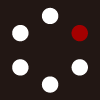
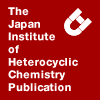
HETEROCYCLES
An International Journal for Reviews and Communications in Heterocyclic ChemistryWeb Edition ISSN: 1881-0942
Published online by The Japan Institute of Heterocyclic Chemistry
e-Journal
Full Text HTML
Received, 17th May, 2011, Accepted, 17th June, 2011, Published online, 30th June, 2011.
DOI: 10.3987/COM-11-S(P)24
■ A Flexible Convergent Route to Azaoxindoles, Azaindolines, Azaindoles, and Tetrahydroazaquinolones
Eric Bacqué, Myriem El Qacémi, and Samir Z. Zard*
Department of Chemistry, Ecole Polytechnique, CNRS UMR 7652, 91128 Palaiseau, France
Abstract
A variety of azaoxindoles, azaindolines, azaindoles, and tetrahydroazaquinolones can be readily prepared by cyclisation onto pyridine rings of radicals derived from xanthates. The xanthate precursors may themselves be produced by intermolecular radical addition-transfer to allylamino- or butenoylamide side-chains.In recent years, much effort has been directed to the synthesis of azaindoles and related derivatives.1 Such molecular scaffolds are related to the enormously important indoles and constitute therefore privileged structures in medicinal chemistry.2 Indeed, aza-analogs of indole-based active compounds often exhibit improved solubility and bioavailability. Access to these derivatives is however much more difficult than for the indole series. Transposition of the classical indole syntheses to their aza analogs is hampered either by the limited availability of the requisite precursors or by reactivity problems. For instance, the immensely powerful and widely used Fischer indole synthesis often gives poor yields and sometimes completely fails when applied to the pyridine series.3 Furthermore, very few pyridinylhydrazines are commercially available. In order to address this important synthetic problem, we have examined the potential of the xanthate transfer process for constructing additional rings around the pyridine nucleus. The degenerative transfer of xanthates and related groups we discovered some years ago has proved quite efficient at accomplishing difficult radical transformations such as intermolecular additions to unactivated alkenes and ring-closure onto aromatic rings.4 We have thus found that it was possible to prepare 7-azaoxindoles, 7-azaindolines, tetrahydro[1,8]naphthyridines, and tetrahydro-5H-pyrido[2,3-b]- azepin-8-ones by radical cyclisation onto the pyridine ring or by a combination of an intermolecular addition and a cyclisation.5
Additional examples of this approach to 7-azaindolines are displayed in Scheme 1. They illustrate the tolerance to functional groups, in particular to the presence of an iodide on the aromatic ring, as well as the rapidity with which a complex structure can be attained. In the first sequence, the intermediate adduct 3 arising from the addition of xanthate 2 to substrate 1 was not isolated but made to cyclise by treatment with a stoichiometric amount of lauroyl peroxide to give 7-azaindoline 4 in good overall yield. This derivative constitutes an ideal molecular scaffold for building large libraries of medicinally interesting compounds, since it contains several well-positioned functional groups with orthogonal chemical reactivity. For instance, the acetyl group can be easily cleaved to give 5 (and eventually replaced by other appendages), and a Sonogashira coupling selectively proceeds on the iodine to provide compound 6 in high yield. Suzuki-Miyaura and other organometallic couplings could in principle be applied, and the use of different starting xanthates would allow an expedient modification of the side chain functionality.
This last possibility is illustrated by the sequence starting from pyridine 7 and xanthate 8 and proceeding by way of adduct 9. The resulting 7-azaindoline 10 contains a masked 1,3-ketoaldehyde; its reaction with hydrazine under acidic conditions furnishes the corresponding pyrazole 11. The nature of the side chain may in principle be easily modified by using substituted hydrazines or other 1,2- or 1,3-bis nucleophiles, such as hydroxylamines, amidines, or guanidines.6
The two sequences in Scheme 1 highlight two procedures for constructing azaindoline structures: (a) a one-pot process where the intermolecular addition and ring closure are performed in the same flask by adding first a small amount of lauroyl peroxide to initiate the addition to the alkene, followed by portion-wise addition of a stoichiometric amount of peroxide to accomplish the ring-closure (prior two- to three-fold dilution with fresh solvent or replacement with a higher boiling solvent is often advantageous for the cyclisation step); (b) a two step process, where the first adduct, such as 9, is separated and characterised (hence the placement of lauroyl peroxide inside parentheses to indicate that it is used in sub-stoichiometric amounts). We have tended to favour the latter as it allows a better monitoring of the reaction progress and provides useful intermediates for various other transformations.
Our next task was to extend this very promising strategy to the synthesis of the other members in the series, namely 4-, 5-, and 6- azaoxindoles, azaindolines, as well as to aza-tetrahydroquinolones. In the case of the 4- and 6- isomers, a problem of regioselectivity arises, that will also need to be examined. Addressing first the azaoxindoles, we prepared 5-(N-t-butyl)amino-2-chloropyridine 15 by reaction of the 5-amino-2-chloropyridine 13 with commercially available O-t-butyliminoether 14 in the presence of boron trifluoride etherate (Scheme 2). This reaction proved to be very convenient for attaching a tertiary butyl group on aminopyridines and anilines; it is based on an earlier example by Anderson and co-workers.7 Chloroacetylation followed by displacement of the chloride with potassium O-ethyl xanthate furnished the desired precursor 16 in good overall yield. Exposure of this substance to the action of lauroyl peroxide in refluxing chlorobenzene resulted in a highly regioselective ring-closure to give 4-azaoxindole 18 in 52% yield; only traces of the isomeric 6-azaoxindole 19 could be detected. This is an unexpected and interesting result since the intermediate radical 17 is electrophilic in character, yet it prefers to attack the most electrophilic of the two possible reaction sites.8 The underlying causes for this high regioselectivity are still not clear. A survey of the limited number of radical ring-closures onto pyridine rings reported in the literature shows mostly a lack of selectivity and the general obtention of regioisomers.9 In any case, the clean formation of the 4-azaoxindole without the need for blocking position-4 on the pyridine ring represents a very convenient synthetic asset. The tertiary butyl group may be removed by treatment with a strong acid such as trifluoroacetic acid, as shown by the formation of azaoxindole 20 unsubstituted on the lactam nitrogen.
In order to obtain the 6-isomer, the position adjacent to the pyridine nitrogen had to be blocked. Thus, starting with 2-chloro-3-(N-methyl)aminopyridine 21, xanthate 22 was prepared in high yield by a similar procedure to that used for compound 16. Treatment with lauroyl peroxide in refluxing chlorobenzene afforded indeed the desired 6-azaoxindole 23 in 39% yield along with a small amount of reduced acetamide 24 (9%). The modest yield is due to restricted rotation caused by the presence of the adjacent chlorine. The unsubstituted amide 25 only gave reduced material 26 under all conditions tested, whereas N-t-butyl-substituted amide 27 underwent an unusual radical Smiles rearrangement proceeding through a spiro-β-lactam (Scheme 2).10 To complete the series, we prepared xanthate 30 from carbamate 28 and subjected it to the action of lauroyl peroxide in refluxing chlorobenzene. In this case, there are no regioselectivity issues, and the radical cyclisation provided 5-azaoxindole 31 in good yield.
In contrast to the approach to azaoxindoles, the synthesis of azaindolines and azaindoles using xanthates is modular, and therefore offers many possibilities for introducing variety. This is illustrated by the transformations depicted in scheme 3. Thus, intermolecular radical addition of xanthate 33 to N-allyl aminopyridine 32 furnished intermediate adduct 34, which was not isolated but further exposed to a stoichiometric amount of lauroyl peroxide to give isomeric indolines 35 and 36 in 68% and 18% yield respectively. Thus, in contrast to the corresponding case of the azaoxindole above (cf. 18), the regioselectivity is not complete, even if the cyclisation is also in favour of the 4-azaindoline isomer. As expected, placing a chlorine atom next to the pyridine nitrogen directs the cyclisation towards the 6-azaindoline regioisomer. This is shown by the clean conversion of N-allyl-3-amino-2-chloropyridine 37 into intermediate adduct 38, and then into 6-azaindoline 39 in moderate yield. The corresponding 6-azaindole 40 may be readily obtained by first removal of the Boc group with trifluoroacetic acid in dichloromethane then by oxidation with DDQ.
The synthesis of 5-azaindoline 44 proved particularly effective. The intermolecular radical addition of xanthate 42 to alkene 41 and the subsequent ring-closure could be accomplished in 75% overall yield without isolation of intermediate adduct 43.
The xanthate-based radical chemistry provides the intermediate radicals with sufficient lifetime to allow contemplating the extension of this strategy to the synthesis of tetrahydroazaquinolones (Scheme 4). Formation of 6-membered rings by radical cyclisation is usually more difficult and much less common than the corresponding 5-membered rings.11 We first tested the feasibility of this approach starting with symmetrical butenoylamide 45 to avoid complications due to regioselectivity. The intermolecular addition with xanthate 33 proceeded smoothly to give the corresponding adduct 46 in high yield. We were indeed relieved to find that exposure of xanthate 46 to stoichiometric amounts of lauroyl peroxide resulted in a reasonably efficient ring-closure to furnish tetrahydro-azaquinolone 47 in 51% yield. Of particular importance is the fact that the cyclisation is successful in the absence of a substituent on the amide nitrogen.12 The origin of the success is not clear in the present instance, for radical cyclisations generally fail with secondary amides because of slow rotation around the amide bond and the domination of the “wrong” rotamer, which is unable to undergo closure owing to its trans conformation.13
A similar sequence was performed starting with butenoylamide 48, which afforded tetrahydroazaquinolone 50 along with a small amount prematurely reduced amide 51, both through the intermediacy of radical adduct 49. The chlorine atom, which served to direct the regiochemistry of the cyclisation and to sterically block the nucleophilicity of the pyridine nitrogen and thus prevent any ionic degadation of the xanthate group, may be reductively removed by catalytic hydrogenation in the presence of ammonia. In this manner, compound 52 could be prepared in high yield.
A simple methyl group is sufficient to neutralise the untoward nucleophilicity of the pyridine nitrogen as illustrated by the conversion of butenoylamide 53 into adduct 54 and then into tetrahydroazaquinolone 55 in good overall yield, further demonstrating the synthetic potential of this novel chemistry.
In summary, we have developed a flexible approach to highly substituted and very diverse azaoxindoles, azaindolines, azaindoles, and tetrahydroazaquinolones, which complements the syntheses we described previously. Many of the compounds reported in the present work would be exceedingly tedious to make by more traditional routes. Indeed, the xanthate transfer technology represents a quite general solution to a longstanding problem in organic synthesis, namely the creation of a carbon-carbon bond in an intermolecular fashion starting with non-activated alkenes, as well as generalizing the ring closure onto aromatic and heteroaromatic structures.
ACKNOWLEDGEMENTS
We thank Sanofi-Aventis for generous financial support to one of us (M.E.)
References
1. For reviews, see: F. Popowycz, S. Routier, B. Joseph, and J.-Y. Mérour, Tetrahedron, 2007, 63, 1031; CrossRef F. Popowycz, J.-Y. Mérour, and B. Joseph, Tetrahedron, 2007, 63, 8689; CrossRef J. J. Song, J. T. Reeves, F. Gallou, Z. Tan, N. K. Yee, and C. H. Senanayake, Chem. Soc. Rev., 2007, 36, 1120; CrossRef H. Schirok, J. Org. Chem., 2006, 71, 5538; CrossRef G. R. Humphrey and J. T. Kuethe, Chem. Rev., 2006, 106, 2875; CrossRef S. Cacchi and G. Fabrizi, Chem. Rev., 2005, 105, 2873; CrossRef For recent examples, see: G. Calvet, M. Livecchi, and F. Schmidt, J. Org. Chem., 2011, 76, 4734; CrossRef S. H. Spergel, D. R. Okoro, and W. Pitts, J. Org. Chem., 2010, 75, 5316; CrossRef J. Lu, X. Cai, and S. M. Hecht, Org. Lett., 2010, 12, 5189; CrossRef J. L. Henderson, S. M. McDermott, and S. L. Buchwald, Org. Lett., 2010, 12, 4438; CrossRef S. Jana, M. D. Clements, B. K. Sharp, and N. Zheng, Org. Lett., 2010, 12, 3736; CrossRef M. M. Abd Rabo Moustafa and B. L. Pagenkopf, Org. Lett., 2010, 12, 3168; CrossRef D. K. Whelligan, D. W. Thomson, D. Taylor, and S. Hoelder, J. Org. Chem., 2010, 75, 11; CrossRef M. P. Huestis and K. Fagnou, Org. Lett., 2009, 11, 1357; CrossRef M. Jeanty, F. Suzenet, and G. Guillaumet, J. Org. Chem., 2008, 73, 7390; CrossRef C. Blaszykowski, E. Aktoudianakis, D. Alberico, C. Bressy, D. G. Hulcoop, F. Jafarpour, A. Joushaghani, B. Laleu, and M. Lautens, J. Org. Chem., 2008, 73, 1888; CrossRef P. Wipf and J. P. Maciejewski, Org. Lett., 2008, 10, 4383; CrossRef Y.-Q. Fang, J. Yuen, and M. Lautens, J. Org. Chem., 2007, 72, 5152; CrossRef X. Zheng and M. A. Kerr, Org. Lett., 2006, 8, 3777; CrossRef M. McLaughlin, M. Palucki, and I. W. Davies, Org. Lett., 2006, 8, 3307; CrossRef J. J. Song, Z. Tan, F. Gallou, J. Xu, N. K. Yee, and C. H. Senanayake, J. Org. Chem., 2005, 70, 6512; CrossRef A. Fayol and J. Zhu, Org. Lett., 2005, 7, 239; CrossRef S. Cacchi, G. Fabrizi, and L. M. Parisi, J. Comb. Chem., 2005, 7, 510; CrossRef C. Thibault, A. L'Heureux, R. S. Bhide, and R. Ruel, Org. Lett., 2003, 5, 5023; CrossRef Z. Zhang, Z. Yang, N. A. Meanwell, J. F. Kadow, and T. Wang, J. Org. Chem., 2002, 67, 2345. CrossRef
2. For a review, see: A. Zhang, J. L. Neumeyer, and R. J. Baldessarini, Chem. Rev., 2007, 107, 274; CrossRef For recent examples, see: T. McHardy, J. J. Caldwell, K.-M. Cheung, L. J. Hunter, K. Taylor, M. Rowlands, R. Ruddle, A. Henley, A. de Haven Brandon, M. Valenti, T. G. Davies, L. Fazal, L. Seavers, F. I. Raynaud, S. A. Eccles, G. W. Aherne, M. D. Garrett, and I. Collins, J. Med. Chem., 2010, 53, 2239; CrossRef D. Riether, C. Harcken, H. Razavi, D. Kuzmich, T. Gilmore, J. Bentzien, E. J. Pack, Jr., D. Souza, R. M. Nelson, A. Kukulka, T. N. Fadra, L. Zuvela-Jelaska, J. Pelletier, R. Dinallo, M. Panzenbeck, C. Torcellini, G. H. Nabozny, and D. S. Thomson, J. Med. Chem., 2010, 53, 6681; CrossRef T. Wang, Z. Yin, Z. Zhang, J. A. Bender, Z. Yang, G. Johnson, Z. Yang, L. M. Zadjura, C. J. D’Arienzo, D. DiGiugno Parker, C. Gesenberg, G. A. Yamanaka, Y.-F. Gong, H.-T. Ho, H. Fang, N. Zhou, B. V. McAuliffe, B. J. Eggers, L. Fan, B. Nowicka-Sans, I. B. Dicker, Q. Gao, R. J. Colonno, P.-F. Lin, N. A. Meanwell, and J. F. Kadow, J. Med. Chem., 2009, 52, 7778; CrossRef A. Echalier, K. Bettayeb, Y. Ferandin, O. Lozach, M. Clément, A. Valette, F. Liger, B. Marquet, J. C. Morris, J. A. Endicott, B. Joseph, and L. Meijer, J. Med. Chem., 2008, 51, 737; CrossRef Y. M. Choi-Sledeski, R. Kearney, G. Poli, H. Pauls, C. Gardner, Y. Gong, M. Becker, R. Davis, A. Spada, G. Liang, V. Chu, K. Brown, D. Collussi, R. Leadley, Jr., S. Rebello, P. Moxey, S. Morgan, R. Bentley, C. Kasiewski, S. Maignan, J.-P. Guilloteau, and V. Mikol, J. Med. Chem., 2003, 46, 681. CrossRef
3. M. Jeanty, J. Blu, F. Suzenet, and G. Guillaumet, Org. Lett., 2009, 11, 5142 and references therein; CrossRef Z. Zhang, Z. Yang, H. Wong, J. Zhu, N. A. Meanwell, J. F. Kadow, and T. Wang, J. Org. Chem., 2002, 67, 6226. CrossRef
4. For reviews, see: S. Z. Zard, Angew. Chem., Int. Ed. Engl., 1997, 36, 672; CrossRef B. Quiclet-Sire and S. Z. Zard, Phosphorus, Sulfur, Silicon, Related Elem., 1999, 137; CrossRef S. Z. Zard, in Radicals in Organic Synthesis, ed. by P. Renaud and M. P. Sibi, Wiley-VCH: Weinheim, 2001, Vol. 1, 90; CrossRef B. Quiclet-Sire and S. Z. Zard, Chem. Eur. J., 2006, 12, 6002; CrossRef B. Quiclet-Sire and S. Z. Zard, Top. Curr. Chem., 2006, 264, 201; CrossRef S. Z. Zard, Aust. J. Chem., 2006, 59, 663; CrossRef S. Z. Zard, Org. Biomol. Chem., 2007, 5, 205; CrossRef B. Quiclet-Sire and S. Z. Zard, Pure & Appl. Chem., 2011, 83, 519. CrossRef
5. E. Bacqué, M. El Qacemi, and S. Z. Zard, Org. Lett., 2004, 6, 3671; CrossRef See also: Y. Laot, L. Petit, N. D. M. Tran, and S. Z. Zard, Aust. J. Chem., 2011, 64, 416; CrossRef Y. Laot, L. Petit, and S. Z. Zard, Org. Lett., 2010, 12, 3426; CrossRef Y. Laot, L. Petit, and S. Z. Zard, Chem. Commun., 2010, 46, 5784; CrossRef M. El Qacemi, L. Ricard, and S. Z. Zard, Chem. Commun., 2006, 4422. CrossRef
6. The condensation of 1,3-diketones with hydrazines, hydroxylamines, amidines, and guanidines is a well-established route to pyrazoles, isoxazoles, pyrimidines, and aminopyrimidines. For general reviews, see: J. A. Joule and K. Mills, Heterocyclic Chemistry, Blackwell: Oxford, 2000; Comprehensive Heterocyclic Chemistry ed. by A. R. Katritzky and C. W. Rees, Pergamon: Oxford, 1984; Comprehensive Heterocyclic Chemistry II, ed. by A. R. Katritzky, C. W. Rees, and E. F. V. Scriven, Pergamon: Oxford, 1996; Comprehensive Heterocyclic Chemistry III, ed. by A. R. Katritzky, C. A. Ramsden, E. F. V. Scriven, and R. J. Taylor, Elsevier: Oxford, 2008.
7. J. C. Anderson, J. W. Cran, and N. P. King, Tetrahedron Lett., 2002, 43, 3849. CrossRef
8. For a similar observation, see: C. Leroi, B. Fenet, J.-L. Couturier, O. Guerret, and M. A. Ciufolini, Org. Lett., 2003, 7, 1079. CrossRef
9. D. C. Harrowven, B. J. Sutton, and S. Coulton, Org. Biomol. Chem., 2003, 1, 4047; CrossRef D. C. Harrowven, M. I. T. Nunn, N. J. Blumire, and D. R. Fenwick, Tetrahedron, 2001, 57, 4447; CrossRef D. C. Harrowven, B. J. Sutton, and S. Coulton, Tetrahedron Lett., 2001, 42, 9061; CrossRef D. C. Harrowven, M. I. T. Nunn, N. J. Blumire, and D. R. Fenwick, Tetrahedron Lett., 2000, 41, 6681; CrossRef D. C. Harrowven and M. I. T. Nunn, Tetrahedron Lett., 1998, 39, 5875; CrossRef W. B. Motherwell, A. M. K. Pennell, and F. Ujjainwalla, J. Chem. Soc., Chem. Commun., 1992, 1067; CrossRef M. L. E. N. De Mata, W. B. Motherwell, and F. Ujjainwalla, Tetrahedron Lett., 1997, 38, 141; CrossRef M. L. E. N. De Mata, W. B. Motherwell, and F. Ujjainwalla, Tetrahedron Lett., 1997, 38, 137; CrossRef R. A. Abramovitch and M. Saha, J. Chem. Soc. B, Phys. Org., 1966, 8, 733; R. A. Abramovitch, Can. J. Chem., 1960, 38, 2273. CrossRef
10. E. Bacqué, M. El Qacemi, and S. Z. Zard, Org. Lett., 2005, 7, 3817. CrossRef
11. B. Giese, B. Kopping, T. Göbel, J. Dickhaut, G. Thoma, K. J. Kulicke, and F. Trach, Org. React., 1996, 48, 301.
12. For related examples, see: B. Quiclet-Sire and S. Z. Zard, Chem. Commun., 2002, 2306; CrossRef G. Binot and S. Z. Zard, Tetrahedron Lett., 2005, 46, 7503. CrossRef
13. R. Yamasaki, A. Tanatani, I. Azumaya, S. Saito, K. Yamaguchi, and H. Kagechika, Org. Lett., 2003, 5, 1265, and references cited therein; CrossRef O. M. Musa, J. Horner, and M. Newcomb, J. Org. Chem., 1999, 64, 1022; CrossRef G. Stork and R. Mah, Heterocycles, 1989, 28, 723. CrossRef