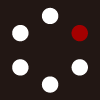
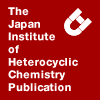
HETEROCYCLES
An International Journal for Reviews and Communications in Heterocyclic ChemistryWeb Edition ISSN: 1881-0942
Published online by The Japan Institute of Heterocyclic Chemistry
e-Journal
Full Text HTML
Received, 21st June, 2011, Accepted, 4th August, 2011, Published online, 15th August, 2011.
DOI: 10.3987/COM-11-S(P)43
■ Rapid One-Pot Versatile Preparation of 2-Aminobenzothiazoles by Highly Efficient Copper(I)-Catalyzed Inorganic Base-Free Intramolecular Cyclization
Haruki Sashida* and Mamoru Kaname
Faculty of Pharmaceutical Sciences, Hokuriku University, 3-Ho, Kanagawa machi, Kanazawa 920-1181, Japan
Abstract
The convenient and versatile one-pot preparation of the 2-aminobenzothiazoles by the highly efficient copper(I)-catalyzed intramolecular cyclization of commercially available o-halophenyl isothiocyanates with N-nucleophiles has been accomplished. The reaction of not only the o-iodophenyl or o-bromophenyl isothiocyanates, but also the o-chlorophenyl one proceeded under inorganic base-free conditions.INTRODUCTION
Various methods for the constructions of benzothiazole moieties2 are available because of their broad range of biological activities.3 A common approach for the synthesis of the 2-substituted benzothiazole involves the treatment of 2-aminothiophenol with acylating agents, aldehydes or benzyl halides (eq. 1).4 The copper catalyzed reaction of aromatic disulfide amines and aldehydes proceeds to give the benzothiazoles via the S-S bond fission (eq. 2).5 2-Aminobenzothiazoles are most often prepared by the coupling reaction of 2-haloanilines and isothiocyanates or cyclization of N-(2-halophenyl)thioureas, which are obtained by the reaction of 2-halophenyl isothiocyanates with amines or the acylation of 2-haloanilines, followed by treatment with Lawesson’s reagent. (eq. 3).6 The intramolecular cyclization of the N-(2-halophenyl)benzothioamides generally are efficient only for the synthesis of 2-phenyl-1,3-benzothiazoles (eq. 4).7 The syntheses of both the O- and S-substituted benzothiazoles were also achieved by the copper(I)-catalyzed reaction of 2-halophenyl isothiocyanates with O- or S- nucleophiles. (eq. 5)8 The copper-catalyzed thiolation reaction of N-(2-haloaryl)trifluoromethylacet- imidoyl chlorides with sulfides has been developed for synthesizing 2-trifluoromethylbenzothiazoles (eq.
6).9 Moreover, the 2-trifluoromethylbenzothiazoles have been synthesized via the C-H bond functionalization from trifluoroimidoyl chlorides and NaSH (eq. 7)10 In addition, the intramolecular cyclization of the unsubstituted thiobenzamides to the benzothiazoles via aryl radical cations by the phenyliodine(III) bis(trifluoroacetate) or thiyl radical using hipervalent iodine reagent has been developed (eq. 8).11
The majority of these methods include a transition metal-catalyst such as Pd or Cu, inorganic base and often a ligand. Moreover, the reactions frequently require heated conditions and very limited types of the 2-substituents on the benzothiazole ring.
RESULTS AND DISCUSSION
To overcome these drawbacks, we now describe a highly efficient method for the synthesis of the 1,3-benzothiazoles having an amino group such as NRR’ and NHR at the 2-position by the copper-catalyzed inorganic base-free one-pot tandem addition-cyclization reaction of 2-halophenyl thiocyanates (1) with N-nucleophiles without the isolation of the intermediates, thioureas, in our continuing studies on chalcogen-containing heterocycles.12,13
The treatment of 2-iodophenyl isothiocyanate (1A, 1.0 mmol) with piperidine (2a, 2.5 mmol) in the presence of CuI (10 mol %) in benzene at room temperature resulted in the direct ring-closure to give 2-piperidino-1,3-benzothiazole (3a) in 100% isolated yield; the reaction took place very rapidly and was completed within a few minutes under an air atmosphere (Table 1, entry 1). When 5 and 1 mol % of CuI were allowed to react with the isothiocyanate (1A) and piperidine (2a) under the same conditions, the 1,3-benzothiazole (3a) was formed in 98 and 99% isolated yields, respectively (entries 2 and 3). However, 3a was only slightly formed in the absence of CuI (entry 4), in addition, the use of a 1.0 equivalent of piperidine did not give the desired cyclized product irrespective of the amount of the catalyst; only the thiourea 4Aa was obtained in 94% yield (entry 5). Both the monovalent (e.g., CuBr and CuCl) and bivalent copper-catalyst (e.g., CuBr2, Cu(OTf)2, CuSO4 and Cu(OH)2) were almost equally effective; the reaction occurs under copper catalytic conditions (entries 6-11). Fortunately, this tandem addition-cyclization reaction of both the 2-bromophenyl (1B) and 2-chlorophenyl isothiocyanates (1C) also took place under inorganic base-free similar mild conditions at room temperature to produce the benzothiazole (3a), although the reaction time had to be increased for completion; 1B and 1C are quite superior to 1A regarding cost of the substrates (entries 12-17). 3a was obtained using 10 mol % CuI
within 24 h from 1B and 1C in quantitative yields, respectively (entries 13 and 16). The heating of the isothiocyanates (1B, 1C) with piperidine (2a) under 5 mol % CuI conditions in refluxing benzene for 15-20 h afforded the benzothiazole (3a) in excellent yields (entries 14 and 17). The use of benzene as a solvent afforded the best results. The thiazole 3a was obtained in trace by the addition of a palladium catalyst (entry 18).
Previously reported methods8 for the syntheses of the benzothiazoles from the 2-halophenyl isothiocyanates and nucleophiles require a metal-catalyst, an inorganic base such as Na2CO3, NaOH, K2CO3, KOt-Bu, K3PO4, and Cs2CO3, various ligands, and often higher reaction temperature (eq. 5, Scheme 1). However, in our present investigation, the reaction smoothly proceeded to give the expected benzothiazoles without any inorganic bases, ligands at room temperature, and even o-chlorophenyl isothiocyanate. Thus, in order to clarify the details of this difference in the reported methods including the mechanism, we tried to develop more experimentation (Scheme 2). When the treatment of the thiourea 4Aa, which was easily obtained by the reaction of the isothiocyanate (1A) and 1.0 equivalent amount of piperidine (2a) in benzene at room temperature, with CuI (5 mol %) as the catalyst in benzene, the reaction did not take place at all. However, the reaction very rapidly proceeded and finished within a few minutes by the addition of piperidine (2a) at room temperature. With the addition of piperidine in the absence of CuI, the thiazole 3a was not obtained from the thiourea 4Aa. Similarly, when diethylamine or triethylamine as an aliphatic amine was employed, the corresponding product 3a was almost quantitatively obtained; the use of triethylamine required a long reaction time (e.g., 20-24 h). In these cases, using inorganic bases and ligands are not essential. This fact evidently indicates that the secondary amine operated as a nucleophile toward the isothiocyanate moiety at the first addition step, and had an effect as a base to remove HX on the second elimination step.
Next, the extension of this tandem addition-cyclization of 2-halophenyl isothiocyanates (1) with several amine nucleophiles (2) was carried out and the obtained results are summarized in Table 2. The present cyclization of the 2-halophenyl isothiocyanates (1) with aliphatic, aromatic, secondary and primary mono and diamines almost quantitatively proceeded except for o-phenylenediamine (2n).2-Piperidinobenzothiazole (3a) was quantitatively obtained from not only o-iodophenyl, but also from the o-bromophenyl
(1B) and o-chlorophenyl isothiocyanates (1C) (entries 1-3, also Table 1, entries 13, 17). o-Iodophenyl isothiocyanate (1A) similarly reacted with 2.5 equivalents of the secondary amine, pyrrolidine (2b) and morpholine (2c) in the presence of CuI (5 mol %) in benzene at room temperature to produce the corresponding 2-aminobenzothiazoles (3b and 3c) in 95 and 97% yields, respectively (entries 4, 5). 2-(Diethylamino)benzothiazole (3d) was also produced by the reaction of 2-iodophenyl (1A) and 2-bromophenyl isothiocyanate (1B) with diethylamine (2d) in excellent yields (entries 6, 7). Unfortunately, 3d was not produced from 2-chlorophenyl isothiocyanate (1C) and diethylamine (2d); only a complex mixture involving the thiourea 4Ca was obtained. When n-butyl (2e) and t-butyl amines (2f) as a primary aliphatic amine were allowed to react with 1A under similar conditions, the corresponding 2-aminobenzothiazoles (3e and 3f) were also obtained in 100 and 93% yields, respectively, although a prolonged reaction time was needed (entries 9, 10). Aromatic amines, aniline (2g), N-methylaniline (2h) and imidazole (2i) also afforded the corresponding products (3g, 3h, 3i) in good yields (entries 11-13). It is also notable that even when a diamine, such as piperazine and propylenediamine, were employed, the symmetrical products 3j and 3k were obtained in 92 and 81% yields, respectively (entries 14, 15). The reaction of three kinds of structural isomers of the phenylenediamine with isothiocyanate 1A was examined. m-Phenylenediamine (2l) and p-phenylenediamine (2m) reacted with 1A to give the normal 1:2 adducts 3l and 3m in good yields as the sole products (entries 16, 17). On the contrary, o-phenylenediamine (2n) afforded the 1:1 cyclization product 5 (17% yield) and the imidazole derivative 6 (13% yield) in addition to the normal expected 1:2 adducts, the bis(benzothiazoyl) derivaive (3n) (13% yield) as shown in Scheme 3. The mono-benzothiazole (5) was produced by the copper-catalyzed ring-closure of the monothiourea (4An). The formation of the imidazole (6) may be explained as follows: the intramolecular nucleophilic attack of the benzothiazol-2-yl-amino moiety on the thiocarbonyl carbon in the mono ring-closure intermediate 8
generated the sulfanylimidazole intermediate (9), followed by dehydrosulfidation to give 6. The mechanism for the formation of 6 through 8 and 9 is essentially similar to that of the 2-aminobenzimidazoles from the isoselenocyanates reported by Xie and co-workers.14
CONCLUSION
In summary, we have developed a simple and practical protocol for synthesizing the benzothiazole moieties having various amino groups at the C-2 position by the copper-catalyzed tandem addition-cyclization reaction of 2-halophenyl isothiocyanates with N-nucleophiles in a one-pot reaction. Most importantly, by comparing the reported methodology, this protocol allows the C-S bond formation under inorganic base-free conditions, the products are obtained in high yields, and all the starting materials, e.g., the 2-haolophenyl isothiocyanates and amines, are commercially available.
EXPERIMENTAL
Melting points were measured on a Yanagimoto micro melting point hot stage apparatus and are uncorrected. IR spectra were determined with a Horiba FT-720 spectrometer. Mass spectra (MS) and HRMS were recorded on a JEOL JMS-DX300 instrument. NMR spectra were determined with a JEOL JNM-GSX 500 (500 MHz) spectrometer in CDCl3 or DMSO-d6 using tetramethylsilane as internal standard and J values are given in Hz. Microanalyses were performed in the Microanalytical Laboratory in this Faculty.
2-Bromophenyl isothiocyanate, 2-chlorophenyl isothiocyanate, 2-iodophenyl isothiocyanate and all amines are commercially available.
Method I: To a stirred mixture of 2-iodophenyl isothiocyanate (1A, 261 mg, 1 mmol) and an appropriate amine (2, 2.5 mmol) in benzene (2.5 mL) at room temperature was added CuI (0.01 mmol) at room temperature. The mixture was stirred at same conditions until disappearance of the starting material by the TLC check and evaporated in vacuo. The obtained residue was purified by silica gel chromatography using CHCl3-MeOH (100:3) as eluent to give pure 2-aminobenzothiazole (3).
Method II: The 2-halophenyl isothiocyanate (1) was treated with CuI (0.05 mmol) and worked up as described for the method I.
Method III: The 2-halophenyl isothiocyanate (1) was treated with CuI (0.1 mmol) and worked up as described for the method I.
Method IV: To a stirred mixture of 2-iodophenyl isothiocyanate (1A, 261 mg, 1 mmol) and an appropriate amine (2, 2.5 mmol) in benzene (2.5 mL) at room temperature was added CuI (0.05 mmol). The mixture was refluxed until disappearance of the starting material and evaporated in vacuo. The obtained residue was purified by silica gel chromatography using CHCl3-MeOH (100:3) as eluent to give pure 2-aminobenzothiazole (3).
Method V: To a stirred mixture of 2-iodophenyl isothiocyanate (1A, 261 mg, 1 mmol), an appropriate amine (2, 1.0 mmol) and Et3N (10 mmol) in benzene (2.5 mL) was added CuI (0.05 mmol). The mixture was refluxed until disappearance of the starting material and evaporated in vacuo. The obtained residue was purified by silica gel chromatography using CHCl3-MeOH (100:3) as eluent to give pure 2-aminobenzothiazole (3).
Method VI: The 2-halophenyl isothiocyanate (1) was treated with the amine (2, 0.5 mmol) and worked up as described for the method V.
The benzothiazoles (3a-i) were identical with the authentic samples; the melting points and the spectral data of the products were in good agreement with those already reported data except for 3d, 3e, 3f, 3h and 3i, and the melting point of 3i is not described in the literature.7g Thus, the 1H and 13C NMR data of them are reported here.
2-(Piperin-1-yl)benzo[d]thiazole (3a)
Colorless needles, mp 95.5-96.5 ˚C (from CHCl3-hexane), lit.15 mp 93-94 ˚C.
2-(Pyrrolin-1-yl)benzo[d]thiazole (3b)
Colorless prisms, mp 102-103 ˚C (from CHCl3-hexane), lit.7f mp 101 ˚C.
2-(Morpholin-yl)benzo[d]thiazole (3c)
Colorless plates, mp 118-119.5 ˚C (from AcOEt-hexane), lit.16 mp 119-120 ˚C.
2-(Diethylamino)benzo[d]thiazole (3d)
Pale yellow oil, lit.7f 1H NMR (500 MHz, CDCl3) δ = 1.27 (6H, t, J = 7.2 Hz), 3.56 (4H, q, J = 7.2 Hz), 7.02 (1H, ddd, J = 7.9, 7.4, 1.1 Hz), 7.26 (1H, ddd, J = 8.1, 7.4, 1.3 Hz), 7.53 (1H, dd, J = 8.1, 1.1 Hz), 7.56 (1H, dd, J = 7.9, 1.3 Hz). 13C NMR (125 MHz, CDCl3) δ = 12.9 (q), 45.4 (t), 118.5 (d), 120.5 (d), 120.7 (d), 125.8 (d), 130.6 (s), 153.3 (s), 167.3 (s).
2-(n-Butylamino)benzo[d]thiazole (3e)
Colorless prisms, mp 85-86 ˚C (from CHCl3-hexane), lit.17 mp 68 ˚C. 1H NMR (500 MHz, CDCl3) δ = 0.94 (3H, t, J = 7.4 Hz), 1.38-1.47 (2H, m), 1.62-1.70 (2H, m), 3.39 (2H, t, J = 7.2 Hz), 6.21-6.36 (1H, br, NH), 7.06 (1H, ddd, J = 7.9, 7.3, 1.1 Hz), 7.28 (1H, ddd, J = 8.1, 7.3, 1.2 Hz), 7.50 (1H, dd, J = 8.1, 1.1 Hz), 7.58 (1H, dd, J = 7.9, 1.2 Hz). 13C NMR (125 MHz, CDCl3) δ = 13.7 (q), 20.0 (t), 31.6 (t), 45.5 (t), 118.5 (d), 120.8 (d), 121.2 (d), 125.9 (d), 130.2 (s), 152.5 (s), 168.1 (s).
2-(tert-Butylamino)benzo[d]thiazole (3f)
Colorless needles, mp 96-96.5 ˚C (from CHCl3-hexane), lit.18 mp 91-95 ˚C (from EtOAc-hexane). 1H NMR (500 MHz, CDCl3) δ = 1.48 (9H, s), 5.20-5.32 (1H, br, NH), 7.06 (1H, ddd, J = 7.9, 7.3, 1.2 Hz), 7.27 (1H, ddd, J = 8.1, 7.3, 1.3 Hz), 7.54 (1H, dd, J = 8.1, 1.2 Hz), 7.56 (1H, dd, J = 7.9, 1.3 Hz). 13C NMR (125 MHz, CDCl3) δ = 29.1 (q), 53.3 (s), 119.0 (d), 120.4 (d), 121.4 (d), 125.7 (d), 130.8 (s), 152.4 (s), 164.6 (s).
2-Anilinobenzo[d]thiazole (3g)
Colorless needles, mp 163-165 ˚C (from CHCl3-hexane), lit.19 mp 159-160 ˚C.
2-(N-Methylanilino)benzo[d]thiazole (3h)
Colorless prisms, mp 76-78 ˚C (from hexane), lit.20 oil. 1H NMR (500 MHz, CDCl3) δ = 3.62 (3H, s), 7.05, 7.38-7.50, 7.62 (1H, ddd, J = 7.7, 7.1, 0.9 Hz, 2H, m, 5H, m, 1H, dd, J = 8.1, 0.5 Hz). 13C NMR (125 MHz, CDCl3) δ = 40.4 (q), 119.2 (d), 120.4 (d), 121.7 (d), 125.8 (d), 126.0 (s), 127.4 (d), 129.9 (d), 131.2 (s), 145.8 (s), 152.6 (s), 168.2 (s).
2-(Imidazol-1-yl)benzo[d]thiazole (3i)
Colorless prisms, mp 135-138 ˚C (from CHCl3-hexane), lit.7g 1H NMR (500 MHz, CDCl3) δ = 7.22 (1H, s), 7.39 (1H, ddd, J = 8.0, 7.4, 1.1 Hz), 7.50 (1H, ddd, J = 8.2, 7.4, 1.2 Hz), 7.61 (1H, s), 7.81 (1H, dd, J = 8.0, 1.2 Hz), 7.92 (1H, dd, J = 8.2, 1.1 Hz), 8.29 (1H, s). 13C NMR (125 MHz, CDCl3) δ = 117.8 (d), 121.6 (d), 122.8 (d), 125.5 (d), 127.1 (d), 131.2 (d), 132.1 (s), 135.9 (d), 150.5 (s), 155.8 (s).
1,4-Bis (benzo[d]thiazol-2-yl)piperazine (3j)
Colorless prisms, mp 292-293 ˚C (from CHCl3). 1H NMR (500 MHz, CDCl3) δ = 3.82 (8H, s, CH2 x 4), 7.12, 7.33, 7.59, 7.63 (2H, ddd, J = 7.8, 7.3, 1.2 Hz, 2H, ddd, J = 8.1, 7.3, 1.3 Hz, 2H, dd, J = 8.1, 1.2 Hz, 2H, dd, 7.8, 1.3 Hz). 13C NMR (125 MHz, CDCl3) δ = 47.8 (t), 119.5 (d), 120.8 (d), 121.9 (d), 126.2 (d), 130.8 (s), 152.5 (s), 168.4 (s). MS (FAB): m/z (relative intensity, %) 353 (7, MH+), 134 (57), 119 (64), 84 (93), 39 (100). HRMS (FAB) m/z MH+ calcd for C18H17N4S2: 353.0895, found: 353.0899.
N, N’-Bis(benzo[d]thiazol-2-yl)1,3-diaminopropane (3k)
Colorless prisms, mp 193-198 ˚C (from CHCl3-hexane). IR (KBr, tab.): 3437 (NH) cm-1. 1H NMR (500 MHz, CDCl3) δ = 1.90-2.15 (2H, m, CH2CH2CH2), 3.44-3.53 (4H, m, CH2CH2CH2), 7.02 (2H, dd, J = 7.8, 7.5 Hz), 7.22 (2H, ddd, J = 7.9, 7.5, 1.0 Hz), 7.40 (2H, d, J = 7.9 Hz), 7.66 (2H, dd, J = 7.8, 1.0 Hz), 8.10 (2H, t, J = 5.2 Hz, NH). 13C NMR (125 MHz, DMSO-d6) δ 28.3 (t), 41.6 (t), 117.9 (d), 120.75 (d), 120.79 (d), 125.4 (d), 130.2 (s), 152.5 (s), 166.0 (s). MS (EI): m/z (relative intensity, %) 340 (47, M+), 190 (37), 177 (100), 150 (34), 136 (40). HRMS (EI) m/z M+ calcd for C17H16N4S2: 340.0816, found: 340.0819.
N,N’-Bis(benzo[d]thiazol-2-yl)-m-phenylenediamine (3l)
A mixture of isothiocyanate (1A, 261 mg, 1 mmol), m-phenylenediamine (2l, 54 mg, 0.5 mmol), Et3N (0.14 mL, 10 mmol) and CuI (10 mg) in benzene (2.5 mL) was refluxed for 48 h. The obtained precipitate was filtered off and washed with H2O and CHCl3.
Colorless powder, mp 263-265 ˚C (from DMF-H2O). IR (KBr, tab.): 3430 (NH) cm-1. 1H NMR (500 MHz, DMSO-d6) δ = 7.18 (2H, ddd, J = 7.8, 7.1, 1.1 Hz), 7.30-7.41 (3H, m), 7.50 (2H, dd, J = 8.1, 1.9 Hz), 7.63 (2H, dd, J = 7.9, 7.9 Hz), 7.83 (2H, dd, J = 7.8, 7.8 Hz), 8.25 (1H, s), 10.57 (2H, s, NH x 2). 13C NMR (125 MHz, DMSO-d6) δ = 107.1 (d), 111.8 (d), 119.0 (d), 121.0 (d), 122.2 (d), 125.8 (d), 129.4 (d), 130.0 (s), 141.0 (s), 152.0 (s), 161.5 (s). MS (EI): m/z (relative intensity, %) 374 (100, M+), 282 (7), 224 (15). HRMS (EI) m/z M+ calcd for C20H14N4S2: 374.0660, found: 374.0659.
N,N’-Bis(benzo[d]thiazol-2-yl)-p-phenylenediamine (3m)
p-Phenylenediamine (2m, 54 mg, 0.5 mmol) was similarly treated with isothiocyanate (1A, 261 mg, 1 mmol) and worked up as described for the preparation of 3l to give 3m.
Colorless needles, mp 287-290 ˚C (from DMF-H2O). IR (KBr, tab.): 3430 (NH) cm-1. 1H NMR (500 MHz, DMSO-d6) δ = 7.15 (2H, dd, J = 7.7, 7.6 Hz), 7.33 (2H, dd, J = 7.8, 7.4 Hz), 7.60 (2H, d, J = 8.0 Hz, 7.75-7.85 (6H, m), 10.45 (2H, s, NH x 2). 13C NMR (125 MHz, DMSO-d6) δ = 118.7 (d), 118.9 (d), 120.9 (d), 122.0 (d), 125.7 (d), 129.9 (s), 135.3 (s), 152.1 (s), 161.7 (s). MS (EI): m/z (relative intensity, %) 374 (100, M+), 224 (8). HRMS (EI) m/z M+ calcd for C20H14N4S2: 374.0660, found: 374.0665.
Reaction of o-phenlenediamine (2n) with isothiocyanate (1A)
A mixture of isothiocyanate (1A, 261 mg, 1 mmol), o-phenylenediamine (2n, 54 mg, 0.5 mmol), Et3N (0.14 mL, 10 mmol) and CuI (10 mg) in benzene (2.5 mL) was refluxed for 48 h. The obtained mixture was purified by silica gel chromatography using CHCl3-MeOH (100:3) as eluent to give pure 2-aminobenzothiazole (3n), 5 and 6.
N,N’-Bis(benzo[d]thiazol-2-yl)-o-phenilenediamine (3n)
Colorless needles, mp 296-300 ˚C (from CHCl3-hexane). IR (KBr, tab.): 3379 (NH) cm-1. 1H NMR (500 MHz, CDCl3) δ = 7.00 (2H, ddd, J = 8.0, 7.8, 1.1 Hz), 7.20 (2H, ddd, J = 8.0, 7.3, 1.2 Hz), 7.31 (2H, dd, J = 6.0, 3.5 Hz), 7.34 (2H, d, J = 7.8 Hz), 7.38 (2H, d, J = 8.0 Hz), 7.84 (2H, dd, J = 6.0, 3.5 Hz), 8.85-10.00 (2H, br, NH). 13C NMR (125 MHz, CDCl3) δ 118.9 (d), 120.7 (d), 122.2 (d), 124.6 (d), 126.0 (d), 126.7 (d), 129.9 (s), 133.9 (s), 150.8 (s), 166.1 (s). MS (EI): m/z (relative intensity, %) 374 (100, M+), 341 (33), 239 (29), 225 (39). HRMS (EI) m/z M+ calcd for C20H14N4S2: 374.0660, found: 374.0655.
2-[(2-Aminophenyl)amino]benzo[d]thiazole (5)
Colorless pinky prisms, 165-167 ˚C (from CHCl3-hexane). IR (KBr, tab.): 3444, 3425, 3377, 3340 (NH) cm-1. 1H NMR (500 MHz, CDCl3) δ = 3.90-4.15 (2H, brs, NH2), 6.81-6.89 (2H, m), 7.07 (1H, ddd, J = 7.8, 7.7, 1.0 Hz), 7.18 (1H, ddd, J = 8.0, 7.9, 1.4 Hz), 7.24 (1H, ddd, J = 7.9, 7.5, 1.3 Hz), 7.36-7.44 (2H, m), 7.52 (1H, dd, J = 7.8, 0.7 Hz), 9.25-9.65 (1H, brs, NH). 13C NMR (125 MHz, CDCl3) δ 116.6 (d), 118.7 (d), 119.2 (d), 120.9 (d), 121.9 (s), 125.7 (s), 126.0 (d), 127.6 (d), 128.7 (d), 130.3 (s), 142.9 (s), 151.7 (s), 169.3 (s). MS (EI): m/z (relative intensity, %) 241(100, M+), 225 (45), 208 (88). HRMS (EI) m/z M+ calcd for C13H11N3S: 241.0674, found: 241.0677.
{2-[(2-Iodophenyl)amino]benz[d]imidazol-1-yl}benzo[d]thiazole (6)
Colorless prisms, 196-198 ˚C (from CHCl3-hexane). IR (KBr, tab.): 3442 (NH) cm-1. 1H NMR (500 MHz, CDCl3) δ = 6.81 (1H, ddd, J = 7.7, 7.5, 1.3 Hz), 7.24 (1H, ddd, J = 8.0, 7.6, 0.8 Hz), 7.32 (1H, dd, J = 7.4, 7.4 Hz), 7.40 (1H, ddd, J = 8.0, 7.3, 0.7 Hz), 7.45 (1H, ddd, J = 8.5, 7.1, 1.4 Hz), 7.53 (1H, ddd, J = 8.1, 7.3, 0.8 Hz), 7.62 (1H, d, J = 7.8 Hz), 7.81-7.92 (3H, m), 8.08 (1H, d, J = 8.1 Hz), 8.84 (1H, dd, J = 8.3, 1.4 Hz), 11.25 (1H, s, NH). 13C NMR (125 MHz, CDCl3) δ 88.3 (s), 110.3 (d), 118.2 (d), 118.2 (d), 120.6 (d), 121.3 (d), 122.1 (d), 124.3 (d), 124.4 (d), 125.2 (d), 127.1 (d), 129.2 (d), 130.3 (s), 130.9 (s), 139.3 (d), 140.6 (s), 142.6 (s), 148.5 (s), 149.2 (s), 157.2 (s). MS (EI): m/z (relative intensity, %) 468 (81, M+), 341 (100). HRMS (EI) m/z M+ calcd for C20H13N4SI: 467.9906, found: 467.9903.
N-(2-Iodophenyl)piperidine-1-carbothioamide (4Aa)
Piperidine (2a, 179 mg, 2.1 mmol) was added to a stirred solution of 2-iodophenyl isothiocyanate (1A, 522 mg, 2.0 mmol) in benzene (5 mL) at room temperature. The mixture was stirred for 5 min, and evaporated in vacuo. The obtained residual solid was recrystallized from benzene-hexane to give pure adduct 4Aa. Yied: 657 mg (95%). Colorless prisms, mp 109-110.5 ˚C. IR (KBr-tab): cm-1. 1HNMR (500 MHz, CDCl3) δ = 1.70, 3.84 (6H, br s, 4H, br s, CH2 x 5), 6.89, 7.33, 7.51, 7.81 (1H, ddd, J = 8.0, 7.4, 1.6 Hz, 1H, ddd, J = 8.1, 7.4, 1.4 Hz, 1H, dd, J = 8.1, 1.6 Hz, 1H, dd, J = 8.0, 1.4 Hz), 7.03 (1H, br, NH). 13CNMR (125 MHz, CDCl3) δ: 24.2 (t), 25.6 (t), 50.6 (t), 94.5 (s), 125.6 (d), 126.8 (d), 128.7 (d), 139.0 (d), 141.2 (s) 181.6 (s). MS (FAB): m/z (relative intensity, %) 347 (100, M+), 219 (94), 128 (30), 84 (20). HRMS (FAB): m/z M+ calcd for C12H16N2IS: 347.0079; found: 347.0072.
Treatment of 4Aa with CuI and piperidine
Piperidine (2a, 213 mg, 2.5 mmol) and CuI (20 mg, 0.1 mmol) were added to a stirred solution of 4Aa (347 mg, 1 mmol) in benzene (2.5 mL) at room temperature. The mixture was stirred for 5 min, and evaporated in vacuo. The obtained residual solid was recrystallized from benzene-hexane to give pure adduct 3a. Yield: 213 mg (98%).
ACKNOWLEDGEMENTS
This work was supported in part by a Grant-in Aid for Scientific Research from the Ministry of Education, Science and Culture, Japan. Thanks are due to Mr. Takahiro Teramae (Hokuriku University) for his technical assistance.
References
1. Studies on Chalcogen-Containing Heterocycles. Part 35, For Part 34: M. Kaname and H. Sashida, Teterahedron Lett., 2011, 52, 3279. CrossRef
2. (a) S. L. Khokra, K. Arora, H. Mehta, A. Aggarwal, and M. Yadav, International Journal Pharmaceutical Sciences and Research, 2011, 2, 1356; (b) J. K. Malik, F. V. Manvi, B. K. Nanjwade, S. Singh, and P. Purohit, Der Pharmacia Letter, 2010, 2, 347; (c) L. Racané, H. Čičak, Z. Mihalić, G. Karminski-Zamola, and V. Tralić-Kulenović, Tetrahedron, 2011, 67, 2760. CrossRef
3. (a) A. Naya, K. Kobayashi, M. Ishikawa, K. Ohwaki, T. Saeki, K. Noguchi, and N. Ohtake, Bioorg. Med. Chem. Lett., 2001, 11, 1219; CrossRef (b) M. N. Greco, W. E. Hageman, E. T. Powell, J. J. Tighe, and F. J. Persico, J. Med. Chem., 1992, 35, 3180; CrossRef (c) A. N. Choudhary, A. Kumar, and V. Juar, Mini-Rev. Med. Chem., 2010, 10, 705; CrossRef (d) S. Massari, D. Daelemans, M. L. Barreca, A. Knezevich, S. Sabatini, V. Cecchetti, A. Marcello, C. Pannecouque, and O. Tabarrini, J. Med. Chem., 2010, 53, 641; CrossRef (e) R. A. Glennon, J. J. Gaines, and M. E. Rogers, J. Med. Chem., 1981, 24, 766; CrossRef (f) M. S. Gomaa, J. L. Armstrong, B. Bobillon, G. J. Veal, A. Brancale, C. P. F. Redfern, and C. Simons, Bioorg. Med. Chem., 2008, 16, 8301; CrossRef (g) J. Van Heusden, R. Van Gineckel, H. Bruwiere, P. Moalans, B. Janssen, W. Floren, B. J. van der Leede, G. Sanz, M. Venet, L. Dillen, C. Van Hove, G. Willemsens, M. Janicot, and W. Wouters, Br. J. Cancer, 2002, 86, 605; CrossRef (h) M. S. Gomaa, J. L. Armstrong, B. Bobillon, G. J. Veal, A. Brancale, C. P. F. Redfern, and C. Simons, Bioorg. Med. Chem., 2008, 16, 8301; CrossRef (i) C. Liu, J. Lin, S. Pitt, R. F. Zhang, J. S. Sack, S. E. Kiefer, K. Kish, A. M. Doweyko, H. Zhang, P. H. Marahe, J. Trzaskos, M. Mckinnon, J. H. Dodd, J. C. Barrish, G. L. Schieven, and K. Letheris, Bioorg. Med. Chem., 2008, 16, 1874; CrossRef (j) E. Song, N. Kaur, N. Y. Park, Y. Jin, K. Lee, G. Kim, K. Y. Lee, J. S. Yang, J. H. Shin, K. Y. Nam, K. T. No, and G. Han, Eur. J. Med. Chem., 2008, 43, 1519; CrossRef (k) P. L. Sangwan, J. L. Koul, S. Koul, M. V. Reddy, N. Thota, J. A. Khan, A. Kumar, N. P. Kallia, and G. N. Qazi, Bioorg. Med. Chem., 2008, 16, 9847. CrossRef
4. Eq. 1: (a) A. K. Chakraborti, S. Rudrawar, G. Kaur, and L. Sharma, Synlett, 2004, 1533; CrossRef (b) M. Kodomari, Y. Tamaru, and T. Aoyama, Synth. Commun., 2004, 34, 3029; CrossRef (c) J. F. Mike, J. J. Inteman, A. Ellern, and M. Jeffries-EL, J. Org. Chem., 2010, 75, 495; CrossRef (d) C. Zhu and T. Akiyama, Synlett, 2010, 2457; CrossRef (e) D. Azarifar, K. Khosravi, Z. Najminejad, and K. Soleimani, Heterocycles, 2010, 81, 2855; CrossRef (f) X. Fan, Y. He, Y. Wang, Z. Xue, X. Zhang, and J. Wang, Tetrahedron Lett., 2011, 52, 899; CrossRef (g) Y. Riadi, R. Mamouni, R. Azzalou, M. El Haddad, S. Roitier, G. Guillaumet, and S. Lazar, Tetrahedron Lett., 2011, 52, 3492. CrossRef
5. Eq. 2: J. Hyvl and J. Srogl, Eur. J. Org. Chem., 2010, 2849. CrossRef
6. Eq. 3: (a) Q. Ding, X. He, and J. Wu, J. Comb. Chem., 2009, 11, 587; CrossRef (b) Y.-J.Guo, R.-Y. Tang, P. Zhong, and J.-H. Li, Tetrahedron Lett., 2010, 51, 649; CrossRef (c) W. Wang, W. Zhong, R. Zhou, J. Yu, J. Dai, Q. Ding, and Y. Peng, Heterocycles, 2010, 81, 2841. CrossRef
7. Eq. 4: (a) C. Bendí, F. Bravo, P. Uriz, E. Fernández, C. Claver, and S. Castillón, Tetrahedron Lett., 2003, 44, 6073; CrossRef (b) L. L. Joyce, G. Evindar, and R. A. Batey, Chem. Commun., 2004, 446; CrossRef (c) G. Evindar and R. Batey, J. Org. Chem., 2006, 71, 1802; CrossRef (d) J. Wang, F. Peng, J. Jiang, Z. Lu, L. Wang, J. Bai, and Y. Pan, Tetrahedron Lett., 2008, 49, 467; CrossRef (e) E. A. Jaseer, D. J. C. Prasad, A. Dandapat, and G. Sekar, Tetrahedron Lett., 2010, 51, 5009; CrossRef (f) E. Feng, H. Huang, Y. Zhou, D. Ye, H. Jiang, and H. Liu, J. Comb. Chem., 2010, 12, 422; CrossRef (g) D. Ma, X. Lu, L. Shi, H. Zhang, Y. Jiang, and X. Liu, Angew. Chem. Int. Ed., 2011, 50, 1118. CrossRef
8. Eq. 5: S. Murru, P. Mondal, R. Yella, and B. Patel, Eur. J. Org. Chem., 2009, 5406. CrossRef
9. Eq. 6: C. Li, X. Zhang, R. Tang, P. Zhong, and J. Li, J. Org. Chem., 2010, 75, 7037. CrossRef
10. Eq. 7: J. Zhu, Z. Chen, H. Xie, S. Li, and Y. Wu, Org. Lett., 2010, 12, 2434. CrossRef
11. Eq. 8: (a) D. S. Bose and M. Idrees, J. Org. Chem., 2006, 71, 8261; CrossRef (b) N. K. Downer-Riley and Y. A. Jackson, Tetrahedron, 2008, 64, 7741; CrossRef (c) R. Yella, S. Murru, A. R. Ali, and B. Patel, Org. Bio. Chem., 2010, 8, 3389; CrossRef (d) W. Lo, W. Hu, H. Lo, C. Chen, C. Kao, J. K. Vandavasi, and J. Wang, Org. Lett., 2010, 12, 5570. CrossRef
12. Reviews: (a) H. Sashida, Rev. Heteroatom Chem., 2000, 22, 59; (b) H. Sashida, J. Syn. Org. Chem. Jpn., 2001, 59, 355; CrossRef (c) H. Sashida, Mini-Rev. in Org. Chem., 2007, 4, 105; CrossRef (d) H. Sashida and M. Minoura, J. Syn. Org. Chem. Jpn., 2009, 67, 714; CrossRef (e) H. Sashida, Heterocycles, 2011, 83, 2223. CrossRef
13. Recent works: (a) H. Sashida, A. Nakayama, and M. Kaname, Synthesis, 2008, 3229; CrossRef (b) H. Sashida, S. Nakabayashi, M. Kaname, and M. Minoura, Heterocycles, 2010, 80, 1339; CrossRef (c) H. Sashida, H. Satoh, K. Ohyanagi, and M. Kaname, Molecules, 2010, 15, 1466; CrossRef (d) H. Sashida, M. Kaname, and K. Ohyanagi, Heterocycles, 2010, 82, 441; CrossRef (e) H. Sashida, M. Kaname, A. Nakayama, H. Suzuki, and M. Minoura, Tetrahedron, 2010, 66, 5149; CrossRef (f) H. Sashida, S. Nakabayashi, H. Suzuki, M. Kaname, and M. Minoura, Tetrahedron Lett., 2010, 51, 5395; CrossRef (g) M. Kaname, M. Minoura, and H. Sashida, Tetrahedron Lett., 2011, 52, 505. CrossRef
14. Y. Xie, F. Zhang, J. Li, and X. Shi, Synlett, 2010, 901. CrossRef
15. M. D. Charles, P. Schults, and S. Buchward, Org. Lett., 2005, 7, 3965. CrossRef
16. L. L. Joyce and R. A. Batey, Org. Lett., 2009, 11, 2792. CrossRef
17. P. Saha, T. Ramana, N. Purkait, M.-A. Ali, R. Paul, and T. Punniyamurthy, J. Org. Chem., 2009, 74, 8719. CrossRef
18. R. Cano, D. J. Ramón, and M. Yus, J. Org. Chem., 2011, 76, 654. CrossRef
19. G. Shen, X. Lv, and W. Bao, Eur. J. Org. Chem., 2009, 5897. CrossRef
20. (a) M. W. Hooper, M. Utsunomiya, and J. F. Hartwig, J. Am. Chem. Soc., 1959, 81, 5957; CrossRef (b) J. J. D’Amico, C. C. Tung, and L. A. Walker, J. Org. Chem., 2003, 68, 2861. CrossRef