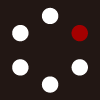
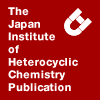
HETEROCYCLES
An International Journal for Reviews and Communications in Heterocyclic ChemistryWeb Edition ISSN: 1881-0942
Published online by The Japan Institute of Heterocyclic Chemistry
e-Journal
Full Text HTML
Received, 30th July, 2011, Accepted, 22nd August, 2011, Published online, 9th September, 2011.
DOI: 10.3987/COM-11-S(P)90
■ Structure and Mutagenicity of a Direct-Acting Mutagen Derived from the Reaction of N-Nitroso-N-methylbutylamine with Hydroxyl Radical
Keiko Inami, Motofumi Miura, Nozomi Tsutsumi, Eriko Okochi, Yoko Susaki, Satoko Ishikawa, Shigeyasu Motohashi, Junko Shiino, Kei Takeda, and Masataka Mochizuki*
Faculty of Pharmaceutical Sciences, Tokyo University of Science, 2641 Yamazaki, Noda, Chiba 278-8510,
Abstract
The mutagenicity of N-nitrosamines is usually detected in the presence of an S9 mix, which includes cytochrome P450. The mutagenicity of N-nitrosodialkylamines is induced by Fe2+-Cu2+-H2O2, which can be used as a chemical model for cytochrome P450. However, a direct-acting mutagen derived from N-nitroso-N-methylbutylamine (NMB) by the same oxidation system has not been reported. In this study, we determined the structure of a direct-acting mutagen obtained from the reaction of NMB with Fe2+-Cu2+-H2O2 by comparing its instrumental data (1H, 13C NMR and IR) with that from the synthesized compound. We confirmed that the direct-acting mutagen derived from NMB with Fe2+-Cu2+-H2O2 was 5-methyl-5-nitro-1-pyrazoline 1-oxide. Furthermore, we investigated the mechanism of the mutagenicity by 5-methyl-5-nitro-1-pyrazoline 1-oxide using Salmonella typhimurium strains. The mutagenicity of 5-methyl-5-nitro-1-pyrazoline 1-oxide in S. typhimurium YG7108, which is deficient O6-alkylguanine alkyltransferase, was higher than that in the parent strain S. typhimurium TA1535, indicating that the mutations are caused by DNA alkylation.INTRODUCTION
N-Nitrosamines are genotoxic chemical carcinogens that occur in the human diet and in the environment and can be formed endogenously in the human body.1,2 Since most N-nitrosamines cause a wide range of tumors in all the species tested so far,3 N-nitroso compounds are suspected to be causative agents for human cancer.4 The oxidative metabolism of nitrosamines is mediated by cytochrome P450 enzymes, which implies the formation of α-hydroxyalkylnitrosamines in animals and humans.5 The α-hydroxyalkylnitrosamines decompose spontaneously to give aldehydes and alkyldiazonium ions via the diazotates. Alkyldiazonium ions are considered to be the ultimate alkylating species formed by the oxidation of nitrosamines, and they react with nucleophilic substrates such as DNA bases. Almost all the nucleophilic oxygen and nitrogen centers in nucleic acid bases can be modified by the alkylating agents generated from nitrosamines.6 The predominant DNA adducts formed by nitrosamines are N-alkylpurines (7-alkylguanine and 3-alkyladenine), which are repaired by glucosylases such as 3-methyladenine DNA glycosylases. A minor amount of O6-methylguanine residues in DNA causes GC→AT transitions during replication. These DNA lesions can be detected by the Ames assay.7 We proposed a possibility of another activation pathway of N-nitrosodialkylamine by Fenton’s reagent supplemented with copper ion (Fe2+-Cu2+-H2O2). Fe2+-Cu2+-H2O2 activates N-nitrosodialkylamines, which has an alkyl chain longer than propyl, into a direct-acting mutagen.8,9 5-Ethyl-5-nitro-1-pyrazoline 1-oxide is a direct-acting mutagen derived from N-nitroso-N-methylpentylamine (NMPe) by Fe2+-Cu2+-H2O2.10 The aim of this study is to identify a direct-acting mutagen derived from N-nitroso-N-methylbutylamine (NMB; Figure 1) in the presence of Fe2+-Cu2+-H2O2.
RESULTS AND DISCUSSION
Identification of a mutagenic NMB oxidation product from NMB and Fe2+-Cu2+-H2O2
NMB and Fe2+-Cu2+-H2O2 were allowed to react in 1 M acetate buffer (pH 4.5) under nitric oxide gas for 2 h at 37 ºC. The reaction mixture was extracted with hexane, CCl4, CH2Cl2, and ethyl acetate consecutively. Each of the organic phases was dried over anhydrous sodium sulfate, and the solvent was subsequently evaporated. The mutagenicity of the CH2Cl2 extract was the highest among the organic phases. The CH2Cl2 extract was fractionated several times by silica gel column chromatography and by a preparative HPLC. A major product, which had no direct mutagenic activity, was identified by 1H-NMR and IR spectra as N-nitroso-N-methyl-3-oxobutylamine (yield 10%). The spectrum of the product was the same as that of an authentic sample (data not shown).
The fraction showing strong mutagenicity contained a single compound, which was recrystallized from CH2Cl2-hexane at a yield of 0.5% (colorless plates, mp 46.5-47.5 ºC). Spectral data of the isolated mutagen are shown in Table 1.
The molecular formula of the direct-acting mutagen was determined to be C4H7N3O3 by elemental analysis of C33.45, H4.84, and N28.60 (calculated for C33.10, H4.86, N28.96). The molecular formula (C4H7N3O3) indicated that the direct-acting mutagen was formed by the demethylation of NMB (C5H12N2O) and the addition of nitric oxide (NO). The involvement of NO in the formation of the direct-acting mutagen is in accordance with the literature.9
The 13C-NMR spectra showed four carbon signals, consistent with the number of carbons found by elemental analysis. The 1H-NMR spectrum suggested that the direct-acting mutagen had a cyclic structure due to the specific couplings observed. A proton signal at 2.13 ppm integrated 3 protons with a single peak, indicating the presence of a methyl group adjacent to a carbon atom. Two proton signals at 3.03 and 2.58 ppm changed to doublet signals (J=15.0 Hz) by decoupling at 4.29 ppm, indicating that the direct-acting mutagen was composed of -CH2CH2- with highly restricted structure such as a ring. A carbon resonance at δ 114.1 was a single peak by off-resonance 13C-NMR, indicating a tertiary carbon in the structure. The 1H- and 13C-NMR spectra indicated that the direct-acting mutagen was composed of -CH2CH2-C-CH3. The IR spectra at 1570 and 1526 cm-1 were characterized as nitro or nitrite groups. The EI-MS spectrum of the mutagen gave a molecular ion at m/z 145 and fragment ions at m/z 101 (M-N2O) and m/z 99 (M-NO2).
Since 5-ethyl-5-nitro-1-pyrazoline 1-oxide is the direct-acting mutagen derived from NMPe and Fe2+-Cu2+-H2O2 in the presence of NO, 5-methyl-5-nitro-1-pyrazoline 1-oxide was presumed to be the mutagen formed from the reaction of NMB with Fe2+-Cu2+-H2O2-NO. 5-Methyl-5-nitro-1-pyrazoline 1-oxide was synthesized by the reaction of 2-nitropropene and diazomethane, followed by the N-oxidation with m-CPBA (Scheme 1). The instrumental data (1H and 13C NMR and IR spectrum) of synthesized 5-methyl-5-nitro-1-pyrazoline 1-oxide was identical to that of the direct-acting mutagen isolated from the reaction extract.
The direct-acting mutagen appeared to have been formed by N-demethylation and ω-1 oxidation at the butyl group on NMB. It was reported that the longer n-alkyl chains of asymmetrical N-nitrosamines, the greater was the oxidation of methyl groups.11 The preferred position of the N-nitrosodibutylamine oxidation is greatest at the ω-1 position, which is in agreement with the position of the oxidation on the alkyl chains of NMB. The formation mechanism of the direct-acting mutagen from NMB and Fe2+-Cu2+-H2O2 has been presumed that hydroxyl radical formed from the metal ion and H2O2, oxidizes NMB to generate NO, the NO reacts with another NMB molecule in the presence of Fe2+ and Cu2+, followed by the formation of the direct-acting mutagen.9,10 The formation of 5-methyl-5-nitro-1-pyrazoline 1-oxide may occur through γ-nitrosation of the butyl group with NO and α-demethylation, followed by cyclization.
Mutagenicity of the 5-methyl-5-nitro-1-pyrazoline 1-oxide
We investigated the mechanism of mutagenicity of 5-methyl-5-nitro-1-pyrazoline 1-oxide by comparing its mutagenic potency in Salmonella typhimurium YG7108, which is deficient in O6-methylguanine methyltransferase, to the parent strain S. typhimurium TA1535.12,13 (Figure 2). The mutagenicity was greater in S. typhimurium YG7108, suggesting that it is due to DNA alkylation. Thus, the present direct-acting mutagen and α-hydroxynitrosamines induced mutation through the DNA alkylation.
The unique structure of the direct-acting mutagen is formed by NMB oxidation. N-Nitrosodialkylamines are known to be metabolically activated through α-hydroxylation, and our results reveal another possible activating pathway.
EXPERIMENTAL
Melting points were measured on a Yanagimoto microapparatus and were uncorrected. The EI-MS was obtained with a Hitachi M-4100 mass spectrometer. The NMR experiments were performed with a JEOL JNM-GX270 using tetramethylsilane as an internal standard. HPLC was performed using a Shimadzu LC-6A system [SPD-6AV UV/vis spectrometric detector, Lichrosorb Si 60 (10 µm, 7.5 × 250 mm)]. TLC was performed on precoated Kieselgel 60F254 (Merck), and spots were visualized under UV light. Column chromatography was performed on silica gel 60 (0.063 – 0.200 mm, Merck).
Chemicals. NMB was synthesized as described,14 and then purified by distillation (b.p. 87 ºC/18 mmHg).8 In order to decompose unknown direct-acting mutagen, crude NMB was dissolved in methanol saturated with sodium hydroxide and the entire solution was stirred overnight at room temperature. The reaction mixture was extracted three times with water and CH2Cl2, and the combined organic phases were dried over Na2SO4, filtered, and then evaporated in vacuo to produce a pale yellow oil. Bacto agar and bacto nutrient broth were obtained from Becton Dickinson Microbiology System (Sparks, USA). Sodium ammonium hydrogen phosphate tetrahydrate was purchased from Merck (Darmstadt, Germany). Copper (II) acetate monohydrate (Cu(OAc)2·H2O) was obtained from Kanto Chemical Co., Ltd. (Tokyo, Japan). Other reagents were purchased from Wako Pure Chemical Industries (Osaka, Japan). 2-Nitropropene was synthesized from 2-nitro-1-propanol as previously reported.15,16 Professor B. N. Ames (University of California, Berkeley, USA) kindly provided the S. typhimurium TA1535 and Dr T. Nohmi (National Institute of Health Sciences, Tokyo, Japan) kindly provided the S. typhimurium YG7108.
Bacterial mutation assay. The bacterial mutation assay was conducted according to the plate-incorporating method.12, 17 All of the yellow oil obtained was dissolved into DMSO. Five DMSO solutions with concentrations of 12.5, 25, 50, 100, and 200 µg / 50 µL were put into separate test tubes. Then 0.5 mL of 0.1 M sodium phosphate buffer (pH 7.4) and 0.1 mL of a culture of tester strain were added to each test tube, followed by 2 mL of top agar. The mixture from each tube was then poured onto a minimal-glucose agar plate. Colonies were counted after incubation for 44 h at 37 ºC. All plates were prepared in duplicate and the experiments were repeated at least twice. Data represent the means of duplicate determinations. The results were considered positive if the assay produced reproducible and dose-related increases in the number of revertants.17
The synthesized 5-methyl-5-nitro-1-pyrazoline 1-oxide was dissolved in 50 µL of acetonitrile and the assay was performed by the method as described above.
Reaction of NMB with Fe2+-Cu2+-H2O2-NO for isolation of the direct-acting mutagen. FeSO4·7H2O (28 g, 100 mmol) and Cu(OAc)2·H2O (20 g, 100 mmol) were added to a solution of NMB (13 g, 100 mmol) in acetate buffer (pH 4.5, 1 L), followed by the addition of 10% H2O2 (34 mL, 100 mmol). NO gas (250 mL, 100 mmol) was introduced to the reaction for 1 h under a nitrogen atmosphere, and the reaction mixture was incubated for 2 h at 37 ºC, and then was extracted twice with 100 mL hexane, twice with 100 mL CCl4, twice with 100 mL CH2Cl2, and twice with 100 mL EtOAc. Each organic phase was dried over Na2SO4, filtered, and evaporated in vacuo to produce a yellow oil. The CH2Cl2 extract showed the highest mutagenicity among the organic extracts. The crude product was purified by column chromatography twice (silica gel 60, hexane:CH2Cl2:Et2O=2:2:1, UV 254 nm), and by preparative HPLC (Lichrosorb Si60 7.5 x 250 mm 10 µm, hexane: CH2Cl2:EtOH=8:1:0.2, 2.0 mL / min, UV 254 nm) to produce a colorless oil. The oil was solidified by cooling, and then recrystallized from CH2Cl2 and hexane (mp 46.5–47.5 ºC).
Synthesis of 3-methyl-3-nitro-1-pyrazoline. Diazomethane was generated from N-methyl-N-nitroso-p-toluenesulfonamide (8.45 g, 39 mmol) with aqueous KOH.18 2-Nitropropene (2.03 g, 28 mmol) in 10 mL Et2O was slowly added to a solution of diazomethane in Et2O at -78 ºC, and the mixture was stirred. After 30 min, the reaction solution was allowed to warm to room temperature. After 3 h, the yellow color disappeared. The reaction mixture was washed twice with 50 mL water and 20 mL brine. The Et2O phase was dried over Na2SO4, filtered, and evaporated in vacuo to produce a yellow oil. The oil was purified chromatographically [Silica gel 60, hexane:CH2Cl2:Et2O=4:2:1, UV 254 nm or diphenylamine] to obtain 3-methyl-3-nitro-1-pyrazoline (yield 30%). 1H-NMR (CDCl3): δ 1.81 (1H, ddd, J=14.0, 9.2, 5.5 Hz, H-4), 2.00 (3H, s-CH3), 2.41 (1H, ddd, J=14.0, 9.2, 5.5 Hz, H-4), 4.79-4.93 (2H, m, H-5). IR (neat) cm-1: 1553, 1385.
Synthesis of 5-methyl-5-nitro-1-pyrazoline 1-oxide. Small portions of m-CPBA (860 mg, 5 mmol) were added to a solution of 3-methyl-3-nitro-1-pyrazoline (333 mg, 2.6 mmol) in 6 mL CH2Cl2 at room temperature. The reaction mixture was refluxed over 12 h, and 1 mL DMSO was added to decompose the excess m-CPBA. The reaction mixture was washed twice with 10 mL water and 5 mL brine, dried over Na2SO4, filtered, and evaporated in vacuo to obtain a residue. The crude product was purified by column chromatography (Silica gel 60, hexane:CH2Cl2:Et2O=2:2:1, UV 254 nm, diphenylamine, anisaldehyde/sulfuric acid), by a preparative HPLC (Lichrosorb Si 60, hexane: CH2Cl2:EtOH=8:1:0.4, UV 254 nm), and by a preparative TLC (silica gel, hexane:CH2Cl2:Et2O=4:4:1, UV 254 nm) to produce a colorless oil (yield 1%). 1H-NMR (CDCl3): δ2.13 (3H, s-CH3), 2.50-2.62 (1H, m, H-4), 2.96-3.06 (1H, m, H-4), 4.27-4.33 (2H, m, H-3). 13C-NMR (CDCl3): 20.9 (-CH3), 33.5 (C-4), 54.3 (C-3), 114.1 (C-5). IR (neat) cm-1: 1520, 1439, 1355, 1051. HR-FAB-MS calcd for C4H8O3N3 146.0566, found 146.0579.
ACKNOWLEDGEMENTS
This work was supported in part by a Grant-in-Aid for the Ministry of Education, Culture, Sports, Sciences and Technology of Japan.
References
1. R. Preussmann and G. Eisenbrand, ‘Chemical Carcinogens: N-Nitroso Carcinogens in the Environment,’ 2nd ed. by C. B. Searle, American Chemical Society, Washington DC, 1984, pp. 829-868; H. Bartsch and B. Spiegelhalder, Eur. J. Cancer Prev., 1996, 5, 1; CrossRef A. R. Tricker, Cancer Surv., 1987, 6, 226; R. C. Schothorst and R. W. Stephany, Int. J. Cosmet. Sci., 2001, 23, 109. CrossRef
2. M. Miwa, D. J. Stuehr, M. A. Marletta, J. S. Wishnok, and S. R. Tannenbaum, Carcinogenesis, 1987, 8, 955. CrossRef
3. H. Druckrey, R. Preussmann, S. Ivankovic, and D. Schmahl, Zeitschrift Krebsforsch, 1967, 69, 103. CrossRef
4. P. Jakszyn and C. A. González, World J. Gastroenterol., 2006, 12, 4296; F. Kamangar, W. Chow, C. Abnet, and S. Dawsey, Gastroenterol Clin. North Am., 2009, 38, 27. CrossRef
5. K. Inami, S. Ishikawa, and M. Mochizuki, Genes and Environment, 2009, 31, 97. CrossRef
6. B. Singer, Regul. Toxicol. Pharmacol., 1996, 23, 2; CrossRef D. E. G. Shuker and H. Bartsch, ‘DNA adduct,’ No. 125, ed. by K. Hemminki, A. Dipple, D. E. G Shuker, F. F. Kadlubar, D. Segerbäck, and H. Bartsch, IARC Scientific Publications, Lyon, 1994, pp. 73-89.
7. J. McCann, E. Choi, E. Yamasaki, and B. N. Ames, Pro. Nat. Aca. Sci., 1975, 72, 5135. CrossRef
8. K. Inami, S. Ishimura, Y. Akaike, E. Suzuki, N. Tsutsumi, K. Takeda, and M. Mochizuki, J. Health Sci., 2010, 56, 576. CrossRef
9. N. Tsutsumi, K. Inami, and M. Mochizuki, Bioorg. Med. Chem., 2010, 18, 8284. CrossRef
10. M. Miura, K. Inami, M. Yoshida, K. Yamaguchi, T. Mashino, and M. Mochizuki, Bioorg. Med. Chem., accepted, 2011.
11. G. Bellec, T. Goasduff, Y. Dreano, J. F. Ménez, and F. Berthou, Cancer Lett., 1996, 100, 115; CrossRef G. Bellec, Y. Dreano, R. Pichon, J. Ménez, and F. Berthou, Cancer Lett., 1996, 108, 171; CrossRef G. Bellec, Y. Dréano, J. P. Bail, J. F. Ménez, and F. Berthou, Mutat. Res., 1997, 377, 199. CrossRef
12. D. M. Maron and B. N. Ames, Mutat. Res., 1983, 113, 173.
13. M. Yamada, K. Matsui, T. Sofuni, and T. Nohmi, Mutat. Res., 1997, 381, 15. CrossRef
14. W. W. Hartman and L. J. Roll, Org. Synth., 1950, Coll. Vol. 2, 460.
15. H. Feuer, R. Miller, and C. B. Lawyer, J. Org, Chem., 1961, 26, 1357.
16. M. Miyashita, T. Yanami, and A. Yoshikoshi, Org. Synth., 1990, Coll. Vol. 7, 396.
17. K. Mortelmans and E. Zeiger, Mutat. Res., 2000, 455, 29. CrossRef
18. J. A. Moore and D. E. Reed, Org. Synth., 1950, Coll. Vol. 5, 351.