworth-Emmons reaction).2 Carboxylic acids and sulfonic acids can be converted into the corresponding acyl chlorides and sulfonyl chlorides using phosphorus oxychloride,3,4 phosphorus pentachloride,5 triphenylphosphine-carbon tetrachloride6 or triphenylphosphine-N-chlorosuccinimide.7 Phosphorus oxyhalides, triphenylphosphine-N-halosuccinimides and triphenylphosphine-trichloroisocyanuric acid are used for halogenation of hydroxyheteroaromatics.8,9 We have reported in a preliminary communication10 that heteroaryltriphenylphosphonium iodides prepared from triphenylphosphine and heteroaryl iodides react with protonic solvents such as water and ethanol in the presence of base to eliminate the heteroaryl group (A). We have now applied this reaction to carbon-carbon bond formation (B) and report our results in this paper (Scheme 1).
RESULTS AND DISCUSSION
Initially, quinolinyltriphenylphosphonium iodides (1-3) were prepared by two routes, Method A and Method B, as shown in Scheme 2.
Method A is a simple addition-elimination reaction between triphenylphosphine and organic halides, but requires the halide to be reactive towards nucleophiles, while Method B is a palladium catalyzed cross coupling reaction11 between triphenylphosphine and organic halides that does not require reactivity towards nucleophiles. Method A12 can be applied to the reaction of 2-iodo- and 4-iodoquinoline derivatives since it is known that the positions α or γ to the ring nitrogen in nitrogen-containing heteroaromatics are reactive with nucleophiles.13 For example, reaction of 7-chloro-4-iodoquinoline with triphenylphosphine at 110 °C gave the disired phosphonium iodide 1 in 85% yield. Similarly, reaction of 2-iodoquinoline with triphenylphosphine at 110 °C afforded 3 in 91% yield. In contrast, reaction of 3-iodoquinoline with triphenylphosphine does not proceed under these conditions because the position β to the ring nitrogen in nitrogen-containing heteroaromatics is inert to nucleophiles. However, 3-iodoquinoline can be converted to the phosphonium salt using Method B. Reaction of 3-iodoquinoline with triphenylphosphine in xylene in the presence of a catalytic amount of tris(dibenzylideneacetone)dipalladium gave the phosphonium salt, 2 in 95% yield.
Method A can also be used to prepare pyridinyl derivatives since the α-position of the pyridine ring is reactive with nucleophiles.13 Preparation of 2-pyridinyltriphenylphosphonium iodides 4 and 5 was carried out by reaction of triphenylphosphine with 2-iodopyridine and 2,5-diiodopyridine at 140 °C in 66% and 79% yields, respectively (Scheme 3).
Next, preparation of 2-pyridinyltriphenylphosphonium trifluoromethanesulfonate (6) by using 2-(trifluoromethanesulfonyloxy)pyridine as a substrate instead of 2-iodopyridine was examined (Table 1). First, reaction of the substrate with triphenylphosphine by Method A was attempted because the trifluoromethanesulfonyloxy group is regarded as a good leaving group.14,15 The product 6 was not obtained, however, even when the reaction was run at 120-130 °C for 0.5 h (Entry 1). Preparation of 6 using the palladium catalyzed coupling reaction was then carried out. A mixture of the substrate, triphenylphosphine, and tetrakis(triphenylphosphine)palladium was heated at 130 °C for 0.5 h to gratifyingly afford 6 in 87% yield (Entry 2).
The reactivity of the quinolinyltriphenylphosphonium iodides (1-3) was then evaluated by treating them with several reagents (Table 2). Reaction of 1 with water or ethanol was unsuccessful (Entries 1, 2). On the other hand, reaction of 1 with basic solvents such as aqueous sodium hydroxide (Entry 3) and ethanol-triethylamine (Entry 4) gave the desired quinoline in 82% and 87% yields, respectively. Moreover, the quinoline was obtained in good yield even though an aprotic solvent, N,N-dimethylformamide containing triethylamine was used (Entry 5). This result suggests that a quinolinyl anion abstracts a proton even from an aprotic compound because of its instability at high temperature. Generation of quinolines by reaction of 2 and 3 with ethanol-triethylamine (Entries 6, 7) proceeded in a manner similar to that in Entry 4.
Functionalization of the quinoline substrates was next examined. Reaction of 3 with base in the presence of pivalaldehyde was carried out to introduce the pivaloyl substituent at the α-position of the quinoline ring (Table 3). This reaction was conducted at room temperature because the generated quinolinyl anion would be unstable at higher temperatures. To avoid generation of quinoline, either no or an aprotic solvent was used. The combination of triethylamine in tetrahydrofuran afforded the product, 2,2-dimethyl-1-(2-quinolinyl)-1-propanol in 39% yield along with 23% of revovered quinoline (Entry 1). When strong bases such as sodium hydroxide (Entry 2), sodium oxide (Entries 3-5), and potassium hydroxide (Entries 6-8) were used, product yields were slightly improved, but recovered quinoline was still isolated as a byproduct. Interestingly, elimination of the quinolinyl group occurs selectively to react with pivalaldehyde or a proton source, but elimination of the phenyl group followed by trapping with pivalaldehyde does not occur.
Treatment of 2 with base in the presence of pivalaldehyde was then carried out to compare its reactivity with that of 3 (Table 4).
A mixture of 2, potassium hydroxide, and pivalaldehyde in a solvent (benzene, THF, and no solvent) was stirred at room temperature to give 2,2-dimethyl-1-(3-quinolinyl)-1-propanol in 29%, 25%, and 41% yields with quinoline in 67%, 56%, and 36% yields, respectively (Entries 1-3). As compared with the results in Table 3, the yield of the desired product was lower and that of quinoline higher. In addition, the use of sodium oxide and potassium t-butoxide as base afforded the product in poor yields (Entries 4, 5).
Next, the reaction of 1 with base in the presence of pivalaldehyde at room temperature was explored (Table 5). In this case, elimination of the 7-chloroquinolinyl group proceeded smoothly to give high total yields of the desired product and 7-chloroquinoline (Entries 2-4, 62-90%), but the eliminated 7-chloroquinolinyl group was preferentially trapped by a proton rather than pivalaldehyde.
Figure 1 shows the speculated stabilities of quinoline carbanions generated from 2-, 3-, and 4-quinolinyltriphenylphosphonium salts and bases. It is reasonable that a carbanion at the α-position of quinoline (α-carbanion) is more stable than the β-carbanion, and the β-carbanion is more stable than the γ-carbanion since the ring nitrogen has a strong inductive effect (“I effect”) for stabilization of nearby carbanions. Unstable carbanions such as the γ-carbanion immediately trap a proton from other reagents.
From the results shown above, it is estimated that carbanions at the α-position of pyridines, by reaction of 2-pyridinyltriphenylphosphonium salts with base, will trap pivalaldehyde to form 2,2-dimethyl-1-(2-pyridinyl)-1-propanols. As shown in Table 6, 2-pyridinyltriphenylphosphonium iodide (4), (5-iodo-2-pyridinyl)triphenylphosphonium iodide (5) and 2-pyridinyltriphenylphosphonium trifluoromethanesulfonate (6) were used as substrates to test this hypothesis. As expected, carbon-carbon bond formation proceeded to give the products in moderate to good yields. It was found that both triflates and iodides are effective in this transformation.
In summary, we have demonstrated that heteroaryltriphenylphosphonium salts react in the presence of base to eliminate the heteroaryl group, which can then be trapped with an aldehyde or proton. Furthermore, the reactivity of the eliminated heteroaryl group with pivalaldehyde varies depending on the position of the carbanion in the heteroaromatic ring.
EXPERIMENTAL
All melting points were not corrected. 1H-NMR spectra were measured with an Hitachi R-90H spectrometer (90 MHz) using tetramethylsilane as an internal standard.
Preparation of 7-chloro-4-quinolinyltriphenylphosphonium iodide (1) (Scheme 2): A mixture of 7-chloro-4-iodoquinoline (5790 mg, 20.0 mmol) and triphenylphosphine (5246 mg, 20.0 mmol) was dissolved in CH2Cl2 (50 mL). The CH2Cl2 was then removed under atmospheric pressure and the residue heated at 110 °C for 1 h followed by recrystallization from EtOH to give 1 (9362 mg, 85%).
1: Yellow prisms. Mp 257-265 °C. 1H-NMR (DMSO-d6) ppm: 7.28-8.13 (18H, m, C3,C5,C6, and triphenyl-H), 8.42 (1H, brs, C8-H), 9.28 (1H, t, J=4.3 Hz, C2-H). Anal. Calcd for C27H20ClINP: C, 58.77; H, 3.65; N, 2.54. Found: C, 58.50; H, 3.86; N, 2.33.
Preparation of 3-quinolinyltriphenylphosphonium iodide (2) (Scheme 2): A mixture of 3-iodoquinoline (3840 mg, 15.1 mmol), tris(dibenzylideneacetone)dipalladium (140 mg, 0.153 mmol), triphenylphosphine (3944 mg, 15.0 mmol) and xylene (10 mL) was heated to reflux for 16 h. The reaction mixture was then washed with AcOEt and the residual solids dried under reduced pressure to give 2 (7400 mg, 95%).
2: Slightly yellow solids (recryst. from AcOEt-EtOH). Mp 234-235 °C. 1H-NMR (CDCl3) ppm: 7.56-8.12 (17H, m, C6,C7, and triphenyl-H), 8.21 (1H, d, J=8.1 Hz, C5-H), 8.43 (1H, d, J=7.7 Hz, C8-H), 8.87 (1H, dd, J=4.5 Hz, 2.3 Hz, C4-H), 9.09 (1H, dd, J=14.7 Hz, 2.1 Hz, C2-H). Anal. Calcd for C27H21INP: C, 62.68; H, 4.09; N, 2.71. Found: C, 62.52; H, 4.21; N, 2.76.
Preparation of 2-quinolinyltriphenylphosphonium iodide (3) (Scheme 2): A mixture of 2-iodoquinoline (3460 mg, 13.6 mmol) and triphenylphosphine (3559 mg, 13.6 mmol) was heated at 110 °C for 1 h 40 min. The reaction mixture was then washed with AcOEt and the residual powder dried under reduced pressure to give 3 (6377 mg, 91%).
3: Milky white powder. Mp 213-214 °C. 1H-NMR (CDCl3) ppm: 7.57-8.30 (20H, m, C3,C5,C6,C7,C8, and triphenyl-H), 8.85 (1H, dd, J=8.6 Hz, 5.5 Hz, C4-H). Anal. Calcd for C27H21INP·0.5H2O: C, 61.61; H, 4.21; N, 2.66. Found: C, 61.60; H, 4.63; N, 2.51.
Preparation of 2-pyridinyltriphenylphosphonium iodide (4) (Scheme 3): A mixture of 2-iodopyridine (4100 mg, 20.0 mmol) and triphenylphosphine (5246 mg, 20.0 mmol) was heated at 140 °C for 3 h. The reaction mixture was cooled and washed with AcOEt (30 mL) to give 4 (6129 mg, 66%).
4: Slight yellow powder. Mp 287.6 °C (lit.,16 289-292 °C). 1H-NMR (DMSO-d6) ppm: 7.46-8.40 (18H, m, C3,C4,C5, and phenyl-H), 9.07 (1H, d, J=4.4 Hz, C6-H).
Preparation of (5-iodo-2-pyridinyl)triphenylphosphonium iodide (5) (Scheme 3): A mixture of 2,5-diiodopyridine (6628 mg, 20.0 mmol) and triphenylphosphine (5258 mg, 20.0 mmol) was heated at 140 °C for 5 h. The reaction mixture was cooled and washed with AcOEt (20 mL) and the residue recrystallized from EtOH to give 5 (9319 mg, 79%).
5: Yellow prisms. Mp 243.4 °C. 1H-NMR (DMSO-d6) ppm: 7.49-8.19 (16H, m, C3 and phenyl-H), 8.48-8.75 (1H, m, C4-H), 9.32 (1H, s, C6-H). Anal. Calcd for C23H18I2NP: C, 46.57; H, 3.06; N, 2.36. Found: C, 46.45; H, 3.07; N, 2.52.
Preparation of 2-pyridinyltriphenylphosphonium trifluoromethanesulfonate (6) (Table 1): A mixture of 2-trifluoromethanesulfonyloxypyridine (3177 mg, 14.0 mmol), triphenylphosphine (3668 mg, 14.0 mmol) and tetrakis(triphenylphosphine)palladium (51 mg, 0.0441 mmol) in CH2Cl2 (10 mL) was stirred and CH2Cl2 was then removed. The residual mixture was heated at 130 °C for 30 min. The reaction mixture was then cooled and washed with Et2O (20 mL). The crude solids were purified by silica gel column chromatography (eluted with hexane-AcOEt (5:1)) to give 6 (5985 mg, 87%).
6: White powder. Mp 251.8-253.4 °C (lit.,16 256-259 °C). 1H-NMR (CDCl3) ppm: 7.48-8.03 (17H, m, C4,C5, and phenyl-H), 8.14 (1H, m, C3-H), 8.99 (1H, d, J=4.9 Hz, C6-H).
Reaction of 1 with 0.5 N NaOH solution (Table 2, Entry 3): A mixture of 1 (552 mg, 1.00 mmol) in 0.5 N aqueous NaOH (20 mL) was heated to reflux for 1 h. The reaction mixture was then neutralized and extracted with AcOEt. The organic layer was concentrated and purified by silica gel column chromatography (eluted with hexane-AcOEt (3:1)) to give 7-chloroquinoline (135 mg, 82%).
7-Chloroquinoline: Colorless liquids. MS 163 (M+). 1H-NMR (CDCl3) ppm: 7.28-7.62 (2H, m, C3 and C6-H), 7.76 (1H, d, J=8.7 Hz, C5-H), 7.96-8.28 (2H, m, C4 and C8-H), 8.91 (1H, dd, J=4.1 Hz, 1.4 Hz, C2-H).
Reaction of 1 with EtOH and triethylamine (Table 2, Entry 4): A mixture of 1 (552 mg, 1.00 mmol), EtOH (10 mL) and triethylamine (3 mL) was heated to reflux for 1 h. After EtOH and triethylamine were removed under reduced pressure, the residue was purified by silica gel column chromatography (eluted with hexane-AcOEt (3:1)) to give 7-chloroquinoline (143 mg, 87%).
Reaction of 1 with N,N-dimethylformamide and triethylamine (Table 2, Entry 5): A mixture of 1 (552 mg, 1.00 mmol), N,N-dimethylformamide (2 mL) and triethylamine (2 mL) was heated at 120 °C for 1.5 h. The reaction mixture was then purified by silica gel column chromatography (eluted with hexane-AcOEt (2:1)) to give 7-chloroquinoline (109 mg, 67%).
Reaction of 2 with EtOH and triethylamine (Table 2, Entry 6): A mixture of 2 (517 mg, 1.00 mmol), EtOH (10 mL) and triethylamine (3 mL) was heated to reflux for 1 h. After EtOH and triethylamine were removed under reduced pressure, the residue was purified by silica gel column chromatography (eluted with hexane-AcOEt (1:1)) to give quinoline (112 mg, 87%).
Quinoline: Colorless liquids. 1H-NMR (CDCl3) ppm: 7.39 (1H, dd, J=8.3 Hz, 4.2 Hz, C3-H), 7.48-7.93 (3H, m, C5, C6, and C7-H), 7.96-8.31 (2H, m, C4 and C8-H), 8.92 (1H, dd, J=4.2 Hz, 1.5 Hz, C2-H).
Reaction of 3 with EtOH and triethylamine (Table 2, Entry 7): A mixture of 3 (523 mg, 1.01 mmol), EtOH (10 mL) and triethylamine (3 mL) was heated to reflux for 1 h. After EtOH and triethylamine were removed under reduced pressure, the residue was purified by silica gel column chromatography (eluted with hexane-AcOEt (1:1)) to give quinoline (95 mg, 73%).
Reaction of heteroaryltriphenylphosphonium salts with base in the presence of pivalaldehyde (General procedure for Table 3-6): A mixture of heteroaryltriphenylphosphonium salts, pivalaldehyde, base, and solvent (if applicable) was stirred at room temperature. Water was then added to the reaction mixture and the suspension neutralized with 1 N HCl solution. The suspension was extracted with AcOEt. After the organic layer was concentrated, the residue was purified by silica gel column chromatography to give the pure compounds.
2,2-Dimethyl-1-(2-quinolinyl)-1-propanol: White solids. Mp 77.7 °C (lit.,17 73-75 °C). 1H-NMR (CDCl3) ppm: 0.98 (9H, s, tBu), 4.52 (1H, d, J=5.8 Hz, CHOH), 4.78 (1H, d, J=5.8 Hz, CHOH), 7.32 (1H, d, J=8.4 Hz, C3-H), 7.40-7.98 (3H, m, C5, C6, and C7-H), 8.08 (2H, d, J=8.4 Hz, C4 and C8-H).
2,2-Dimethyl-1-(3-quinolinyl)-1-propanol: Pale yellow needles. Mp 177.4 °C. 1H-NMR (CDCl3) ppm: 0.98 (9H, s, tBu), 2.29 (1H, brs, CHOH), 4.61 (1H, s, CHOH), 7.38-7.91 (3H, m, C5, C6, and C7-H), 8.06 (2H, brs, C4 and C8-H), 8.83 (1H, d, J=1.9 Hz, C2-H). Anal. Calcd for C14H17NO: C, 78.10; H, 7.96; N, 6.51. Found: C, 78.06; H, 8.04; N, 6.66.
2,2-Dimethyl-1-(7-chloro-4-quinolinyl)-1-propanol: Yellow solids. Mp 160.2 °C. 1H-NMR (CDCl3) ppm: 0.97 (9H, s, tBu), 2.54 (1H, brs, CHOH), 5.26 (1H, s, CHOH), 7.30-7.65 (2H, m, C3 and C6-H), 7.92-8.16 (2H, m, C4 and C8-H), 8.83 (1H, d, J=4.6 Hz, C2-H). Anal. Calcd for C14H16ClNO: C, 67.33; H, 6.46; N, 5.61. Found: C, 67.43; H, 6.53; N, 5.76.
2,2-Dimethyl-1-(2-pyridinyl)-1-propanol: Yellow solids. Mp 54.1-55.2 °C (lit.,18 53-57 °C). 1H-NMR (CDCl3) ppm: 0.92 (9H, s, tBu), 4.06-4.52 (2H, br, CHOH), 7.08-7.31 (2H, m, C3 and C5-H), 7.63 (1H, ddd, J=7.6 Hz, 7.6 Hz, 1.8 Hz, C4-H), 8.55 (1H, dd, J=4.7 Hz, 1.8 Hz, C6-H).
2,2-Dimethyl-1-(5-iodo-2-pyridinyl)-1-propanol: Colorless prisms. Mp 77.5-78.5 °C. 1H-NMR (CDCl3) ppm: 0.91 (9H, s, tBu), 3.81 (1H, d, J=7.3 Hz, CHOH), 4.31 (1H, d, J=7.3 Hz, CHOH), 7.02 (1H, d, J=8.2 Hz, C3-H), 7.93 (1H, dd, J=8.2 Hz, 1.9 Hz, C4-H), 8.76 (1H, d, J=1.9 Hz, C6-H).
3-Iodopyridine: Yellow solids. Mp 53.8 °C (lit.,19 52.3-53.0 °C). 1H-NMR (CDCl3) ppm: 7.09 (1H, dd, J=8.1 Hz, 4.6 Hz, C3-H), 8.00 (1H, ddd, J=8.1 Hz, 1.5 Hz, 1.1 Hz, C4-H), 8.55 (1H, dd, J=4.6 Hz, 1.1 Hz, C2-H), 8.84 (1H, d, J=1.5 Hz, C6-H).
References
1. G. Wittig and U. Schollkopf, Chem. Ber., 1954, 87, 1318. CrossRef
2. L. Horner, H. Hoffmann, and H. G. Wippel, Chem. Ber., 1958, 91, 61. CrossRef
3. W. N. Haworth, H. Gregory, and L. F. Wiggins, J. Chem. Soc., 1946, 488. CrossRef
4. R. L. Hinman and L. Locatell, Jr., J. Am. Chem. Soc., 1959, 81, 5655. CrossRef
5. M. Frankel, Y. Liwschitz, and A. Zilkha, J. Am. Chem. Soc., 1954, 76, 2814. CrossRef
6. C. R. Harrison, P. Hodge, B. J. Hunt, E. Khoshdel, and G. Richardson, J. Org. Chem., 1983, 48, 3721. CrossRef
7. J. D. Brown, M. A. Foley, and D. L. Comins, J. Am. Chem. Soc., 1988, 110, 7445. CrossRef
8. O. Sugimoto, M. Mori, K. Moriya, and K. Tanji, Helv. Chim. Acta, 2001, 84, 1112. CrossRef
9. O. Sugimoto and K. Tanji, Heterocycles, 2005, 65, 181. CrossRef
10. A. Sato, O. Sugimoto, and K. Tanji, Heterocycles, 2009, 78, 2735. CrossRef
11. D. Marcoux and A. B. Charette, J. Org. Chem., 2008, 73, 590. CrossRef
12. I. N. Zhmurova, I. M. Kosinskaya, and A. M. Pinchuk, Zh. Obshch. Khim., 1981, 51, 1538.
13. For a review on reaction of heteroaromatics with nucleophiles, see: R. G. Shepherd and J. L. Fedrick, Adv. Heterocycl. Chem., 1965, 4, 145. CrossRef
14. S. Cacchi, A. Carangio, G. Fabrizi, L. Moro, and P. Pace, Synlett, 1997, 1400. CrossRef
15. J. S. Owen, J. A. Labinger, and J. E. Bercaw, J. Am. Chem. Soc., 2004, 126, 8247. CrossRef
16. M. Meciarova, S. Toma, A. Loupy, and B. Horvath, Phosphorus, Sulfur, Silicon, Rel. Elem., 2008, 183, 21.
17. O. Sugimoto, M. Sudo, and K. Tanji, Tetrahedron, 2001, 57, 2133. CrossRef
18. E. Fukuda, Y. Takahashi, N. Hirasawa, O. Sugimoto, and K. Tanji, Heterocycles, 2009, 77, 1163. CrossRef
19. C. B. Herbert and H. M. Darl, J. Am. Chem. Soc., 1955, 77, 3752. CrossRef