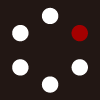
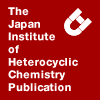
HETEROCYCLES
An International Journal for Reviews and Communications in Heterocyclic ChemistryWeb Edition ISSN: 1881-0942
Published online by The Japan Institute of Heterocyclic Chemistry
e-Journal
Full Text HTML
Received, 8th July, 2011, Accepted, 1st August, 2011, Published online, 11th August, 2011.
DOI: 10.3987/COM-11-S(P)79
■ Alkyne-Acetal Cyclisation Reactions Mediated by Formic Acid; 3-Acylated-2,5-dihydrofurans and Related Oxygen and Nitrogen Heterocycles
James D. Cuthbertson, Andrew A. Godfrey, William P. Unsworth, and Richard J. K. Taylor*
Department of Chemistry, University of York, Heslington, York YO10 5DD, U.K.
Abstract
The utility of formic acid for the cyclisation of alkyne ω-acetals is described; the scope and limitations of this process are outlined and a range of acylated heterocyclic building blocks (2,5-dihydrofurans, 2,5-dihydro-1H-pyrroles, tetrahydropyridines, 2H-chromenes, 1,2-dihydroquinolines and benzoxepin-5(2H)-ones) are reported.INTRODUCTION
Partially reduced heterocycles bearing acyl substituents are valuable synthetic building blocks and are also found in biologically active natural products including cycloepoxydon (1), anatoxin A (2) and myceliothermophin E (3) (Figure 1).1-3
As part of a natural product synthesis programme,4 we required ready access to a range of acylated dihydrofurans, dihydropyrroles, and higher homologues 5 of this type. Given our interest in tandem reactions,4,5 we became attracted to approaches based on the cyclisation of ω-formyl alkynes which can be mediated by both Lewis and Brønsted acids.6,7 As aliphatic aldehydes can be difficult to purify and prone to decomposition on storage, we explored the direct use of the corresponding alkyne ω-acetals 4 as cyclisation precursors (Scheme 1).8
RESULTS AND DISCUSSION
Initial studies were carried out using the known alkynyl acetal 4a,7e which was readily prepared from alcohol 6 and commercially available acetal 7 using a modification of the published procedure (HMPA was omitted).7e When the acetal is prepared using this method, its direct use in the subsequent cyclisation reaction, removing the need to hydrolyse to the aldehyde, is obviously beneficial. A range of Lewis and Brønsted acids have been employed for alkyne cyclisations,6,7 but our attention was taken by a report from Menashe and Shvo on the use of formic acid as an economical, metal-free method for the conversion of alkynes into carbonyl compounds.9 We therefore explored the use of these formic acid conditions for the conversion of acetal 4a into dihydrofuran 5a (Scheme 2). We were delighted to observe that, on heating acetal 4a in neat formic acid at 100 ºC, rapid conversion into dihydrofuran 5a took place and, after purification, compound 5a was isolated in 98% yield. The reaction was also found to proceed at lower temperatures, with a resulting increase in reaction time (e.g. rt, 24 h, 98%). The practicality of this procedure should be emphasised – on completion of the reaction, the formic acid can be simply removed in vacuo to give the product which can be used directly or further purified by chromatography.
Based on related studies, a number of mechanistic proposals could be considered, including: (i) initial addition of formic acid to the alkyne giving an intermediate 7 followed by an aldol-type condensation;7d (ii) proceeding by the intermediacy of an oxete 8;7a,7f,7g,10 (iii) a Prins-type cyclisation initiated by formation of oxonium ion 9.7e,g,h We have not carried out a detailed mechanistic study on this process but when the reaction was carried at rt and stopped after ~30 min, potential intermediates tentatively assigned as enol formates 10 {e.g. for 10a, HRMS (ESI): calcd. for C12H12NaO4, 243.0628. Found: [MNa]+, 243.0627 (0.1 ppm error); 1H NMR, 8.14 ppm, OCHO} were isolated in addition to the expected product 5a; this observation is in accord with the operation of a Prins-type process.
Having established conditions for the efficient cyclisation of acetal 4a, we were in a position to investigate the scope of the transformation. Thus, a range of substrates 4b-4g were prepared via alkylation of the corresponding propargylic alcohols or N-tosyl amines and subjected to the formic acid conditions (Table 1).
As can be seen from Table 1, all of the substrates 4a-4g were found to cyclise in moderate to excellent yield. In terms of dihydrofuran preparation, both aromatic and aliphatic alkyne substituents were tolerated (entries i-iv). The reaction was also found to be applicable to secondary and tertiary propargylic alcohols, which gave the methyl substituted dihydrofuran 5c and the spirocyclic example 5d, respectively. This methodology was equally applicable to the production of nitrogen heterocycles (entries v-vi); thus, N-Ts amine 4e gave 2,5-dihydro-1H-pyrrole 5e, and the higher homologue 4f gave tetrahydropyridine 5f. Finally (entry vii), an example is shown to illustrate that heteroatom-substituted alkynes are also compatible with this methodology.4c Alkyne 4g, easily available from L-proline, was treated with formic acid under the standard conditions giving almost quantitative cyclisation to the bicyclic thioester 5g {[α]D −87 (c 0.97, CHCl3)}. Such thioesters appear to be valuable synthetic building blocks and, indeed, thioester 5g has recently been employed by our group to prepare (−)-grandisine B and (+)-grandisine D via a novel synthetic route.4c
In the final part of this study, we examined the cyclisation reactions of phenol- and aniline-derived substrates 11, 12 and 15. On subjection to the standard reaction conditions, the aromatic precursors 11 and 12 were converted into the novel chromene and 1,2-dihydroquinoline derivatives 13 and 14, respectively, in reasonable yield. In all of the previous examples, cyclisation was observed at the proximal alkyne carbon atom but endo-cyclisation was seen when unsubstituted alkyne 15 was subjected to the reaction conditions. In this instance the known benzoxepinone derivative 1611 was isolated in 31% yield along with the formyl analogue 17 (39%; when re-subjected to the reaction conditions slow conversion of formate 17 into oxepine 16 was observed).
In summary, a simple formic acid procedure has been developed for the conversion of alkyne ω-acetals directly into acyl heterocyclic building blocks. Preliminary studies into the scope and limitations of the methodology have been investigated and the sequence has been utilised to prepare 2,5-dihydrofurans, 3,6-dihydro-2H-pyrans, 2,5-dihydro-1H-pyrroles, tetrahydropyridines, 2H-chromenes, 1,2-dihydroquinolines and benzoxepin-5(2H)-ones. We are currently exploring the use of several of these compounds in natural product synthesis.
EXPERIMENTAL
NMR spectra were recorded on a Jeol ECX-400 instrument at 400 MHz (1H) and 100 MHz (13C); chemical shifts (δ) are quoted in parts per million (ppm) calibrated to residual non-deuterated solvent (1H NMR: CDCl3 at 7.26 ppm; 13C NMR: CDCl3 at 77.0 ppm). Coupling constants (J) are quoted in Hertz and are to the nearest 0.1 Hz. Infrared spectra were recorded on a ThermoNicolet IR100 spectrometer with NaCl plates. Low resolution electrospray ionisation (ESI) mass spectra were recorded on a Kratos MS 25 spectrometer. High resolution mass spectra were recorded on a Bruker MicrOTOF spectrometer. Melting points were recorded on Gallenkamp apparatus and are uncorrected. Thin layer chromatography was performed on aluminium plates coated with Merck Silica gel 60 F254 and flash column chromatography was carried out using Fluka flash silica gel 60 and the specified eluent. PE refers to the fraction of petroleum ether that boils in the range 40-60 °C. Cyclisation substrates 4a,7e 4e,7e 4f,7f 4g4c and 2-(pent-1-ynyl)aniline12 were prepared using previously reported methods and other starting materials were prepared using related procedures. All other chemicals used in this study were commercially available and used as received.
Typical Alkyne-Acetal Cyclisation Procedure:
(2,5-Dihydrofuran-3-yl)(phenyl)methanone 5a: A stirred solution of alkyne 4a (62 mg, 0.25 mmol) in formic acid (1 mL) was heated at 100 ºC (oil-bath pre-heated) for 30 min. The solution was cooled to rt and then concentrated in vacuo to afford the crude product which was purified by column chromatography (SiO2, PE/EtOAc, 9:1) to give compound 5a (43 mg, 98%) as a crystalline solid; mp. 67-69 ºC; Rf 0.23 (PE/EtOAc, 4:1) (other data fully consistent to published values7a).
All other products were prepared using the above procedure. Products 5e,7a 5f,7f 5g4c and 1611 gave data fully consistent to published values. Data for novel products follow:
1-(2,5-Dihydrofuran-3-yl)propan-1-one 5b (22 mg, 70%); pale yellow oil; Rf 0.21 (PE/EtOAc, 4:1); IR (neat) 2855, 1738, 1124, 1074 cm−1; 1H NMR (400 MHz, CDCl3) δ 1.13 (t, J = 7.3 Hz, 3H), 2.72 (q, J = 7.3 Hz, 2H), 4.80-4.88 (m, 4H), 6.71-6.74 (m, 1H); 13C NMR (100 MHz, CDCl3) δ 7.9 (CH3), 32.7 (CH2), 74.4 (CH2), 76.3 (CH2), 136.3 (CH), 141.0 (C), 197.0 (C); m/z (ESI) 127 [MH]+; [HRMS (ESI): calcd. for C7H11O2, 127.0754. Found: [MH]+, 127.0757 (2.7 ppm error)].
(2-Methyl-2,5-dihydrofuran-3-yl)(phenyl)methanone 5c: purified by column chromatography (SiO2, PE/EtOAc, 9:1) as a pale yellow oil (38 mg, 80%); Rf 0.33 (PE/EtOAc, 4:1); IR (neat) 2973, 2848, 1644, 1359, 1276, 1240, 1084 cm−1; 1H NMR (400 MHz, CDCl3) δ 1.45 (d, J = 6.3 Hz, 3H), 4.77 (ddd, J = 16.1, 4.9, 1.9 Hz, 1H), 4.93 (ddd, J = 16.1, 5.6, 1.9 Hz, 1H), 5.30-5.38 (m, 1H), 6.54-6.56 (m, 1H), 7.44-7.49 (m, 2H), 7.54-7.60 (m, 1H), 7.77-7.81 (m, 2H); 13C NMR (100 MHz, CDCl3) δ 20.5 (CH3), 74.5 (CH2), 82.2 (CH), 128.4 (2 × CH), 128.8 (2 × CH), 132.6 (CH), 138.2 (C), 139.5 (CH), 143.5 (C), 191.4 (C); m/z (ESI) 189 [MH]+; [HRMS (ESI): calcd. for C12H13O2, 189.0910. Found: [MH]+, 189.0912 (1.2 ppm error)].
1-Oxaspiro[4.5]dec-3-en-4-yl(phenyl)methanone 5d: purified by column chromatography (SiO2, PE/EtOAc, 19:1 to 9:1) as a yellow oil (39 mg, 64%); Rf 0.49 (PE/EtOAc, 4:1); IR (neat) 2929, 1646, 1317, 1240, 1089 cm−1; 1H NMR (400 MHz, CDCl3) δ 1.23-1.36 (m, 1H), 1.61-1.76 (m, 7H), 2.00-2.11 (m, 2H), 4.75 (d, J = 1.9 Hz, 2H), 6.45 (t, J = 1.9 Hz, 1H), 7.42-7.47 (m, 2H), 7.53-7.58 (m, 1H), 7.73-7.77 (m, 2H); 13C NMR (100 MHz, CDCl3) δ 22.3 (2 × CH2), 25.0 (CH2), 34.1 (2 × CH2), 71.9 (CH2), 90.4 (C), 128.3 (2 × CH), 128.9 (2 × CH2), 132.4 (CH), 139.1 (C), 139.9 (CH), 145.5 (C), 192.4 (C); m/z (ESI) 265 [MNa]+; [HRMS (ESI): calcd. for C16H18NaO2, 265.1199. Found: [MNa]+, 265.1192 (2.5 ppm error)].
1-(2H-Chromen-4-yl)butan-1-one 13: purified by column chromatography (SiO2, PE/EtOAc, 9:1) as a yellow oil (29 mg, 57%); Rf 0.48 (PE/EtOAc, 4:1); IR (neat) 2964, 1682, 1485, 1456, 1226 cm−1; 1H NMR (400 MHz, CDCl3) δ 0.98 (t, J = 7.4 Hz, 3H), 1.72 (qt, J = 7.4, 7.3 Hz, 2H), 2.75 (t, J = 7.3 Hz, 2H), 4.82 (d, J = 4.1 Hz, 2H), 6.56 (t, J = 4.1 Hz, 1H), 6.87 (dd, J = 8.0, 1.3 Hz, 1H), 6.95 (ddd, J = 7.8, 7.6, 1.3 Hz, 1H), 7.18 (ddd, J = 8.0, 7.6, 1.6 Hz, 1H), 7.75 (dd, J = 7.8, 1.6 Hz, 1H); 13C NMR (100 MHz, CDCl3) δ 13.8 (CH3), 17.9 (CH2), 41.6 (CH2), 64.3 (CH2), 116.3 (CH), 119.7 (C), 121.6 (CH), 126.6 (CH), 128.9 (CH), 129.8 (CH), 135.1 (C), 154.3 (C), 200.7 (C); m/z (ESI) 225 [MNa]+; [HRMS (ESI): calcd. for C13H14NaO2, 225.0886. Found: [MNa]+, 225.0890 (1.8 ppm error)].
1-{1-[(4-Methylphenyl)sulfonyl]-1,2-dihydroquinolin-4-yl}butan-1-one 14: purified by column chromatography (SiO2, PE/EtOAc, 9:1) as a yellow oil (54 mg, 60%); Rf 0.33 (PE/EtOAc, 4:1); IR (neat) 2964, 1683, 1354, 1165, 1089 cm−1; 1H NMR (400 MHz, CDCl3) δ 0.85 (t, J = 7.4 Hz, 3H), 1.47 (qt, J = 7.4, 7.3 Hz, 2H), 2.24 (t, J = 7.3 Hz, 2H), 2.30 (s, 3H), 4.47 (d, J = 4.6 Hz, 2H), 6.24 (t, J = 4.6 Hz, 1H), 7.08 (d, J = 8.3 Hz, 2H), 7.27 (d, J = 8.3 Hz, 2H), 7.25-7.30 (m, 1H), 7.37 (ddd, J = 7.8, 7.7, 1.6 Hz, 1H), 7.61 (d, J = 7.8, 1.4, 1H), 7.73 (dd, J = 8.1, 1.6 Hz, 1H); 13C NMR (100 MHz, CDCl3) δ 13.7 (CH3), 17.5 (CH2), 21.4 (CH3), 41.2 (CH2), 44.8 (CH2), 126.7 (CH), 126.8 (C), 126.9 (CH), 127.2 (2 × CH), 127.7 (CH), 128.9 (CH), 129.3 (2 × CH), 130.7 (CH), 135.6 (C), 136.1 (C), 136.5 (C), 143.7 (C), 199.7 (C); m/z (ESI) 356 [MH]+; [HRMS (ESI): calcd. for C20H22NO3S, 356.1315. Found: [MH]+, 356.1313 (0.6 ppm error)].
5-Oxo-2,3,4,5-tetrahydrobenzo[b]oxepin-3-yl formate 17: purified by column chromatography (SiO2, PE/Et2O, 20:1 to 10:1) as a colourless crystalline solid (15 mg 39%); mp 60-62 °C; Rf 0.19 (PE/Et2O, 4:1); IR (thin film) 3429, 1718, 1653, 1475, 1302, 1165, 1108, 1012 cm−1; 1H NMR (400 MHz, CDCl3) δ 3.21 (dd, J = 12.3, 7.5 Hz, 1H), 3.28 (dd, J = 12.3, 6.0 Hz, 1H), 4.28-4.37 (m, 2H), 5.52-5.58 (m, 1H), 7.11-7.16 (m, 2H), 7.44-7.48 (m, 1H), 7.84 (dd, J = 7.9, 1.8, 1H), 8.06 (s, 1H); 13C NMR (100 MHz, CDCl3) δ 46.2 (CH2), 76.1 (CH), 76.7 (CH2), 120.7 (CH), 123.4 (CH), 127.8 (C), 129.8 (CH), 134.4 (CH), 159.9 (CH), 162.8 (C), 194.7 (C); ); m/z (ESI) 229 [MNa]+; [HRMS (ESI): calcd. for C11H10NaO4, 229.0471. Found: [MNa]+, 229.0470 (1.8 ppm error)].
ACKNOWLEDGEMENTS
The authors would like to thank the EPSRC and AstraZeneca for studentship support (J.D.C) and the EPSRC for postdoctoral support (W.P.U., EP/G068313/1).
References
1. A. Gehrt, G. Erkel, T. Anke, and O. Sterner, J. Antibiot., 1998, 51, 455.
2. J. P. Devlin, O. E. Edwards, P. R. Gorham, N. R. Hunter, R. K. Pike, and B. Stavric, Can. J. Chem., 1977, 55, 1367. CrossRef
3. Y.-L. Yang, C.-P. Lu, M.-Y. Chen, K.-Y. Chen, Y.-C. Wu, and S.-H. Wu, Chem. Eur. J., 2007, 13, 6985. CrossRef
4. (a) J. D. Cuthbertson, A. A. Godfrey, and R. J. K. Taylor, Synlett, 2010, 2805; CrossRef (b) J. D. Cuthbertson, A. A. Godfrey, and R. J. K. Taylor, Tetrahedron Lett., 2011, 52, 2024; CrossRef (c) J. D. Cuthbertson, A. A. Godfrey, and R. J. K. Taylor, Org. Lett., 2011, 13, 3976.
5. R. J. K. Taylor, M. Reid, J. Foot, and S. A. Raw, Acc. Chem. Res., 2005, 38, 851; CrossRef A. R. Burns, G. D. McAllister, S. E. Shanahan, and R. J. K. Taylor, Angew. Chem. Int. Ed., 2010, 49, 5574 and references therein. CrossRef
6. For the pioneering research on ω-carbonyl alkyne cyclisations see: (a) C. E. Harding and M. Hanack, Tetrahedron Lett., 1971, 12, 1253; CrossRef (b) R. J. Balf, B. Rao, and L. Weiler, Can. J. Chem., 1971, 49, 3135. CrossRef
7. For recent research on ω-carbonyl alkyne cyclisations see: (a) J. U. Rhee and M. J. Krische, Org. Lett., 2005, 7, 249; CrossRef (b) T. Jin and Y. Yamamoto, Org. Lett., 2007, 9, 5259; CrossRef (c) A. Saito, M. Umakoshi, N. Yagyu, and Y. Hanzawa, Org. Lett., 2008, 10, 1783; CrossRef (d) C. González-Rodríguez, L. Escalante, J. A. Varela, L. Castedo, and C. Saá, Org. Lett., 2009, 11, 1531; CrossRef (e) T. Xu, Z. Yu, and L. Wang, Org. Lett., 2009, 11, 2113; CrossRef (f) T. Jin, F. Yang, C. Liu, and Y. Yamamoto, Chem. Commun., 2009, 3533; CrossRef (g) T. Xu, Q. Yang, Z. Yu, D. Li, J. Dong, and Y. Li, Chem. Eur. J., 2010, 16, 9264; CrossRef (h) D. Zheng, W. Gong, Z. Ma, B. Ma, X. Zhao, Z. Xie, and Y. Li, Tetrahedron Lett., 2011, 52, 314 and references therein. CrossRef
8. To the best of our knowledge, there are only two reports of the use of alkyne-acetals in a Brønsted acid mediated cyclisation (reference 7d, footnote 8 and reference 7f).
9. N. Menashe and Y. Shvo, J. Org. Chem., 1993, 58, 7434. CrossRef
10. C. E. Harding and S. L. King, J. Org. Chem., 1992, 57, 883. CrossRef
11. S.-R. Li, H.-M. Chen, L.-Y. Chen, J.-C. Tsai, P.-Y. Chen,
S. C.-N. Hsu, and E.-C. Wang, ARKIVOC, 2008, (ii), 172.
12. L. F. Kuyper, D. P. Baccanari, M. L. Jones, R. N. Hunter, R. L. Tansik, S. S. Joyner, C. M. Boytos, S. K. Rudolph, V. Knick, H. R. Wilson, J. M. Caddell, H. S. Friedman, J. C. W. Comley, and J. N. Stables, J. Med. Chem., 1996, 392, 892. CrossRef