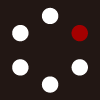
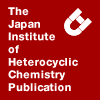
HETEROCYCLES
An International Journal for Reviews and Communications in Heterocyclic ChemistryWeb Edition ISSN: 1881-0942
Published online by The Japan Institute of Heterocyclic Chemistry
e-Journal
Full Text HTML
Received, 5th August, 2011, Accepted, 1st September, 2011, Published online, 8th September, 2011.
DOI: 10.3987/COM-11-S(P)95
■ Convergent Total Syntheses of the Pentacyclic Lamellarins K, T, U and W via the Addition of Azomethine Ylides to Tethered Tolans
Bernard L. Flynn and Martin G. Banwell*
Research School of Chemistry, Institute of Advanced Studies, The Australian National University, Canberra, ACT 0200, Australia
Abstract
The title compounds, 1–4 respectively, have been prepared in a concise and fully regiocontrolled manner via the addition of an azomethine ylide to an ester-linked tolan. The resulting annulated dihydropyrrole was oxidized to the corresponding fully aromatic system and the associated isopropyl ethers then selectively cleaved with aluminium trichloride to reveal the free phenolic hydroxyl groups associated with the target compounds.INTRODUCTION
The large family of pentacyclic lamellarins, of which the title compounds 1–4 are representative members, only vary in the degree, position and nature (hydroxy vs. methoxy and 5,6-single bond vs. 5,6-double bond) of the oxygenation pattern around their aromatic perimeters.1 They have been isolated from diverse sources of marine organisms including molluscs, ascidians and sponges but are most likely the products of bacterial symbionts associated with such species.2 The lamellarins have been subjected to a wide range of biological assays and these have revealed that they possess a remarkable array of useful properties, many of which have been highlighted in recent reviews.1 Lamellarin D, for example, the C8-hydroxy analogue of compound 3, is a potent inhibitor of topoisomerase I but exerts its impressive cytotoxicity primarily by inducing mitochondrial apoptosis independently of nuclear signaling.1a This compound is currently in preclinical development as an anti-cancer drug. Given all of this, it is unsurprising that considerable effort has been devoted to the synthesis of the lamellarins and a wide-range of approaches have been described.1 One of the earliest of these was reported by us in communication form3 in 1997 and involved, as a pivotal step, the addition of an azomethine ylide to a tolan that are tethered to one another through an ester linkage. This very efficient process allowed for the rapid assembly of the pentatcyclic framework, 5, of the target compounds and enabled us to prepare multigram quantities of lamellarin K (1).4 Others have since exploited this approach to prepare a wide range of lamellarin analogues for biological evaluation.5 Herein we now provide full details of our original work as well as extensions of it to the total synthesis of lamellarins T (2),6 U (3)6 and W (4).6 The preparation of the (thus far) non-natural system 6 (7-deoxylamellarin K) is also described.
RESULTS AND DISCUSSION
Our initial investigations3 were focused on establishing the basic framework, 5, of the title natural products and the ultimately successful route is shown in Scheme 1.
Thus, phenylacetylene (7) was subjected Sonogashira cross-coupling7 with the acetate derivative, 8, of o-iodophenol. The resulting tolan derivative 9 (quant.)8 was hydrolyzed using potassium carbonate in methanol and the o-hydroxytolan (10)8 so-formed was re-esterified using α-bromoacetic acid in dichloromethane (DCM) in the presence of 4-(N,N-dimethylamino)pyridine (DMAP) and dicyclohexylcarbodiimide (DCC). The ensuing ester 11 (91% from 9) was treated with isoquinoline and the quinolinium salt 12 thus generated was immediately treated with triethylamine so as to form the corresponding azomethine ylide. This could not be isolated because it engaged in a seemingly spontaneous [3 + 2] cycloaddition reaction9 to give a mixture of dihydropyrroles. Accordingly, the crude reaction mixture was treated with 2,3-dichloro-5,6-dicyano-1,4-benzoquinone (DDQ) so as to effect oxidation of these dihydro-species to the fully aromatic system 5 and, after silica gel chromatography, this was obtained in 92% yield (from precursor 11). All the spectral data acquired on this compound were in complete accord with the assigned structure but final confirmation of this came from a single-crystal X-ray analysis, details of which have been reported previously.2
As a necessary prelude to extending the chemistry described immediately above to the synthesis of lamellarin K (1) several “building blocks” had to be prepared. In the construction of each of these the free hydroxyl groups associated with the target compound were carried through the reactions sequences as isopropyl ethers on the basis that these could be cleaved selectively in the presence of the corresponding methyl ethers using aluminium trichloride or boron trichloride.10 The first of the building blocks to be prepared was that corresponding to the isolated F-ring of target 1 as well as C-1 and C-2 of the central pyrrole ring. Thus, vanillin (13) (Scheme 2) was protected as the corresponding isopropyl ether 141c by treating the former compound with a mixture of isopropyl bromide and potassium carbonate in DMF at 80 °C for 15 h. Ether 14 (quant.) so obtained was subjected to a Corey–Fuchs olefination reaction11 using carbon tetrabromide in the presence of triphenylphosphine and zinc metal and thereby forming the β,β-dibromostyrene 15 (99%) which would serve (see below) as a precursor to a metal acetylide that engaged in a Sonogashira-type cross-coupling reaction with an aryl iodide so as to generate the relevant tolan derivative.
The aryl iodide required for the abovementioned cross-coupling reaction, and which would become the A-ring of target 1, was prepared by first converting isovanillin (16) (Scheme 3) into the corresponding isopropyl ether using essentially the same conditions as employed for the conversion 13 → 14. Compound 1712 so formed (in quantitative yield) was then subject to electrophilic aromatic iodination using molecular iodine in the presence of silver trifluoroacetate and thus producing the target iodide 185b,13 in 93% yield. The 1,2,4,5-substitution pattern about the aromatic ring of this product was evident from the 1H NMR spectrum that displayed two one-proton singlets at δ 7.34 and 7.24 and which arise from the 3,6-related aromatic protons.
The third and final building block required for the assembly of lamellarin K was that corresponding to the D- and E-rings of the target. This was prepared by the route shown in Scheme 4 and involved initial conversion of readily available 2-hydroxy-3,4-dimethoxybenzaldehyde (19)14 into the corresponding isopropyl ether 20 (96%) under the same conditions as used earlier. Compound 20 was subjected to a Henry reaction15 with nitromethane using ammonium acetate as base. The β-nitrostyrene 21 so formed (in 98% yield) was treated with lithium aluminium hydride in diethyl ether and thereby producing the β-phenethylamine 22 (75%) that was subjected to a Pictet–Spengler16 reaction using paraformaldehyde and formic acid. By such means the tetrahydroisoquinoline 23 was obtained in 91% yield. Selective oxidation of compound 23 to its 1,2-dehydro-congener 24 was readily effected (in 94% yield) by exposing the former compound to Fremy’s salt in the presence of sodium carbonate.17
With compounds 15, 18 and 24 to hand the assembly of lamellarin K from these via the approach shown in Scheme 1 could be pursued. To such ends the first of these compounds, viz. β,β-dibromostyrene 15, was treated with butyllithium (to effect a Fritsch–Buttenberg–Wiechell rearrangement)18 and the ensuing lithium acetylide was trans-metallated using anhydrous zinc chloride to generate the corresponding zincated species 25 (Scheme 5). Compound 25 was not isolated but subjected to in situ cross-coupling with aryl iodide 18 and using Pd(PPh3)4 as catalyst. In this way the o-formylated tolan 26 was obtained in 84% yield. Upon exposure to m-chloroperbenzoic acid (m-CPBA) in the presence of potassium bicarbonate compound 26 underwent a Dakin oxidation19 to give phenol 27 (92%) that was immediately esterified with α-iodoacetic acid in the presence of DMAP and DCC. Ester 28 (97%) so-formed was treated with the 3,4-dihydroisoquinoline 24 and the isoquinolinium salt 29 thus obtained was treated, in refluxing 1,2-dichloroethane, with Hünig’s base. Interestingly, under these conditions the fully aromatic triisopropyl ether, 30, of lamellarin K was obtained in 81% yield. Presumably the aromatization process involved in this conversion is effected via the combination of iodide ion and molecular oxygen present in the reaction mixture. The completion of the synthesis of lamellarin K (1)20 was readily achieved by reacting compound 30 with ca. three molar equivalents of aluminium trichloride in DCM at ambient temperatures. In this way compound 1 was obtained in 95% yield as a white, crystalline solid. The spectroscopic data derived from this material were in complete accord with the assigned structure and compared favorably those reported4 for the natural product (Table 1).
A synthesis of lamellarin T (2)21 using the same chemistry and related building blocks is shown in Scheme 6. Thus, the previously prepared isopropyl ether 17 was engaged in a Corey–Fuchs olefination reaction and the ensuing β,β-dibromostyrene 3113 (99%) subjected to sequential reaction with butyllithium then zinc chloride. Acetylide 32 so-formed was cross-coupled with iodide 18 and thereby generating tolan 335b,13 in 92% yield. Dakin oxidation of compound 33 and in situ hydrolysis of the ensuing formate ester5b then afforded phenol 345b,13 (93%) that was esterified with α-iodoacetic acid in the presence of DMAP and DCC to give compound 355b (94%). Reaction of ester 35 with the readily available 3,4-dihydroisoquinoline 3622 followed by treatment of the ensuing salt 37 with Hünig’s base in refluxing 1,2-dichloroethane afforded the diisopropyl ether, 38, of lamellarin T in 71% yield. Cleavage of these ether residues within compound 38 using AlCl3 in DCM then gave lamellarin T (2) itself in 90% yield.
Once again, the derived spectroscopic data were in complete accord with the assigned structure and matched those reported for earlier by Ruchirawat20b for synthetically-derived material (Table 2). Since the 13C NMR spectrum of naturally-derived lamellarin T has not been reported appropriate comparisons could not be made. However, the comparison of the corresponding 1H NMR spectral data was possible6 and the levels of agreement were very good.
For the purposes of obtaining material for biological screening, the diacetate derivative, 39, of lamellarin T was prepared in 83% yield by reacting the latter compound with acetic anhydride in the presence of DMAP and pyridine (Scheme 6).
The tolan-based ester 35 proved to be an effective precursor to lamellarin U (3).23 Thus, as shown in Scheme 7, reaction of compound 35 with 6,7-dimethoxy-3,4-dihydroisoquinoline (40)24 in 1,2-dichloroethane at room temperature and then Hünig’s base at reflux afforded the diisopropyl ether, 41,5b of compound 3 in 70% yield. Once again, treatment of this last compound with AlCl3 in DCM resulted in selective cleavage of the more substituted ether residues and thereby generating lamellarin U (3) in 94% yield. The derived spectroscopic data were in complete accord with the assigned structure and matched those reported earlier by Ruchirawat20b for synthetically-derived material (Table 3). As was the case with congener 2 (lamellarin T), the 13C NMR spectrum of naturally-derived lamellarin U (3) hasn’t been reported so appropriate comparisons with our synthetic material could not be made. However, a comparison of the corresponding 1H NMR data sets was possible6 and the levels of agreement were very good.
Since lamellarin W (4)25 is the 5,6-dehydro-analogue of lamallerin T (2) we sought to effect the necessary oxidation of the diisopropyl ether, 38, of the latter compound (Scheme 8). In the event, treatment of compound 38 with DDQ in refluxing CHCl3 afforded the corresponding fully aromatic system 42 in essentially quantitative yield.26 Deprotection of compound 42 in the usual manner then gave the natural product 4 in 94% yield and, once again, all the derived spectral data were in accord with the assigned structure and in relatively good agreement with those reported6 for the natural product. It seems reasonable to attribute the minor discrepancies between the two data sets shown in Table 4 to the different solvent systems used in acquiring them [CDCl3/(CD3)2SO mixtures vs. (CD3)2SO alone].
Unsurprisingly, the tolan ester 28 that had served as a precursor to lamellarin K also proved to be a useful synthon in the preparation of related systems. As part of a proposed development of a structure-activity relationship (SAR) profile for the pentacyclic lamellarins, we sought to make 7-deoxylamellarin K (6) and were able to do so using compound 28 as shown in Scheme 9. Thus, reaction of this α-iodoacetate with the 1,2-dihydroisoquinoline 40 used earlier in the synthesis of lamellarin U and treatment of the ensuing salt with Hünig’s base in refluxing 1,2-dichloroethane afforded the diisopropyl ether, 43, of the final target in 79% yield. Exposure of this last compound to aluminium trichloride in DCM at 18 °C for 0.5 h then gave compound 6 as a white, crystalline solid in 89% yield. The spectral data derived from this material, which are presented in the Experimental section, were in complete accord with the assigned structure.
CONCLUSIONS
The ready assembly of the pentacyclic lamellarins K, T, U and W by the methods detailed above serves to highlight the utility of this highly modular approach to such natural products. Indeed, its exploitation by others5 during the course of developing SAR profiles for the lamellarins suggests the title process will continue serve as a useful tool for the development of analogues with therapeutic potential. It may also prove useful for the assembly of lamellarins that incorporate unusual isotopic labeling patterns.
EXPERIMENTAL
General Protocols
Proton (1H) and carbon (13C) NMR spectra were recorded on a Varian Gemini 300 NMR spectrometer operating at 300 MHz (for proton) and 75 MHz (for carbon). Unless otherwise specified, spectra were acquired at 20 °C in deuterochloroform (CDCl3) that had been filtered through basic alumina immediately prior to use. Chemical shifts are recorded as δ values in parts per million (ppm). Infrared spectra (νmax) were recorded on a Perkin–Elmer 1800 Series FTIR Spectrometer and samples were analyzed as thin films on KBr plates. Low resolution mass spectra were recorded on a Micromass–Waters LC-ZMD single quadrupole liquid chromatograph-MS or VG Quattro II triple quadrupole MS instrument using electron impact techniques. High resolution mass spectra were recorded on an AUTOSPEC spectrometer. Dichloromethane (DCM) was distilled from calcium hydride and THF was distilled, under nitrogen, from sodium benzophenone ketyl. Where necessary, reactions were performed under a nitrogen atmosphere. Flash chromatographic separations were carried out using protocols defined by Still et al.27
Specific Transformations
Compound 9. Pd(PPh3)4 (132 mg 0.11 mmol), phenylacetylene (7) (1.30 mL, 1.20 g, 12.6 mmol) and CuI (44 mg, 0.23 mmol) were added, sequentially, to a magnetically stirred solution of 2-acetoxyiodobenzene (8) (3.00 g, 11.45 mmol) in Et3N (20.0 mL) and the ensuing mixture stirred at 18 °C for 4 h. After this time the reaction mixture was concentrated under reduced pressure and the residue thus obtained dissolved in DCM (50 mL) and the resulting solution washed with HCl (1 × 50 mL of a 0.5 M aqueous solution) and brine (1 50 mL) before being dried (MgSO4), filtered and concentrated onto TLC-grade silica gel (8.0 g). The free-flowing solid thus obtained was subjected to flash chromatography (silica gel; 3:1, 2:1, 1:1 then 1:2 v/v hexane/DCM gradient elution) and concentration of the appropriate fractions gave the tolan 98 (2.70 g, 100%). The physical and spectroscopic data acquired on this material were in complete accord with those recorded8 previously.
Compound 11. Compound 9 (2.24 g, 9.50 mmol) was added to a magnetically stirred slurry of anhydrous K2CO3 (2.0 g, mmol) in MeOH (20 mL). After 0.25 h the reaction mixture was diluted with HCl (50 mL of a 0.5 M aqueous solution) and extracted with DCM (2 40 mL). The combined extracts were dried (MgSO4) filtered and then concentrated under reduced pressure. The crude sample of o-hydroxytolan (10)8 thus obtained was dissolved in DCM (20 mL) and the resulting solution treated with α-bromoacetic acid (1.32 g, 9.5 mmol) and DMAP (58 mg, 0.48 mmol). DCC (2.07 g, 10 mmol) was then added in portions and the ensuing mixture stirred at 18 °C for 3 h before being filtered through Celite™ and the filtrate concentrated onto TLC-grade silica gel (8 g). The free-flowing solid thus obtained was subjected to flash chromatography (silica gel; 2:1, 1:1 then 1:2 v/v hexane/DCM gradient elution) and concentration of the appropriate fractions (Rf = 0.5 in 2:1 hexane/DCM) gave compound 11 (2.73 g, 91%) as an amber-coloured oil (Anal. Calcd for C16H11BrO2: C, 60.98; H, 3.52; Br, 25.35. Found: C, 61.26; H, 3.45; Br, 25.06%). 1H NMR (300 MHz, CDCl3) δ 7.61 (dd, J = 7.5 and 1.5 Hz, 1H), 7.54–7.52 (complex m, 2H), 7.41–7.35 (complex m, 4H), 7.29 (m, 1H), 7.17 (dd, J = 8.1 and 1.4 Hz, 1H), 4.13 (s, 2H); 13C NMR (75.5 MHz, CDCl3) δ 165.0 (C), 150.6 (C), 133.0 (CH), 131.4 (CH), 129.3 (CH), 128.5 (CH), 128.2 (CH), 126.3 (CH), 122.4 (C), 121.7 (CH), 116.9 (C), 94.6 (C), 83.5 (C), 25.1 (CH2); νmax (KBr) 3060, 2221, 1761, 1596, 1571, 1496, 1444, 1258, 1195, 1116, 1099 756, 691 cm–1; MS (70 eV) m/z 316 and 314 (30) (M+•, 31 and 30%, respectively), 277 (15), 221 (24), 193 (100), 165 (42).
Compound 5. Isoquinoline (98 µL, 0.83 mmol) was added to a magnetically stirred solution of compound 11 (262 mg, 0.83 mmol) in THF (5 mL) and the ensuing mixture allowed to stir at 18 °C for 6 h before being treated with Et3N (215 µL, 0.83 mmol) and CHCl3 (10 mL). The bright-orange solution thus formed, and presumed to contain the isoquinolinium salt 12 and/or the derived azomethine ylide, was heated at reflux for 4 h then the by now light-yellow solution was cooled and concentrated under reduced pressure. The resulting light-yellow oil was dissolved in DCM (7 mL) and DDQ (189 mg, 0.83 mmol) then added. The ensuing mixture was immediately concentrated onto TLC-grade silica gel (2 g) and the resulting free-flowing solid subjected to flash chromatography (silica gel; 2:1, 1:1 then 1:2 v/v hexane/DCM gradient elution). Concentration of the appropriate fractions (Rf = 0.5 in 1:2 hexane/DCM) gave compound 5 (277 mg, 92%) as white, crystalline masses, mp 313–315 °C (Found M+•, 361.1101. C25H15NO2 requires M+•, 361.1103). 1H NMR (300 MHz, CDCl3) δ 9.29 (d, J = 7.2 Hz, 1H), 7.67–7.60 (complex m, 4H), 7.56–7.39 (complex m, 5H), 7.32 (dt, J = 7.5 and 1.8 Hz, 1H), 7.25 (dt, J = 7.5 and 1.5 Hz, 1H), 7.12–7.05 (complex m, 2H), 6.99 (dt, J = 7.5 and 1.5 Hz, 1H); 13C NMR (75.5 MHz, CDCl3) δ 155.2 (C), 151.7 (C), 135.6 (C), 134.1 (C), 131.0 (CH), 129.9 (CH), 129.6 (C), 128.7 (CH), 128.6 (C), 128.4 (CH), 128.1 (CH), 127.5 (CH), 127.3 (CH), 125.0 (C), 124.4 (CH), 124.1 (CH), 123.9 (CH), 117.9 (C), 117.3 (CH), 114.3 (C), 113.4 (CH), 109.3 (CH) (one signal obscured or overlapping); νmax (KBr) 3047, 1705, 1470, 1445, 1411, 1369, 1312, 1179, 1110, 1049, 790, 741 cm–1; MS (70 eV) m/z 361 (M+•, 100%).
Compound 14. Isopropyl bromide (40.0 mL, 421 mmol) was added to a magnetically stirred suspension of anhydrous K2CO3 (48.0 g, 348 mmol) and vanillin (13) (40.0 g, 263 mmol) in DMF (150 mL) and the resulting slurry heated to 80 °C for 15 h. The reaction mixture was then cooled to 18 °C, diluted with Et2O (200 mL) and washed with water (4 200 mL) before being dried (MgSO4), filtered and concentrated under reduced pressure to give compound 141c (51.0 g, 100%) as a tan-coloured oil. The spectroscopic data acquired on this material were in complete accord with those recorded1c previously.
This material was used without purification in the next step of the reaction sequence.
Compound 15. Carbon tetrabromide (51.3 g, 154.7 mmol) was added, in portions, to a magnetically stirred mixture of zinc dust (10.1 g, 154.5 g.atom) and PPh3 (40.5 g, 159.4 mmol) in DCM (350 mL) maintained at 0 °C on an ice-salt bath. The resulting suspension was allowed to warm to 18 °C and then stirred at this temperature for a further 22 h before being re-cooled to 0 °C then treated, over 0.03 h, with compound 14 (15.0 g, 77.3 mmol). The resulting mixture was allowed to stir at 18 °C for 1 h then diluted with hexane (200 mL) and filtered through a sintered glass funnel and the filtrate concentrated onto TLC-grade silica gel (30.0 g). The resulting free-flowing solid was subjected to flash chromatography (silica gel; 2:1, 1:1 then 1:2 v/v hexane/DCM gradient elution). Concentration of the appropriate fractions (Rf = 0.5 in 1:1 v/v hexane/DCM) then afforded compound 15 (27.2 g, 99%) as white, crystalline masses, mp 36–38 °C (Anal. Calcd for C12H14Br2O2: C, 41.17; H, 4.03; Br, 45.65. Found: C, 41.21; H, 3.92; Br, 45.47%). 1H NMR (300 MHz, CDCl3) δ 7.41 (s, 1H), 7.21 (d, J = 2.1 Hz, 1H), 7.07 (dd, J = 8.4 and 2.1 Hz, 1H), 6.87 (d, J = 8.4 Hz, 1H), 4.58 (septet, J = 6.0 Hz, 1H), 3.87 (s, 3H), 1.39 (d, J = 6.0 Hz, 6H); 13C NMR (75.5 MHz, CDCl3) δ 149.7 (C), 147.7 (C), 136.5 (CH), 127.9 (C), 121.9 (CH), 114.4 (CH), 111.8 (CH), 87.1 (C), 71.1 (CH), 56.0 (CH3), 22.1 (CH3); νmax (KBr) 2976, 2933, 1599, 1510, 1464, 1418, 1383, 1373, 1267, 1235, 1141, 1110, 1036, 953, 872, 839, 816, 632 cm–1; MS (70 eV) m/z 352, 350 and 348 (M+•, 28, 49 and 31%, respectively), 310, 308 and 306 (51, 100 and 54, respectively), 295 (34), 293 (38), 291 (36).
Compound 17. Isopropyl bromide (19.0 mL, 200 mmol) was added to a magnetically suspension of anhydrous K2CO3 (42.0 g, 302 mmol) and isovanillin (16) (23.0 g, 151.3 mmol) in DMF (100 mL). The ensuing slurry was heated at 80 °C for 13 h then cooled to 18 °C before being diluted with Et2O (200 mL) then washed with water (4 200 mL). The separated organic phase was dried (MgSO4), filtered and concentrated under reduced pressure to give the diether 1712 (29.3 g, 100%). The spectroscopic data acquired on this material were in complete accord with those recorded12 previously.
This material was used without purification in the next step of the reaction sequence.
Compound 18. Compound 18 was prepared by electrophilic iodination of aldehyde 17 using a procedure defined earlier.13 The physical and spectroscopic data acquired on this material were in complete accord with those recorded5b,13 previously.
Compound 19. AlCl3 (14.0 g, 10.5 mmol) was added, in portions over 0.5 h, to a rapidly stirred solution of 2,3,5-trimethoxybenzaldehyde (20.0 g, 10.2 mmol) in anhydrous benzene (150 mL) and the resulting mixture heated at reflux for 5 h then the cooled reaction mixture concentrated under reduced pressure. The ensuing residue was dissolved in DCM (200 mL) and the resulting solution washed with HC1 (2 80 mL of a 5 M aqueous solution) then the organic layer was extracted with NaOH (2 80 mL of 10% w/v aqueous solution). The combined aqueous extracts were cooled to 0 °C and acidified to pH = 2 by the dropwise addition of HCl (32% by weight in water). The resulting slurry was extracted with DCM (2 100 mL) and the combined organic fractions were dried (MgSO4), filtered and concentrated under reduced pressure. The solid residue thus obtained was suspended in hexane (120 mL) and the ensuing mixture stirred vigorously at 18 °C for 24 h then stored at 0 °C for 6 h before being filtered to give 2-hydroxy-3,4-dimethoxybenzaldehyde (19)14 (18.3 g, 99%). The physical and spectroscopic data acquired on this material were in complete accord with those recorded14 previously.
This material was used without purification in the next step of the reaction sequence.
Compound 20. Isopropyl bromide (11.5 mL, 121.9 mmol) was added to a magnetically stirred suspension of anhydrous K2CO3 (16.9 g, 122.5 mmol) and 2-hydroxy-3,4-dimethoxybenzaldehyde (19) (17.0 g, 93.8 mmol) in DMF (100 mL). The resulting mixture was heated at 80 °C for 15 h then cooled to 18 °C, diluted with Et2O (200 mL) and washed with water (4 200 mL) before being dried (MgSO4), filtered and concentrated under reduced pressure to give compound 20 (20.0 g, 96%) as a clear, colourless oil (Anal. Calcd for C12H16O4: C, 64.27; H, 7.19. Found: C, 64.05; H, 7.18%). 1H NMR (300 MHz, CDC13) δ 10.26 (s, 1H), 7.61 (d, J = 9.0 Hz, 1H), 6.74 (d, J = 9.0 Hz, 1H), 4.71 (septet, J = 6.3 Hz, 1H), 3.92 (s, 3H), 3.86 (s, 3H), 1.32 (d, J = 6.3 Hz, 6H); 13C NMR (75.5 MHz, CDC13) δ 189.0 (CH), 159.0 (C), 154.6 (C), 141.7 (C), 124.4 (C) 123.5 (CH), 107.1 (CH), 76.5 (CH), 60.3 (CH3), 55.9 (CH3), 22.1 (CH3); νmax (KBr) 2976, 2937, 2843, 1680, 1587, 1493, 1455, 1427, 1382, 1289, 1261, 1229, 1198, 1092 cm–1; MS (70 eV) m/z 224 (M+•, 67%), 182 (100), 167 (60), 139 (72).
Compound 21. Nitromethane (8.0 mL, 146.5 mmol) and NH4OAc (4.0 g, 51.4 mmol) were added to a solution of aldehyde 20 (14.5 g, 64.7 mmol) in toluene (150 mL) contained in an apparatus fitted with a condenser and Dean–Stark trap. The reaction mixture was heated at reflux for 5 h then cooled and concentrated under reduced pressure. The residue thus obtained was subjected to flash chromatography (silica gel; 2:1, 1:1 then 1:2 v/v hexane/DCM gradient elution) and concentration of the appropriate fractions (Rf = 0.5 in 1:1 v/v hexane/DCM) then gave β-nitrostyrene 21 (17.0 g, 98%) as a white, crystalline solid, mp 63–65 °C (Anal. Calcd for C11H17IO3: C, 58.42; H, 6.41; N, 5.24. Found: C, 58.39; H, 6.43; N, 5.16%). 1H NMR (300 MHz, CDC13) δ 8.12 (d, J = 13.5 Hz, 1H), 7.72 (d, J = 13.5 Hz, 1H), 7.19 (d, J = 9.0 Hz, 1H), 6.69 (d, J = 9.0 Hz, 1H) 4.71 (septet, J = 6.3 Hz, 1H), 3.88 (s, 3H), 3.80 (s, 3H), 1.27 (d, J = 6.3 Hz, 6H); 13C NMR (75.5 MHz, CDC13) δ 157.1 (C), 152.0 (C), 142.4 (C), 135.9 (CH), 135.5 (CH), 125.5 (CH), 118.0 (C), 107.4 (CH), 76.5 (CH), 60.3 (CH3), 55.9 (CH3), 22.4 (CH3); νmax (KBr) 3108, 2976, 2935, 1626, 1598, 1495, 1465, 1451, 1440, 1384, 1337, 1274, 1245, 1222, 1172, 1096, 1034, 986, 965, 796 cm–1; MS (70 eV) m/z 267 (M+•, 54%), 225 (32), 178 (100), 163 (66).
Compound 22. A solution of β-nitrostyrene 21 (4.00 g, 14.96 mmol) in Et2O/THF (4.0 mL of a 3:1 v/v mixture) was added dropwise, over a period of 0.66 h, to a magnetically stirred suspension of LiAlH4 (2.27g, 59.81 mmol) in dry Et2O (200 mL) (CAUTION: the reaction is strongly exothermic and causes reflux). After the addition was complete and the reaction mixture had been cooled to 18 °C it was allowed to stir at this temperature for 1 h before being further cooled to 0 °C and treated, dropwise over 1.5 h, with H2SO4 (60 mL of a 1.5 M aqueous solution). The two phases were separated and the aqueous one extracted with Et2O (2 100 mL). The aqueous layer was cooled to 0 °C and treated with KOH (1 M aqueous solution) until pH = 9 was attained. The resulting mixture was extracted with Et2O (2 100 mL) and the combined organic phases were dried (MgSO4), filtered and concentrated under reduced pressure to give amine 22 (2.70 g, 75%) as an amber-coloured oil (Anal. Calcd for C13H21NO3: C, 65.25; H, 8.84; N, 5.85. Found: C, 65.13; H, 8.76; N, 5.59%). 1H NMR (300 MHz, CDCl3) δ 6.67 (d, J = 8.4 Hz, 1H), 6.43 (d, J = 8.4 Hz, 1H) 4.46 (septet, J = 6.3 Hz, 1H), 3.67 (s, 3H), 3.66 (s, 3H), 2.74 (t, J = 7.2 Hz, 2H), 2.56 (t, J = 7.2 Hz, 2H), 1.11 (d, J = 6.3 Hz, 6H) (signals due to NH2 group protons not observed); 13C NMR (75.5 MHz, CDCl3) δ 151.7 (C), 149.4 (C), 141.9 (C), 126.1 (C), 123.7 (CH), 106.3 (CH), 74.4 (CH), 59.9 (CH3), 55.5 (CH3), 42.4 (CH2), 33.9 (CH2), 22.3 (CH3); νmax (KBr) 2974, 2935, 2837, 1682, 1600, 1494, 1456, 1424, 1382, 1371, 1285, 1257, 1229, 1098, 1044 cm-1; MS (70 eV) m/z 239 (M+•, 34%), 210 (52), 168 (98), 167 (100), 153 (33).
Compound 23. Paraformaldehyde (330 mg, 11.0 mmol) was added to a magnetically stirred solution of amine 22 (2.60 g, 10.88 mmol) in formic acid (15 mL) and the ensuing mixture stirred at 55 °C for 24 h before being cooled and concentrated under reduced pressure to give a light-yellow oil. This was dissolved in EtOH (35 mL), the resulting solution treated with oxalic acid (1.96 g, 21.8 mmol) and the slurry thus obtained concentrated under reduced pressure. The solid so-formed was washed with EtOH (2 15 mL) then dried under reduced pressure to give a white solid. This material, which is presumed to be the oxalate salt of the target tetrahydroisoquinoline, was not characterized but converted into the free base by suspending it in cold water (20 mL) and then adding KOH (673 mg, 12.0 mmol) in portions. The emulsion thus formed was extracted with Et2O (2 20 mL) and the combined organic phases were dried (MgSO4), filtered and concentrated under reduced pressure to give compound 23 (2.49 g, 91%) as an amber-coloured oil (Anal. Calcd for C14H21NO3 M+•, 251.1521. Found 251.1516). 1H NMR (300 MHz, CDCl3) δ 6.29 (s, 1H), 4.58 (septet, J = 6.0 Hz, 1H), 3.91 (s, 2H), 3.78 (s, 6H), 3.05 (t, J = 6.0 Hz, 2H), 2.62 (t, J = 6.0 Hz, 2H), 1.95 (broad s, 1H), 1.25 (d, J = 6.0 Hz, 6H); 13C NMR (75.5 MHz, CDCl3) δ 151.5 (C), 149.4 (C), 140.3 (C), 131.2 (C), 121.8 (C), 104.4 (CH), 74.7 (CH), 60.4 (CH3), 55.8 (CH3), 48.3 (CH2), 43.7 (CH2), 24.0 (CH2), 22.8 (CH3); νmax (KBr) 3311, 2972, 2933, 1601, 1492, 1465, 1452, 1424, 1381, 1323, 1298, 1276, 1238, 1222, 1176, 1116, 1027 cm–1; MS (70 eV) m/z 251 (M+•, 49%), 208 (52), 192 (56), 180 (100).
This material was used without purification in the next step of the reaction sequence.
Compound 24. Na2CO3 (100 mL of a 4% w/v aqueous solution) was added to a magnetically stirred solution of compound 23 (4.73 g, 18.8 mmol) in MeOH (15 mL) maintained at 18 °C and the ensuing mixture was treated with Fremy's salt (10.1 g, 37.7 mmol). The resulting dark-purple solution was stirred at 18 °C for 2 h then concentrated under reduced pressure and extracted with DCM (3 70 mL). The combined organic phases were dried (MgSO4), filtered and concentrated under reduced pressure. Subjection of the ensuing residue to column chromatography (alumina; Et2O then 98:2, 95:5 and 9:1 v/v Et2O/MeOH gradient elution) and concentration of the relevant fractions (Rf = 0.2 in 9:1 v/v Et2O/MeOH on silica gel) gave compound 24 (4.41 g, 94%) as a clear, colourless oil (Anal. Calcd for C14H19NO3: M+•, 249.1365. Found: M+•, 249.1365). 1H NMR (300 MHz, CDCl3) δ 8.15 (d, J = 2.1 Hz, 1H), 6.29 (s, 1H), 4.43 (septet, J = 6.3 Hz, 1H), 3.80 (s, 6H), 3.61 (dt, J = 7.8 and 2.1 Hz, 2H), 2.59 (t, J = 7.8 Hz, 2H), 1.20 (d, J = 6.3 Hz, 6H); 13C NMR (75.5 MHz, CDCl3) δ 159.5 (CH), 152.0 (C), 148.0 (C), 144.8 (C), 123.8 (C), 123.4 (C), 106.5 (CH), 75.3 (CH), 60.4 (CH3), 55.9 (CH3), 47.1 (CH2), 22.5 (CH3), 19.0 (CH2); νmax (KBr) 2973, 2937, 2841, 1629, 1596, 1571, 1489, 1454, 1421, 1367, 1352, 1318, 1124, 1110, 1100 cm–1; MS (70 eV) m/z 249 (M+•, 49%), 207 (50), 192 (100).
Compound 26. Butyllithium (5.25 mL of a 2.5 M solution in hexane, 13.15 mmol) was added, dropwise over 0.05 h, to a magnetically stirred solution of styrene 15 (2.30 g, 6.58 mmol) in THF (30 mL) maintained at –78 °C on a dry-ice acetone bath. The light-tan coloured solution thus obtained was allowed to stir for a further 0.83 h at –78 °C then anhydrous ZnCl2 (dried under high vacuum at 120 °C for 20 h) was added to the reaction mixture which slowly became colourless as it was warmed to 18 °C over 1 h. Pd(PPh3)4 (145 mg, 0.125 mmol) and aldehyde 185b,13 (2.00 g, 6.26 mmol) were then added to the reaction mixture which was stirred at 18 °C for 4 h before being diluted with EtOAc (150 mL) and washed with brine (2 100 mL). The separated organic phase was dried (MgSO4), filtered and concentrated on to TLC-grade silica (10 g). The resulting free-flowing solid was subjected to flash chromatography (silica gel; 1:1, 1:2 v/v hexane/DCM then 9:1 v/v DCM/EtOAc gradient elution). Concentration of the appropriate fractions (Rf = 0.4 in DCM) then afforded compound 26 (2.01 g, 84%) as white, crystalline masses, mp 121–122 °C (Anal. Calcd for C23H26O5: C, 72.23; H, 6.85. Found: C, 72.08; H, 6.92%). 1H NMR (300 MHz, CDCl3) δ 10.48 (s, 1H), 7.41 (s, 1H), 7.12 (d, J = 8.1 Hz, 1H), 7.04 (s, 2H), 6.87 (d, J = 8.1 Hz, 1H), 4.68 (septet, J = 6.0 Hz, 1H), 4.58 (septet, J = 6.0 Hz, 1H), 3.95 (s, 3H), 3.88 (s, 3H), 1.40 (d, J = 6.0 Hz, 12H); 13C NMR (75.5 MHz, CDCl3) δ 190.2 (CH), 154.4 (C), 149.7 (C), 148.1 (C), 147.6 (C), 129.6 (C), 124.7 (CH), 121.3 (C), 114.6 (CH), 114.5 (CH), 114.3 (CH), 110.6 (CH), 94.9 (C), 83.4 (C), 71.0(3) (CH), 70.9(7) (CH), 56.0 (CH3), 55.7 (CH3), 21.8 (CH3), 21.6 (CH3) (one signal obscured or overlapping); νmax (KBr) 2978, 2933, 2837, 2204, 1687, 1589, 1515, 1509, 1397, 1357, 1275, 1241, 1217, 1133, 953 cm–1; MS (70 eV) m/z 382 (M+•, 47%), 298 (100), 283 (54), 255 (18).
Compound 27. m-Chloroperoxybenzoic acid [1.20 g, ALDRICH, 50% (remainder 3-chlorobenzoic acid and water), ca. 7.0 mmol] was added, in portions over 0.25 h, to a magnetically stirred mixture of compound 26 (2.20 g, 5.75 mmol) and anhydrous KHCO3 (1.73 g, 17.3 mmol) in DCM (50 mL) maintained at 0 °C (ice-bath). The resulting slurry was stirred for a further 1 h between 0 and 18 °C then filtered through Celite™ and the retained solids were rinsed with DCM (1 50 mL). The combined filtrates were concentrated under reduced pressure and the residue thus obtained treated with NH3 (30 mL of a saturated methanolic solution). After 1 h at 18 °C the reaction mixture was concentrated under reduced pressure and the ensuing residue dissolved in DCM (200 mL) then concentrated onto TLC-grade silica gel (5.0 g). The resulting free-flowing solid was subjected to flash chromatography (silica gel; 2:1, 1:1 then 1:2 v/v hexane/DCM then DCM gradient elution). Concentration of the appropriate fractions (Rf = 0.3 in 1:2 v/v hexane/DCM) gave compound 27 (1.97 g, 92%) as white, crystalline masses, mp 130–131 °C (Anal. Calcd for C22H26O5: C, 71.33; H, 7.07. Found: C, 70.91; H, 7.24.%). 1H NMR (300 MHz, CDCl3) δ 7.03 (dd, J = 8.4 and 1.8 Hz, 1H), 7.00 (d, J = 1.8 Hz, 1H), 6.87 (s, 1H), 6.81 (d, J = 8.4 Hz, 1H), 6.53 (s, 1H), 5.81 (s, 1H), 4.52 (septet, J = 6.0 Hz, 2H), 3.82 (s, 3H), 3.78 (s, 3H), 1.35 (d, J = 6.0 Hz, 12H); 13C NMR (75.5 MHz, CDCl3) δ 151.8 (C), 149.7 (C), 149.3 (C), 147.8 (C), 143.6 (C), 124.6 (CH), 115.0 (C), 114.7 (CH), 114.6 (CH), 114.3 (CH), 102.0 (CH), 100.1 (C), 94.7 (C), 82.2 (C), 71.1 (CH), 71.0 (CH), 56.4 (CH3), 55.7 (CH3), 21.9 (CH3), 21.8 (CH3); νmax (KBr) 3404, 2981, 2935, 1620, 1599, 1573, 1513, 1467, 1450, 1418, 1383, 1373, 1330, 1269, 1240, 1214, 1166, 1135, 1113, 1027, 951, 863 cm–1; MS (70 eV) m/z 370 (M+•, 89%), 328 (18), 327 (20), 286 (100), 271 (42).
Compound 28. DCC (1.60 g, 7.75 mmol) was added to a magnetically stirred solution of α-iodoacetic acid (1.44 g, 7.74 mmol), compound 27 (2.60 g, 7.03 mmol) and DMAP (43 mg, 0.35 mmol) in DCM (30 mL) and the ensuing mixture stirred at 18 °C for 3 h then filtered through a sintered glass funnel. The retained solid was washed with DCM and the combined filtrates concentrated under reduced pressure. The residue thus obtained was suspended, via magnetic stirring, in Et2O (30 mL) at 0 °C then filtered [rinsing with Et2O (20 mL) pre-cooled to 0 °C] to give compound 28 (3.67 g, 97%) as a cream-coloured solid, mp 127–128 °C (Anal. Calcd for C24H27IO6: M+•, 538.0852. Found: M+•, 538.0854). 1H NMR (300 MHz, CDCl3) δ 7.08 (dd, J = 8.4 and 1.8 Hz, 1H), 7.04 (d, J = 1.8 Hz, 1H), 7.01 (s, 1H), 6.82 (d, J = 8.4 Hz, 1H), 6.65 (s, 1H), 4.53 (septet, J = 6.0 Hz, 2Hz), 3.95 (s, 2H), 3.85 (s, 6H), 1.38 (d, J = 6.0 Hz, 6H), 1.37 (d, J = 6.0 Hz, 6H); 13C NMR (75.5 MHz, CDCl3) δ 167.3 (C), 150.0 (C), 148.4 (C), 148.3 (C), 148.2 (C), 145.1 (C), 125.2 (CH), 115.6 (C), 115.3 (CH), 115.2 (CH), 115.1 (CH), 108.8 (CH), 93.6 (C), 82.8 (C), 72.0 (CH), 71.6 (CH), 56.6 (CH3), 56.3 (CH3), 22.3 (CH3), 22.0 (CH3), –6.2 (CH2) (one signal obscured or overlapping); νmax (KBr) 2969, 2930, 2833, 1755, 1611, 1575, 1516, 1467, 1416, 1402, 1385, 1365, 1320, 1252, 1236, 1212, 1187, 1175, 1157, 1135, 1108 954, 851 cm–1; MS (70 eV) m/z 538 (M+•, 80%), 496 (10), 328 (34), 286 (100), 196 (54), 153 (38), 86 (76), 84 (96).
Compound 30. 3,4-Dihydroisoquinoline 24 (1.20 g, 4.81 mmol) was added to a magnetically stirred solution of ester 28 (2.30 g, 4.27 mmol) in dry 1,2-dichloroethane (40 mL) and the resulting mixture maintained at 18 °C for 8 h then treated with diisopropylethylamine (750 µL, 4.30 mmol) and heated at reflux for 32 h. The cooled reaction mixture was treated with TLC-grade silica gel (6.0 g) then concentrated under reduced pressure and subjected to flash chromatography (silica gel; 2:1:0 then 2:3:1 v/v/v hexane/DCM/Et2O gradient elution). Concentration of the appropriate fractions (Rf = 0.4 in 5:5:2 v/v/v hexane/DCM/Et2O) gave compound 30 (2.28 g, 81%) as a white, crystalline solid, mp 244–245 °C (Anal. Calcd for C38H43NO9: M+•, 657.2938. Found M+•, 657.2938). 1H NMR (300 MHz, CDCl3) δ 7.10 (m, 2H), 7.05 (s, 1H), 6.92 (s, 1H), 6.64 (s, 1H), 6.60 (s, 1H), 4.74 (broad t, J = 6.6 Hz, 2H), 4.56 (m, 3H), 3.83 (s, 6H), 3.42 (s, 3H), 3.34 (s, 3H), 3.15 (broad t, J = 6.6 Hz, 2H), 1.41 (d, J = 6.0 Hz, 6H), 1.39 (d, J = 6.0 Hz, 6H), 1.31 (d, J = 6.0 Hz, 6H); 13C NMR (75.5 MHz, CDCl3) δ 155.6, 151.7, 151.2, 148.5, 147.0, 146.9, 146.5, 145.9, 142.5, 135.5, 128.5, 128.1, 123.3, 123.0, 121.1, 116.8, 115.5, 114.5, 113.8, 110.3, 104.9, 104.8, 104.7, 103.4, 75.7, 71.7, 71.4, 60.6, 56.1, 55.4, 55.1, 42.3, 22.7, 21.8, 21.7; νmax (KBr) 2974, 2935, 2832, 1702, 1620, 1539, 1506, 1476, 1465, 1444, 1420, 1259, 1237, 1203, 1175, 1161, 1110, 1083, 1040 cm–1; MS (70 eV) m/z 657 (M+•, 100%), 615 (44), 572 (9), 516 (11), 265 (13).
Lamellarin K (1). Aluminium chloride (1.33 g, 9.94 mmol) was added to a magnetically stirred solution of compound 30 (1.80 g, 2.76 mmol) in dry DCM (20 mL) and the ensuing mixture maintained at 18 °C for 4 h then treated with NH4Cl (20 mL of a saturated aqueous solution) and EtOAc (50 mL). The resulting mixture was washed with water (40 mL) and the separated aqueous layer extracted with EtOAc (2 40 mL). The combined organic phases were then dried (MgSO4), filtered and concentrated onto TLC-grade silica gel (8.0 g). The resulting free-flowing solid was subjected to flash chromatography (silica gel; 20:1, 10:1 then 5:1 v/v DCM/MeOH gradient elution). Concentration of the relevant fractions (Rf = 0.6 in 10:1 v/v DCM/MeOH) gave lamellarin K (1)4,20 (1.40 g, 95%) as a white, crystalline solid, mp 230–232 °C (lit.6 mp 196–198 °C) (Anal. Calcd for C29H25NO9: M+•, 531.1529. Found M+•, 531.1524). 1H NMR (300 MHz, CDCl3) δ 7.13 (d, J = 8.1 Hz, 1H), 7.07 (dd, J = 8.1 and 1.5 Hz, 1H), 6.97 (s, 1H), 6.96 (d, J = 1.5 Hz, 1H), 6.59 (s, 1H), 6.38 (s, 1H), 5.95 (s, 1H), 5.75 (s, 1H), 5.71 (s, 1H), 4.90 (m, 1H), 4.64 (m, 1H), 3.89 (s, 3H), 3.87 (s, 3H), 3.49 (s, 3H), 3.36 (s, 3H), 3.12 (m, 2H). 1H NMR [300 MHz, (CD3)2SO] δ see Table 1; 13C NMR [75.5 MHz, (CD3)2SO] δ see Table 1; νmax (KBr) 3472, 2940, 2839, 1709, 1667, 1600, 1549, 1511, 1479, 1458, 1428, 1407, 1265, 1207, 1142, 1122, 1031 cm–1; MS (70 eV) m/z 531 (M+•, 100%), 516 (18).
Compound 31. Compound 31 was prepared by Corey–Fuchs olefination of aldehyde 17 using a procedure defined earlier.13 The physical and spectroscopic data acquired on this material were in complete accord with those recorded13 previously.
Compound 33. Compound 33 was prepared from precursors 18 and 31 using a procedure defined earlier.13 The physical and spectroscopic data acquired on this material were in complete accord with those recorded13 previously.
Compound 34. Compound 34 was prepared by Dakin oxidation of aldehyde 33 using a procedure defined earlier.13 The physical and spectroscopic data acquired on this material were in complete accord with those recorded13 previously.
Compound 35. DCC (6.43 g, 31.1 mmol) was added to a magnetically stirred solution of α-iodoacetic acid (5.80 g, 31.2 mmol), phenol 34 (11.0 g, 29.7 mmol) and DMAP (36 mg, 0.30 mmol) in DCM (200 mL) and the resulting mixture maintained at 18 °C for 3 h then filtered and the solids thus retained rinsed with DCM (100 mL). The combined filtrates were concentrated under reduced pressure and the ensuing residue was suspended, via magnetic stirring, in Et2O (150 mL) at 0 °C. After 2 h the reaction mixture was filtered and the retained solids rinsed with Et2O (70 mL cooled to 0 °C) and thus giving ester 355b (15.0 g, 94%) as a cream solid, mp 136–137 °C (Anal. Calcd for C24H27IO6: M+•, 538.0852. Found M+•, 538.0843). 1H NMR (300 MHz, CDCl3) δ 7.12 (dd, J = 8.4 and 1.8 Hz, 1H), 7.06 (d, J = 1.8 Hz, 1H), 7.02 (s, 1H), 6.82 (d, J = 8.4 Hz, 1H), 6.66 (s, 1H), 4.55 (septet, J = 6.0 Hz, 2H), 3.96 (s, 2H), 3.87 (s, 6H), 1.40 (d, J = 6.0 Hz, 6H), 1.38 (d, J = 6.0 Hz, 6H); 13C NMR (75.5 MHz, CDCl3) δ 166.9 (C), 150.8 (C), 147.9 (C), 147.8 (C), 146.7 (C), 144.6 (C), 125.1 (CH), 118.3 (CH), 115.0 (C), 114.7 (CH), 111.5 (CH), 108.3 (CH), 93.2 (C), 82.3 (C), 71.5 (CH), 71.3 (CH), 56.1 (CH3), 55.8 (CH3), 22.0 (CH3), 21.8 (CH3), –6.6 (CH2) (one signal obscured or overlapping); νmax (KBr) 3021, 2978, 2930, 1771, 1607, 1512, 1460, 1444, 1420, 1330, 1241, 1211, 1133, 1110, 1096 cm–1; MS (70 eV) m/z 538 (M+•, 100%), 496 (12), 412 (18), 411 (17), 370 (26), 328 (62), 286 (81), 271 (57).
Compound 38. 3,4-Dihydroisoquinoline 3622 (986 mg, 4.46 mmol) was added to a magnetically stirred solution of ester 35 (2.0 g, 3.71 mmol) in dry 1,2-dichloroethane (50 mL) and the resulting mixture maintained at 18 °C for 15 h at which point diisopropylethylamine (677 µL, 3.90 mmol) was added and the reaction mixture then heated at reflux for 24 h. After this time the reaction mixture was cooled to 18 °C, treated with TLC-grade silica gel (5.0 g) and then concentrated under reduced pressure. The free-flowing solid thus obtained was subjected to flash chromatography (silica gel; 9:1 then 6:1 v/v DCM/Et2O gradient elution) and concentration of the appropriate fractions (Rf = 0.5 in 7:1 v/v DCM/Et2O) gave compound 38 (1.67 g, 71%) as a white, crystalline solid, mp 192–195 °C (Anal. Calcd for C36H39NO9: M+•, 629.2625. Found: M+•, 629.2642). 1H NMR (300 MHz, CDCl3) δ 7.07 (broad s, 2H), 7.03 (s, 1H), 6.89 (s, 1H), 6.63 (s, 1H), 6.56 (s, 1H), 4.74 (broad t, J = 6.6 Hz, 2H), 4.52 (m, 2H), 3.92 (s, 3H), 3.88 (s, 3H), 3.85 (s, 3H), 3.42 (s, 3H), 3.33 (s, 3H), 3.12 (broad t, J = 6.6 Hz, 2H), 1.36 (d, J = 6.0 Hz, 6H), 1.32 (d, J = 6.0 Hz, 6H); 13C NMR (75.5 MHz, CDCl3) δ 155.6 (C), 151.8 (C), 150.6 (C), 150.0 (C), 148.0 (C), 147.0 (C), 146.5 (C), 145.9 (C), 142.1 (C), 135.3 (C), 128.1 (C), 127.9 (C), 123.6 (CH), 123.0 (C), 120.0 (C), 117.7 (CH), 115.5 (C), 113.8 (C), 112.6 (CH), 110.3 (C), 105.1 (CH), 104.8 (CH), 103.3 (CH), 71.3 (CH), 71.2 (CH), 61.0 (CH3), 60.9 (CH3), 56.3 (CH3), 55.4 (CH3), 55.1 (CH3), 42.1 (CH2), 22.0 (CH3), 21.9 (CH2), 21.8 (CH3); νmax (KBr) 2975, 2935, 2834, 1700, 1620, 1540, 1506, 1476, 1455, 1442, 1416, 1259, 1239, 1208, 1175, 1156, 1137, 1116, 1084, 1037, 1013 cm–1; MS (70 eV) m/z 629 (M+•, 100%), 587 (70).
Lamellarin T (2). Aluminium chloride (480 mg, 3.60 mmol) was added to a magnetically stirred solution of compound 38 (945 mg, 1.50 mmol) in dry DCM (10 mL) and the ensuing mixture maintained at 18 °C for 0.5 h then treated with NH4Cl (5 mL of a saturated aqueous solution) and water (100 mL). The separated aqueous phase was extracted with EtOAc (3 100 mL) and the combined organic phases were dried (MgSO4), filtered and then treated with TLC-grade silica gel (4.0 g). The resulting mixture was concentrated under reduced pressure and the free-flowing solid thus obtained subjected to flash chromatography (silica: 99:1 then 20:1 v/v DCM/MeOH gradient elution). Concentration of the appropriate fractions (Rf = 0.2 in 20:1 v/v DCM/MeOH) gave lamellarin T (2)6,20b (737 mg, 90%) as a white, crystalline solid, mp 283–284 °C (lit.6 mp 214–218 °C; lit.20b mp >250 °C) (Anal. Calcd for C30H27NO9: M+•, 545.1686. Found: M+•, 545.1690). 1H NMR [300 MHz, (CD3)2SO] δ see Table 2; 13C NMR [75.5 MHz, (CD3)2SO] δ see Table 2; νmax (KBr) 3492, 3303, 2998, 2928, 2835, 1677, 1624, 1604, 1582, 1551, 1532, 1507, 1476, 1462, 1450, 1413, 1273, 1249, 1207, 1160, 1122, 1041, 1023, 859 cm–1; MS (70 eV) m/z 545 (M+•, 100%), 530 (31), 272 (24).
Compound 39. Lamellarin T (2) (48 mg, 0.09 mmol) was added to a magnetically stirred mixture of acetic anhydride (1.0 mL) and pyridine (1.0 mL) containing DMAP (ca. 2 mg) and the ensuing mixture maintained at 18 °C for 26 h then diluted with EtOAc (15 mL) and washed, sequentially, with NaHCO3 (20 mL of a saturated aqueous) then citric acid (20 mL of a 10% w/v aqueous solution) before being dried (MgSO4), filtered and concentrated under reduced pressure. The residue was thus obtained was subjected to flash chromatography (silica gel; 2:1 then 1:1 v/v hexane/EtOAc gradient elution). Concentration of the appropriate fractions (Rf = 0.4 in 1:1 v/v hexane/EtOAc) then gave diacetate 39 (47 mg, 83%) as a white, crystalline solid, mp 251–252 °C (Anal. Calcd for C34H31NO11: M+•, 629.1897. Found: M+•, 629.1907). 1H NMR (300 MHz, CDCl3) δ 7.31 (dd, J = 8.4 and 2.1 Hz, 1H), 7.22 (d, J = 2.1 Hz, 1H), 7.14 (d, J = 8.4 Hz, 1H), 7.07 (s, 1H), 6.70 (s, 1H), 6.48 (s, 1H), 4.90 (m, 1H), 4.60 (m, 1H), 3.90 (s, 3H), 3.89 (s, 3H), 3.86 (s, 3H), 3.45 (s, 3H), 3.39 (s, 3H), 3.13 (m, 2H), 2.30 (s, 6H); 13C NMR (75.5 MHz, CDCl3) δ 168.7 (C), 168.4 (C), 155.0 (C), 151.9 (C), 151.2 (C), 150.5 (C), 147.5 (C), 144.8 (C), 142.2 (C), 140.6 (C), 138.7 (C), 135.6 (C), 129.5 (CH), 127.6 (C), 127.2 (C), 125.4 (CH), 122.6 (C), 119.8 (C), 116.1 (C), 114.8 (C), 114.4 (C), 112.9 (CH), 111.7 (CH), 105.4 (CH), 105.0 (CH), 60.9 (CH3), 60.8 (CH3), 56.2 (CH3), 55.5 (CH3), 55.2 (CH3), 42.1 (CH2), 21.7 (CH2), 20.5 (CH3), 20.4 (CH3); νmax (KBr) 2937, 2840, 1769, 1718, 1603, 1545, 1507, 1475, 1456, 1442, 1414, 1385, 1370, 1295, 1266, 1201, 1141, 1131, 1117, 1084, 1036, 1013 cm–1; MS (70 eV) m/z 629 (M+•, 1%), 587 (8), 412 (10), 370 (32), 328 (100).
Compound 41. 3,4-Dihydro-6,7-dimethoxyisoquinoline (40)24 (425 mg, 2.23 mmol) was added to a magnetically stirred solution of compound 35 (1.00 g, 1.86 mmol) in dry 1,2-dichloroethane (30 mL). The resulting mixture was maintained at 18 °C for 17 h then treated with diisopropylethylamine (340 µL, 1.95 mmol) before being heated at 83 °C for 20 h then cooled, treated with TLC-grade silica gel (5.0 g) and concentrated under reduced pressure. The free-flowing solid thus obtained was subjected to flash chromatography (silica gel; 9:1 v/v DCM/Et2O elution) and concentration of the appropriate fractions (Rf = 0.5 in 7:1 v/v DCM/Et2O) gave compound 41 (780 mg, 70%) as a white, crystalline solid, mp 213–214 °C (Anal. Calcd for C35H37NO8: M+•, 599.2519. Found: M+•, 599.2526). 1H NMR (300 MHz, CDCl3) δ 7.01 (broad s, 2H), 7.03 (s, 1H), 6.87 (s, 1H), 6.74 (s, 1H), 6.69 (s, 1H), 6.65 (s, 1H), 4.75 (m, 2H), 4.50 (m, 2H), 3.90 (s, 3H), 3.86 (s, 3H), 3.41 (s, 3H), 3.35 (s, 3H), 3.10 (broad t, J = 6.9 Hz, 2H), 1.34 (d, J = 6.0 Hz, 6H), 1.32 (d, J = 6.0 Hz, 6H); 13C NMR (75.5 MHz, CDCl3) δ 155.6 (C), 150.0 (C), 148.8 (C), 147.9 (C), 147.4 (C), 146.9 (C), 146.4 (C), 145.9 (C), 135.8 (C), 128.2 (C), 127.8 (C), 126.5 (C), 123.6 (CH), 120.0 (C), 117.7 (CH), 114.8 (C), 113.6 (C), 112.5 (CH), 110.9 (CH), 110.3 (C), 108.5 (CH), 104.8 (CH), 103.3 (CH), 71.3 (CH), 71.2 (CH), 56.3 (CH3), 55.9 (CH3), 55.5 (CH3), 55.1 (CH3), 42.3 (CH2), 28.6 (CH2), 21.9 (CH3), 21.8 (CH3); νmax (KBr) 2975, 2936, 2836, 1694, 1620, 1608, 1580, 1542, 1511, 1484, 1463, 1440, 1415, 1271, 1259, 1241, 1213, 1167, 1043, 1026 cm–1; MS (70 eV) m/z 599 (M+•, 100%), 557 (81), 515 (41), 412 (45), 328 (82), 258 (37).
Lamellarin U (3). Aluminium chloride (315.8 mg, 2.36 mmol) was added to a magnetically stirred solution of compound 41 (430 mg, 0.72 mmol) in dry DCM (20 mL) and the ensuing mixture maintained at 18 °C for 14 h then treated with NH4Cl (10 mL of a saturated aqueous solution) and EtOAc (40 mL). The resulting mixture was washed with water (1 20 mL) and the separated aqueous layer extracted with EtOAc (2 20 mL). The combined organic phases were then dried (MgSO4), filtered and treated with TLC-grade silica gel (2.0 g). The ensuing mixture was concentrated under reduced pressure and the resulting free-flowing solid subjected to flash chromatography (silica gel; 20:1 then 10:1 v/v DCM/MeOH gradient elution). Concentration of the relevant fractions (Rf = 0.7 in 10:1 v/v DCM/MeOH) afforded lamellarin U (3)6,23 (347 mg, 94%) as white, crystalline solid, mp 242–243 °C (lit.6 mp 200–204 °C; lit.20b mp 247–250 °C; lit.23a mp 198–200 °C) (Anal. Calcd for C29H25NO8: M+•, 515.1580. Found: M+•, 515.1586). 1H NMR [300 MHz, (CD3)2SO] δ see Table 3; 13C NMR [75.5 MHz, (CD3)2SO] δ see Table 3; νmax (KBr) 3526, 3449, 3296, 3001, 2954, 2933, 2838, 1683, 1674, 1609, 1584, 1548, 1515, 1485, 1464, 1440, 1413, 1273, 1247, 1215, 1171, 1130, 1048, 1022, 865 cm–1; MS (70 eV) m/z 515 (M+•, 100%).
Compound 42. DDQ (219 mg 0.96 mmol) was added to a magnetically solution of compound 38 (485 mg, 0.77 mmol) in dry CHCl3 (10 mL) and the resulting mixture heated at reflux for 2 h then cooled, treated with TLC-grade silica gel (3.0 g) and concentrated under reduced pressure. The resulting free-flowing solid was subjected to flash chromatography (silica gel; 9:1 then 4:1 v/v DCM/Et2O gradient elution) and concentration of the appropriate fractions (Rf = 0.6 in 6:1 v/v DCM/Et2O) gave compound 42 (479 mg, 99%) as a white, crystalline solid, mp 200–201 °C (Anal. Calcd for C36H37NO9: M+•, 627.2468. Found: M+•, 627.2475). 1H NMR (300 MHz, CDCl3) δ 9.21 (d, J = 7.8 Hz, 1H), 7.38 (d, J = 7.8 Hz, 1H), 7.17 (broad s, 2H), 7.14 (s, 1H), 7.01 (s, 1H), 6.97 (s, 1H), 6.72 (s, 1H), 4.58 (m, 2H), 4.03 (s, 3H), 3.97 (s, 3H), 3.94 (s, 3H), 3.46 (s, 6H), 1.41 (d, J = 6.3 Hz, 6H), 1.36 (d, J = 6.0 Hz, 6H); 13C NMR (75.5 MHz, CDCl3) δ 155.5 (C), 153.2 (C), 150.2 (C), 148.4 (C), 148.2 (C), 147.9 (C), 146.6 (C), 146.5 (C), 142.6 (C), 133.8 (C), 129.3 (C), 128.1 (C), 124.0 (CH), 122.8 (CH), 121.3 (C), 119.3 (C), 114.1 (CH), 112.7 (CH), 111.9 (C), 109.8 (C), 108.1 (C), 106.8 (CH), 105.4 (CH), 103.3 (CH), 101.5 (CH), 71.3 (CH), 61.6 (CH3), 61.1 (CH3), 56.4 (CH3), 55.4 (CH3), 55.1 (CH3), 21.9 (CH3), 21.8 (CH3) (one signal obscured or overlapping); νmax (KBr) 2975, 2935, 2834, 1698, 1621, 1606, 1536, 1498, 1480, 1453, 1429, 1418, 1395, 1375, 1329, 1260, 1233, 1206, 1177, 1158, 1137, 1117, 1073, 1045, 975 cm–1; MS (70 eV) m/z 627 (M+•, 100%), 585 (85).
Lamellarin W (4). Aluminium chloride (112 mg, 0.836 mmol) was added to a magnetically stirred solution of compound 42 (175 mg, 0.279 mmol) in dry DCM (10.0 mL). The ensuing mixture was allowed to stir at 18 °C for 14 h then treated with NH4Cl (5 mL of a saturated aqueous) and water (40 mL) before being extracted with EtOAc (3 40 mL). The combined organic phases were then dried (MgSO4), filtered, treated with TLC-grade silica gel (2.0 g) and concentrated under reduced pressure. The resulting free-flowing solid was subjected to flash chromatography (silica gel; 99:1 then 20:1 v/v DCM/MeOH gradient elution) and concentration of the relevant fractions (Rf = 0.2 in 20:1 v/v DCM/MeOH) gave lamellarin W (4)6,20b (143 mg, 94%) as a white, crystalline solid, mp 284–286 °C (lit.6 mp 224–228 °C; lit.20b mp >250 °C) (Anal. Calcd for C30H25NO9: M+•, 543.1529. Found: M+•, 543.1533). 1H NMR [300 MHz, CDCl3/(CD3)2SO] δ see Table 4; 13C NMR [75.5 MHz, CDCl3/(CD3)2SO] δ see Table 4; νmax (KBr) 3413, 3135, 2937, 2841, 1667, 1606, 1481, 1424, 1382, 1275, 1240, 1207, 1176, 1156, 1116, 1075, 1045, 1021, 965 cm–1; MS (70 eV) m/z 543 (M+•, 100%).
Compound 43. The 3,4-dihydroisoquinoline 28 (170 mg, 0.82 mmol) was added to a magnetically solution of α-iodoacetate 40 (400 mg, 0.74 mmol) in dry 1,2-dichloroethane (4 mL) and the ensuing mixture maintained at 18 °C for 5 h. After this time diisopropylethylamine (136 µL, 0.78 mmol) was added to the reaction mixture which was heated at reflux for 28 h then cooled, treated with TLC-grade silica gel (3.0 g) and concentrated under reduced pressure. The free-flowing solid thus obtained was subjected to flash chromatography (silica gel; 1:2:0, 3:6:1 then 0:5:1 v/v/v hexane/DCM/Et2O gradient elution) and concentration of the appropriate fractions (Rf = 0.7 in 9:1 v/v DCM/Et2O) gave compound 43 (352 mg, 79%) as a white, crystalline solid, mp 222–223 °C (Anal. Calcd for C35H37NO8: M+•, 599.2519. Found: M+•, 599.2519). 1H NMR (300 MHz, CDCl3) δ 7.13–6.98 (complex m, 3H), 6.92 (s, 1H), 6.77 (s, 1H), 6.75 (s, 1H), 6.68 (s, 1H), 4.80 (m, 2H), 4.57 (m, 2H), 3.90 (s, 3H), 3.83 (s, 3H), 3.43 (s, 3H), 3.37 (s, 3H), 3.12 (broad t, J = 7.0 Hz, 2H), 1.41 (d, J = 6.0 Hz, 6H), 1.39 (d, J = 6.0 Hz, 6H); 13C NMR (75.5 MHz, CDCl3) δ 151.2 (C), 148.9 (C), 147.4 (C), 147.0 (C), 146.9 (C), 146.5 (C), 146.0 (C), 135.9 (C), 128.5 (C), 128.3 (C), 126.6 (C), 123.4 (CH), 120.1 (C), 116.8 (CH), 114.9 (C), 114.5 (CH), 113.7 (C), 110.9 (CH), 110.3 (C), 108.6 (CH), 104.8 (CH), 103.4 (CH), 71.7 (CH), 71.4 (CH), 56.2 (CH3), 55.9 (CH3), 55.5 (CH3), 55.1 (CH3), 42.4 (CH2), 28.7 (CH2), 21.9 (CH3), 21.8 (CH3) (one signal obscured or overlapping); νmax (KBr) 2975, 2933, 2831, 1709, 1611, 1578, 1543, 1514, 1485, 1464, 1438, 1415, 1383, 1339, 1271, 1240, 1212, 1165, 1138, 1042, 1008, 959, 858 cm–1; MS (70 eV) m/z 599 (M+•, 100%), 557 (61), 515 (49).
Compound 6. Aluminium chloride (80.3 mg, 0.60 mmol) was added to a magnetically stirred solution of compound 43 (120 mg, 0.20 mmol) in dry DCM (10 mL) and the ensuing mixture maintained at 18 °C for 1 h then treated with NH4Cl (10 mL of a saturated aqueous solution), EtOAc (40 mL) and water (40 mL). The separated aqueous phase was extracted with EtOAc (2 20 mL) and the combined organic phases were then dried (MgSO4), filtered and treated with TLC-grade silica gel (2.0 g) before being concentrated under reduced pressure. Subjection of the resulting free-flowing solid to flash chromatography (silica gel; 20:1 then 10:1 v/v DCM/MeOH gradient elution) and concentration of the relevant fractions (Rf = 0.7 in 10:1 v/v DCM/MeOH) gave 7-deoxylamellarin K (6) (92 mg, 89%) as a white, crystalline solid, mp 292–294 °C (Anal. Calcd for C29H25NO8: M+•, 515.1580. Found: M+•, 515.1576). 1H NMR [300 MHz, CDCl3/(CD3)2SO] δ 9.40 (s, 1H), 9.09 (s, 1H), 6.99 (d, J = 8.1 Hz, 1H), 6.96 (d, J = 1.5 Hz, 1H), 6.85 (dd, J = 8.1 and 1.5 Hz, 1H), 6.82 (s, 1H), 6.74 (s, 1H), 6.67 (s, 1H), 6.59 (s, 1H), 4.62 (m, 2H), 3.78 (s, 6H), 3.39 (s, 3H), 3.27 (s, 3H), 3.05 (broad t, J = 7.0 Hz, 2H); 13C NMR [75.5 MHz, CDCl3/(CD3)2SO] δ 152.9 (C), 147.0 (C), 146.7 (C), 145.3 (C), 145.0 (C), 144.9 (C), 144.1 (C), 142.7 (C), 133.8 (C), 126.2 (C), 124.8 (C), 123.9 (C), 121.8 (CH), 117.9 (C), 114.5 (CH), 113.0 (C), 112.8 (CH), 111.0 (C), 109.7 (CH), 107.3 (C), 107.0 (CH), 103.4 (CH), 101.9 (CH), 54.3 (CH3), 53.9 (CH3), 53.4 (CH3), 52.9 (CH3), 40.3 (CH2), 26.3 (CH2); νmax (KBr) 3529, 3105, 3003, 2937, 2833, 1667, 1609, 1546, 1520, 1486, 1464, 1439, 1416, 1274, 1256, 1234, 1217, 1184, 1163, 1047 cm–1; MS (70 eV) m/z 515 (M+•, 100%).
ACKNOWLEDGEMENTS
We thank the Institute of Advanced Studies for financial support and Professor Bruce Bowden (James Cook University) for providing copies of the 1H and 13C NMR spectra recorded on naturally-derived lamellarin K.
References
1. For reviews and recent key papers on the isolation, synthesis and/or biology of the lamellarins and related compounds see: (a) D. Pla, F. Albericio, and M. Álvarez, Med. Chem. Commun., 2011, 2, 689; CrossRef (b) T. Fukuda, F. Ishibashi, and M. Iwao, Heterocycles, 2011, 83, 491; CrossRef (c) Q. Li, J. Jiang, A. Fan, Y. Cui, and Y. Jia, Org. Lett., 2011, 13, 312; CrossRef (d) K. Hasse, A. C. Willis, and M. G. Banwell, Eur. J. Org. Chem., 2011, 88; CrossRef (e) P. Thipnate, M. Chittchang, N. Thasana, P. Saparpakorn, P. Ploypradith, and S. Hannongbua, Monatsh. Chem., 2011, 142, 97; CrossRef (f) C. Ballot, J. Kluza, S. Lancel, A. Martoriati, S. M. Hassoun, L. Mortier, J.-C. Vienne, G. Briand, P. Formstecher, C. Bailly, R. Nevière, and P. Marchetti, Apoptosis, 2010, 15, 769; CrossRef (g) S. Boonya-udtayan, N. Yotapan, C. Woo, C. J. Bruns, S. Ruchirawat, and N. Thasana, Chem. Asian J., 2010, 5, 2113; CrossRef (h) M. Chittchang, M. P. Gleeson, P. Ploypradith, and S. Ruchirawat, Eur. J. Med. Chem., 2010, 45, 2165; CrossRef (i) M. Chittchang, P. Batsomboon, S. Ruchirawat, and P. Ploypradith, ChemMedChem., 2009, 4, 457; CrossRef (j) H. Fan, J. Peng, M. T. Hamann, and J.-F. Hu, Chem. Rev., 2008, 108, 264; CrossRef (k) J. Kluza, P. Marchetti, and C. Bailly, in Modern Alkaloids: Structure, Isolation, Synthesis and Biology, ed. by E. Fattorusso and O. Taglialatela-Scafati, Wiley-VCH, Weinheim, 2008, Chapter 7; (l) D. Pla, F. Albericio, and M. Álvarez, Anti-Cancer Agents Med. Chem., 2008, 8, 746; (m) D. Baunbæk, N. Trinkler, Y. Ferandin, O. Lozach, P. Ploypradith, S. Rucirawat, F. Ishibashi, M. Iwao, and L. Meijer, Mar. Drugs, 2008, 6, 514; CrossRef (n) S. T. Handy and Y. Zhang, Org. Prep. Proced. Int., 2005, 37, 411; CrossRef (o) P. Cironi, F. Albericio, and M. Álvarez, Prog. Heterocycl. Chem., 2005, 16, 1; CrossRef (p) C. Bailly, Curr. Med. Chem. – Anti-Cancer Agents, 2004, 4, 363. CrossRef
2. S. Urban, L. Hobbs, J. N. A. Hooper, and R. J. Capon, Aust. J. Chem., 1995, 48, 1491. CrossRef
3. (a) M. Banwell, B. Flynn, and D. Hockless, Chem. Commun., 1997, 2259; CrossRef Aspects of the present work have also been disclosed in a patent: (b) M. G. Banwell and B. L. Flynn, Preparation of Fused Polycyclic Alkaloids by Ring Closure of Azomethine Ylides, Novel Compounds Thereof and their Use as Chemotherapeutic Agents, Int. Patent No. WO 9850365, 1998; US Patent No. US7122673 B2, 2006.
4. A. R. Carroll, B. F. Bowden, and J. C. Coll, Aust. J. Chem., 1993, 46, 489. CrossRef
5. (a) P. Cironi, I. Manzanares, F. Albericio, and M. Álvarez, Org. Lett., 2003, 5, 2959; CrossRef (b) C. P. Ridley, M. V. R. Reddy, G. Rocha, F. D. Bushman, and D. J. Faulkner, Bioorg. Med. Chem., 2002, 10, 3285; CrossRef For related approaches see: c) M. Díaz, E. Guitián, and L. Castedo, Synlett, 2001, 1164; CrossRef (d) M. Nyerges and L. Töke, Tetrahedron Lett., 2005, 46, 7531; CrossRef (e) S. Su and J. A. Porco Jr., J. Am. Chem. Soc., 2007, 129, 7744. CrossRef
6. M. V. R. Reddy, D. J. Faulkner, Y. Venkateswarlu, and M. R. Rao, Tetrahedron, 1997, 53, 345. CrossRef
7. L. Kürti and B. Czakó, Strategic Applications of Named Reactions in Organic Synthesis, Elsevier, Amsterdam, 2005, pp. 424–425.
8. A. Arcadi, S. Cacchi, M. Del Rosario, G. Fabrizi, and F. Marinelli, J. Org. Chem., 1996, 61, 9280. CrossRef
9. For a useful review on the intramolecular cycloaddition reactions of azomethine ylides, see: I. Coldham and R. Hufton, Chem. Rev., 2005, 105, 2765. CrossRef
10. (a) M. G. Banwell, B. L. Flynn, and S. G. Stewart, J. Org. Chem., 1998, 63, 9139; CrossRef (b) T. Sala and M. V. Sargent, J. Chem. Soc., Perkin Trans. 1, 1979, 2593; CrossRef (c) J. P. Gillespie, L. G. Amoros, and F. R. Stermitz, J. Org. Chem., 1974, 39, 3239; CrossRef (d) T. H. Simpson, J. Org. Chem., 1963, 28, 2107; CrossRef (e) C. Szántay, Acta Chim. Hung., 1957, 12, 83.
11. E. J. Corey and P. L. Fuchs, Tetrahedron Lett., 1972, 13, 3769. CrossRef
12. J. Bourgeois, I. Dion, P. H. Cebrowski, F. Loiseau, A.-C. Bédard, and A. M. Beauchemin, J. Am. Chem. Soc., 2009, 131, 874. CrossRef
13. M. G. Banwell, B. L. Flynn, A. C. Willis, and E. Hamel, Aust. J. Chem., 1999, 52, 767. CrossRef
14. X. Wan and M. M. Joullié, J. Am. Chem. Soc., 2008, 130, 17236. CrossRef
15. L. Kürti and B. Czakó, Strategic Applications of Named Reactions in Organic Synthesis, Elsevier, Amsterdam, 2005, pp. 202–203.
16. L. Kürti and B. Czakó, Strategic Applications of Named Reactions in Organic Synthesis, Elsevier, Amsterdam, 2005, pp. 348–349.
17. For related conversions, see: N. Sotomayor, E. Domínguez, and E. Lete, Tetrahedron, 1995, 51, 12721 and references cited therein. CrossRef
18. For a useful and up-to-date commentary on this rearrangement, see: E. Jahnke and R. R. Tykwinski, Chem. Commun., 2010, 46, 3235. CrossRef
19. L. Kürti and B. Czakó, Strategic Applications of Named Reactions in Organic Synthesis, Elsevier, Amsterdam, 2005, pp. 118–119.
20. Several other syntheses of lamellarin K have been reported: (a) C. Peschko, C. Winklhofer, A. Terpin, and W. Steglich, Synthesis, 2006, 3048; CrossRef (b) P. Ploypradith, T. Petchmanee, P. Sahakitpichan, N. D. Litvinas, and S. Ruchirawat, J. Org. Chem., 2006, 71, 9440; CrossRef (c) M. Díaz, E. Guitián, and L. Castedo, Synlett, 2001, 1164. CrossRef
21. Another synthesis of lamellarin T has been reported, see: Ref. 20b.
22. N. Thasana, B. Bjerke-Kroll, and S. Ruchirawat, Synlett, 2008, 505. CrossRef
23. Several other syntheses of lamellarin U have been reported: (a) J. C. Liermann and T. Opatz, J. Org. Chem., 2008, 73, 4526; CrossRef (b) see Ref. 5a; (c) see Ref. 20b.
24. R. N. Warrener, L. Liu, and R. A. Russell, Tetrahedron, 1998, 54, 7485. CrossRef
25. Another synthesis of lamellarin W has been reported, see: Ref. 20b.
26. Ruchirawat has demonstrated the utility of this reagent for generating pentacyclic lamellarins incorporating an unsaturated D-ring from their saturated counterparts, see: Ref. 20b.
27. W. C. Still, M. Kahn, and A. Mitra, J. Org. Chem., 1978, 43, 2923. CrossRef