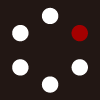
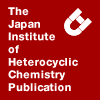
HETEROCYCLES
An International Journal for Reviews and Communications in Heterocyclic ChemistryWeb Edition ISSN: 1881-0942
Published online by The Japan Institute of Heterocyclic Chemistry
e-Journal
Full Text HTML
Received, 20th March, 2011, Accepted, 21st April, 2011, Published online, 9th May, 2011.
DOI: 10.3987/COM-11-S(P)6
■ Synthesis and Spectroscopic Properties of 1,6-Methano[10]annulene-Fused and 2,4-Dimethyl-7,12-methano-3H-cyclohepta[10]annulen-3-one-Fused 10H-Pyrrolo[1,2-a]perimidin-10-ones
Shigeyasu Kuroda,* Naoko Matsumoto, Yanmei Zhang, Takako Abe, Yoshikazu Horino, Yurie Fujiwara, and Mitsunori Oda*
Department of Environmental Applied Chemistry, Faculty of Engineering, Toyama University, Gofuku 3190, Toyama 930-8555, Japan
Abstract
The title 1,6-methano[10]annulene-fused and 2,4-dimethyl-7,12-methano-3H-cyclohepta[10]annulen-3-one-fused 10H-pyrrolo[1,2-a]perimidin-10-ones, denoted by 2 and 3, respectively, were synthesized. A novel and efficient synthetic method involving tandem [4 + 3] cycloaddition and 10π-electrocyclization was developed for constructing the 2,4-dimethyl-7,12-methano- 3H-cyclohepta[10]annulen-3-one skeleton toward the synthesis of 3 from 6H-cyclohepta[c]furan-5,7-dicarbaldehyde (5). Spectroscopic properties of 2 and 3 were also described in relation to the tropone-fusion.Recently, Zuo et al. and we reported the synthesis of 1,6-methano[10]annulene-3,4-dicarboximides (1).1,2 We also disclosed that most imides having the general structure 1 exhibited the emission of green light in a narrow range of 467–472 nm upon excitation and that their emission quantum yields depended on the substituent on the nitrogen atom.2 In order to develop materials having a 1,6-methano[10]annulene-3,4-
dicarboximide skeleton with emission of longer wavelength light, we have been interested in π-extended derivatives of 1, namely 1,6-methano[10]-annulene-fused and 2,4-dimethyl-7,12-methano-3H- cyclohepta[10]annulen-3-one-fused 10H-pyrrolo[1,2-a]perimidin-10-ones, 2 and 3.3 In this communication, we describe the synthesis and spectroscopic properties of 2 and 3. Furthermore, this study highlights the development of a novel synthetic method for constructing the 2,4-dimethyl-7,12- methano-3H-cyclohepta[10]annulen-3-one-fused 1,6-methano[10]annulene skeleton toward the synthesis of 3.
The synthesis of 2 was achieved by condensation of 1,6- methano[10]annulene-3,4-dicaboxylic anhydride (4)1,2 with 1,6- diaminonaphthalene as shown in Scheme 1. Compound 2 was obtained as red microcrystals in 68% yield.4 On the other hand, the synthesis of 3 was accomplished in six steps starting from 6H-cyclohepta[c]furan-5,7-dicarbaldehyde (5)5 as shown in
Scheme 2. Consecutive Horner–Wadsworth–Emmons olefinations of 5 gave 6 in an overall yield of 86%. The treatment of 6 with 2,4-dibromo-3-pentanone in the presence of KI/Cu in refluxing acetonitrile gave 86 in 55% yield. This conversion involved a [4 + 3] cycloaddition7 at the furan ring of 6, forming the tetrahydroheptalenone skeleton of intermediate 7, followed by a thermal 10π-electrocyclization and subsequent dehydrobromination of 7, leading to the formation of the methano[10]annulene core. Treatment of 8 with fluorosulfonic acid facilitated not only the dehydration of the ether linkage in the seven-membered ring of 8 but also concomitant formation of an anhydride group to give 9 in 87% yield. Finally, condensation of 9 with 1,6-diaminonaphthalene in refluxing DMF afforded 3 as deep red microcrystals in 95% yield. It should be noted that the series of reactions in Scheme 2 represents a novel and efficient method for constructing a tropone-fused 1,6-methano[10]annulene framework. Although we had already reported the synthesis of 3,4-tropono-1,6-methano[10]annulene,8 the method developed in this study provides an efficient alternative route to this ring system. Since a furan ring can be transformed into various functional groups, such as dialdehydes, lactones, and acetals, the utility of this method could be extended to the synthesis of 3,4,8,9-functionalized 1,6-methano[10]annulenes.
The UV–vis absorption and emission spectra of 2 and 3 are shown in Figures 1 and 2, respectively. The UV-vis absorption spectra in CH2Cl2 show main absorption maxima at 291 and 486 nm for 2 and at 334 and 494 nm for 3. The shorter wavelength bands of 2 and 3 exhibit relatively large intensities and a large difference of 42 nm, while the longer wavelength bands exhibit small intensities and a modest difference of only 8 nm, suggesting that the latter bands are derived from excitation of the common chromophore, the pyrrolo[1,2-a]perimidin-10-one fragment, in 2 and 3. In other words, the fused tropone does not have a significant effect on the shift of the long wavelength absorption. Compound 2 exhibits emission at 456
and 561 nm upon excitations at 291 and 486 nm, respectively. Interestingly, these two emission peaks are observed upon excitations at 322 nm at a time (vide infra). Following excitations at 334 and 494 nm, compound 3 exhibits emission at 501 and 561 nm, respectively. The emission curve resulting from excitation at 334 nm additionally includes the 561 nm peak at its foot slope. These emission behaviors of 2 and 3 are in contrast to those of 1, which emit light only from the lowest excited state. Emission quantum yields upon excitation at 356 nm were determined to be 4.8 x 10–3 for 2 and 1.1 x 10–4 for 3, which are far smaller than those of 1.9 In acidic media, UV-vis absorptions of 2 and 3 vary. In a solution of 1% CF3CO2H in CH2Cl2, 2 and 3 show three and two distinct absorption bands in the range 250–400 nm, respectively, and their long wavelength absorptions appear as broad bands (Figures 3 and 4).
Emissions of 2 and 3 in this solvent system also vary. Compound 2 exhibits emission at 480 nm upon excitation at 322 nm and 3 emits at 496 nm upon excitation at 372 nm. It is noteworthy that the emission of 2 changes dramatically depending on the concentration of CF3CO2H in CH2Cl2,10 while the emission of 3 does not (Figures 5 and 6). This suggests that compound 2 can be used for sensing an acid in an organic solvent by monitoring its emission spectrum, though it is not yet clear why such a drastic quenching of the emission of 2 occurs by simply adjusting the pH. Further structural modifications to 2 and analyses of the spectroscopic properties of the resulting analogs are currently in progress.
In conclusion, we have synthesized the title 10H-pyrrolo[1,2-a]perimidin-10-one derivatives, 2 and 3. For the synthesis of 3, we have developed a novel and efficient synthetic method involving tandem [4 + 3] cycloaddition and 10π-elctrocyclization reactions for construction of the tropono-1,6- methano[10]annulene skeleton from 6H-cyclohepta[c]furan-5,7-dicarbaldehyde (5). The absorption and emission properties of 2 and 3 were also studied to evaluate the effects of the fused tropone. Incorporation of this fused ring onto 2 brought about restraint in quenching of the emission by protonation, and also resulted in a reduction in quantum yield.
ACKNOWLEDGEMENTS
We thank Ms Naoko Tamura at the University of Toyama for her preliminary research assistance in the preparation of compound 3.
References
1. F. Jin, J.-J. Liu, H.-P. Chen, and S. Zuo, Hecheng Huaxue, 2009, 17, 606; F. Jin, J. Liu, H.-P. Chen, and S. Zuo, Beijing Huagong Daxue Xuebao, Ziran Kexueban, 2010, 37, 28.
2. S. Kuroda, N. Tamura, R. Miyatake, N. Matsumoto, Y. Horino, D. Miyawaki, and M. Oda, Heterocycles, 2011, 83, 789. CrossRef
3. For most recent photophysical properties of the pyrroperimidinone derivatives, see; S. Goswami, D. Sen, N. K. Das, and G. Hazra, Tetrahedron Lett., 2010, 51, 5563. CrossRef
4. Selected physical properties of new compounds are as follows. 2: Mp 242-244 ºC; IR (KBr) νmax = 3033w, 2946w, 1706vs, 1634vs, 1582s, 1498m, 1407s, 1379m, 1328m, 1195m, 1159m, 1104m, 882w, 824m, 767m, 732m, 608w, 574w cm–1; 1H NMR (CDCl3, 400 MHz) δ = 8.54 (s, 1H), 8.51 (dd, J = 7.9, 1.6 Hz, 1H), 8.34 (s, 1H), 7.71 (m, 2H), 7.524 (dd, J = 7.9, 1.6 Hz, 1H), 7.520 (dd, J = 7.9, 1.6 Hz, 1H), 7.47 (t, J = 7.9 Hz, 1H), 7.46 (t, J = 7.9 Hz, 1H), 7.41 (dd, J = 7.9, 1.2 Hz, 1H), 7.33 (m, 2H), –0.08 (dt, J = 9.6, 1.2 Hz, 1H), –0.13 (dt, J = 9.6, 1.2 Hz, 1H) ppm; UV-vis (CH2Cl2) λmax= 291 (logε = 4.66), 320sh (4.28), 335 (4.22), 347sh (4.13), 465 (3.85), 486 (3.83) nm; MS m/z (rel int) 334 (M+, 100), 333 (M+–1, 7), 305 (36), 277 (3), 167 (4), 153 (9), 127 (5). HRMS m/z Calcd for C23H14N2O (M+) 334.1106, found 334.1096. 3: Mp 279-281 ˚C. IR (ATR) νmax = 2925w, 1700s, 1634s, 1588s, 1498w, 1455m, 1406s, 1372m, 1331w, 1198m, 1157s, 1100m, 915s, 825s, 759s, 717s cm–1; 1H-NMR (CDCl3, 400 MHz) δ = 8.63 (s, 1H), 8.49 (dd, J = 7.9, 1.2 Hz, 1H), 8.47 (s, 1H), 7.75 (s, 2H), 7.67 (s, 1H), 7.65 (s, 1H), 7.53 (dd, J = 7.9, 1.2 Hz, 1H), 7.51 (dd, J = 7.9, 1.2 Hz, 1H), 7.459 (t, J = 7.9 Hz, 1H), 7.453 (t, J = 7.9 Hz, 1H), 7.36 (dd, J = 7.9, 1.2 Hz, 1H), 2.39 (s, 6H), 0.74 (dt, J = 9.6, 1.2 Hz, 1H), –0.27 (dt, J = 9.6, 1.2 Hz, 1H) ppm; UV-vis (CH2Cl2) λmax = 334 (logε = 4.59), 348sh (4.51), 387sh (4.17), 431sh (3.95), 494 (3.82) nm; MS m/z (rel int) 440 (M+, 26%), 412 (47), 216 (28), 97 (39), 55 (100). HRMS m/z Calcd for C30H20N2O2 (M+) 440.1525, found 440.1525. 6: a slightly yellow oil; 1H-NMR (CDCl3, 400 MHz) δ = 7.80 (s, 1H), 7.58 (s, 2H), 7.42 (d, J = 16.0 Hz, 1H), 7.20 (s, 1H), 6.83 (s, 1H), 6.09 (d, J = 16.0 Hz, 1H), 4.33 (q, J = 7.2 Hz, 2H), 4.24 (q, J = 7.2 Hz, 2H), 3.36 (s, 2H), 1.37 (t, J = 7.2 Hz, 3H), 1.32 (t, J = 7.2 Hz, 3H) ppm. 8: a yellow oil; 1H- NMR (CDCl3, 400 MHz) of the major stereoisomer, δ = 7.89 (s, 2H), 7.41 (s, 2H), 5.15 (d, J = 5.0 Hz, 2H), 4.36 (q, J = 7.0 Hz, 4H), 3.17 (qd, J = 7.6, 5.0 Hz, 2H), 1.38 (t, J = 7.0 Hz, 6H), 1.09 (d, J = 7.6 Hz, 6H), –0.22 (d, J = 9.6 Hz, 1H) , –0.27 (d, J = 9.6 Hz, 1H) ppm; 1H-NMR (CDCl3, 400 MHz) of the minor stereoisomer, δ = 7.82 (s, 2H), 7.31 (s, 2H), 5.35 (d, J = 5.0 Hz, 2H), 4.19 (q, J = 7.0 Hz, 4H), 2.97 (qd, J = 7.1, 5.0 Hz, 2H), 1.26 (t, J = 7.0 Hz, 6H), 1.04 (d, J = 7.1 Hz, 6H), 0.04 (d, J = 9.6 Hz, 1H) , –0.12 (d, J = 9.6 Hz, 1H) ppm. 9: yellow microcrystals, Mp >250 ºC (dec.); 1H-NMR (CDCl3, 400 MHz) δ = 8.52 (s, 2H), 7.70 (s, 2H), 7.61 (s, 2H), 2.39 (s, 6H), 0.74 (d, J = 9.2 Hz, 1H), –0.16 (d, J = 9.2 Hz, 1H) ppm. Assigment of the proton signals of 2 and 3, based on homodecoupling and NOE experiments, are as shown in Figure 7.
5. Y. Zhang, N. Terazawa, Y. Horino, A. Takai, Y. Tsuji, R. Miyatake, M. Oda, and S. Kuroda, Heterocycles, 2009, 77, 241. CrossRef
6. Compound 8 was obtained as an inseparable mixture of two stereoisomers. Signals for the two methyl groups on the seven-membered ring and the two ethyl groups of the esters appear to be equivalent for both stereoisomers in the 1H NMR spectrum, suggesting that these isomers have σ-symmetry through a plane containing both the carbon and hydrogen atoms of the methano bridge. Since both isomers have coupling constants of 5 Hz between adjacent methine protons on the seven-membered ring, relative stereochemistry between the methyl groups and the ether group is assigned as trans for both isomers, based on previously analyzed NMR data by Hoffmann et al. However, the stereochemical relationship between the ether and methano bridges has been obscure. H. M. R. Hoffmann, K. E. Clemens, and R. H. Smithers, J. Am. Chem. Soc., 1972, 94, 3940. CrossRef
7. For reviews on the [4 + 3] cycloadditions, see; J. H. Rigby and F. C. Pigge, Org. React., 1997, 51, 351; M. Harmata, Acc. Chem. Res., 2001, 34, 595; CrossRef M. Harmata and P. Rashatasakhon, Tetrahedron, 2003, 59, 2371; CrossRef H. M. R. Hoffmann, Angew. Chem., Int. Ed. Engl., 1984, 23, 1; CrossRef J. Mann, Tetrahedron, 1986, 42, 4611; CrossRef H. M. R. Hoffmann, Angew. Chem., Int. Ed. Engl., 1973, 12, 819; CrossRef R. Noyori and Y. Hayakawa, Org. React., 1983, 29, 163.
8. Y. Zhang, E. Hisano, R. Ohta, R. Miyatake, Y. Horino, M. Oda, and S. Kuroda, Tetrahedron Lett., 2008, 49, 888. CrossRef
9. Emission quantum yields were determined by comparison of the total emission area with that of anthracene (Φ = 0.27, upon excitation at 356 nm in ethanol). Corrections were made based on the refractive indices for the different solvents, n = 1.37 (ethanol) and n = 1.42 (dichloromethane). The small quantum yields of 2 and 3 may be attributed to the low-lying n–π* excited state.
10. For emission quenching of a 10H-pyrrolo[1,2-a]perimidin-10-one derivative with Cu(II) ion, see ref 3. For tuning the photoluminescent wavelength of a pyridine-capped oligothiophene by proton bonding, see; M. Kondo, J. Miyake, K. Tada, and N. Kawatsuki, Chem. Lett., 2011, 40, 264. CrossRef