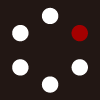
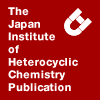
HETEROCYCLES
An International Journal for Reviews and Communications in Heterocyclic ChemistryWeb Edition ISSN: 1881-0942
Published online by The Japan Institute of Heterocyclic Chemistry
e-Journal
Full Text HTML
Received, 27th May, 2011, Accepted, 17th June, 2011, Published online, 28th June, 2011.
DOI: 10.3987/COM-11-S(P)26
■ Regioselective Ring-Opening Reaction of 2-Mono-Substituted Azetidin-3-ones Promoted by the Combined Use of Titanium Tetraiodide and Its Chloro or Bromo Counterpart
Shingo Hata, Daisuke Fukuda, Iwao Hachiya, and Makoto Shimizu*
Department of Chemistry for Materials, Graduate School of Engineering, Mie University, 1577 Tsu, Mie 514-8507, Japan
Abstract
The ring-opening reaction of 2-mono-substituted azetidin-3-ones at the more substituted bond occurred with the combined use of TiI4 and TiCl4 to give the corresponding ketones in moderate to high yields with high regioselectivity. The reductive aldol reaction of the enolate thus prepared proceeded with chloral in the presence of Pd(O2CCF3)2 to give the syn-aldol adduct in moderate yield.Azetidines are four-membered cycles including one nitrogen atom. Among them, most representative derivatives are azetidin-2-ones such as penicillin, cepharosporin, and thienamycin, etc. On the other hand, azetidin-3-ones have not been well studied because azetidin-3-ones do not exist in nature. However, azetidin-3-ones are proposed as intermediates for the synthesis of natural products or biologically active compounds containing an azetidine ring.1,2 We have reported titanium tetraiodide promoted aldol and Mannich-type reactions by the reductive generation of enolates via ring-opening of aziridines bearing carbonyl groups such as alkoxycarbonyls or keto carbonyls to give adducts in good to excellent yields.3 Aza-aldol reaction has also been reported using reductive ring-opening of 2-(1-benzyloxyiminoethyl)- aziridine to afford α-aminomethyl-β-hydroxy ketone O-alkyl oximes in good to high yields with anti-selectivities.4 In these reactions, it is crucial that the regioselective ring opening reaction of aziridines followed by reductive enolate formations, which are regarded as β-amino carbonyl compounds synthons. We next examined the reaction of azetidin-3-ones with TiI4 and found that the enolates were readily prepared followed by the subsequent aldol or Mannich-type reactions.5 In the reductive ring-opening reaction of 2-mono-substituted azetidin-3-ones, C-N bond cleavages occurred at the less sterically congested bond to give the ketone 2 preferentially. On the other hand, C-N bond cleavages of 2,2-disubstituted azetidin-3-ones proceeded at the more substituted bond to afford the only ketone 6 (Scheme 1). Regarding the different regioselectivity, we proposed two different pathways including SN2-like process by TiI4 or a one-electron transfer promoted by low-valent titanium species, which were in situ generated via the disproportion of TiI4. In order to realize the ring-opening reaction of 2-mono-substituted azetidin-3-ones at the more substituted bond, several conditions were examined. Herein, we describe regioselective ring-opening of 2-mono-substituted azetidin-3-ones with a combined use of TiI4 and titanium chloride or bromide.
First, effects of additives to reduce TiI4 to low-valent titanium species were examined in the model reaction of 2-mono-substituted azetidin-3-one 7, which is the most suitable substrate for the reductive aldol reaction.5 Although among them, Al powder was effective for the regioselectivity, the yield was low (entries 1-5 in Table 1). We next investigated several aluminum reagents. When AlBr3, AlCl3, and EtAlCl2 were used, ketone 9 was obtained in moderate yield with good regioselectivity (entries 6-10).6
The reductive aldol reaction of azetidin-3-one 7 with chloral was carried out with a combined use of TiI4 and AlBr3, AlCl3, or EtAlCl2. No desired aldol adduct 10 was obtained, and instead, those reactions gave ketones 8 and 9 or complex mixtures (Eq. 1).
Effects of other additives were investigated in the reaction of 2-mono-substituted azetidin-3-one 11. Table 2 summarizes the results. Among additives tested at room temperature, TiBr4 and TiCl4 were found to be efficient in both yields and regioselectivities (entries 5 and 6). The ring-opening reaction of 11 with a combined use of TiI4 and TiBr4 or TiCl4 at lower temperatures gave the desired ketone in high yields with good to high selectivities (entries 7 and 8).7
The reductive ring-opening reactions of several 2-mono-substituted azetidin-3-ones 1 were examined using TiI4-TiBr4 or TiCl4. Table 3 summarizes the results. When TiBr4 was used as an additive, the reductive ring-opening reaction of several 2-mono-substituted azetidin-3-ones regioselectively proceeded to give ketones in low to moderate yields (entries 1-3). Yields were improved using TiCl4 as an additive to give ketones in moderate to high yields with high regioselectivities (entries 4-6).
A plausible reaction mechanism is shown in Scheme 2. The disproportion of titanium tetraiodide and tetrachloride gives low-valent titanium species. A one-electron transfer to 2-mono-substituted azetidin-3-one 1 at the C-3 position gives a radical intermediate 14. The ring-opening reaction of 14 would proceed by fragmentation at the N-C(2) bond to give the more substituted titanium enolate 16, which is more stable than the less substituted one, and the subsequent protonation with water to quench the reaction would give the corresponding ketone 3.
Finally, we examined the reductive aldol reaction of the more substituted titanium enolate generated in situ with an aldehyde. The reaction of 2-mono-substituted azetidin-3-one 11 with chloral was carried out using TiI4-TiCl4 to give the aldol adduct 18 in 24% yield along with the reduction product 13 in 64% yields (Table 4, entry 1). To improve the yield, several Lewis acids were investigated as an additive (entries 2-7). Among metal salts examined, PdCl2 was slightly effective, and therefore, several palladium salts were examined (entries 8-12). As a result, Pd(O2CCF3)2 was found to be the most effective to give the aldol adduct in 66% yield with syn-selectivity.8 Although the role of Pd(O2CCF3)2 is not yet clear, we presumed that a transmetallation of titanium to palladium would occur to generate the palladium enolate 17, which would be responsible for the improvement of the yield under the present conditions.
In conclusion, we found that the ring-opening reaction of 2-mono-substituted azetidin-3-ones occurred at the more substituted bonds using TiI4-TiCl4 to give the corresponding ketones in moderate to high yields with high regioselectivity and also that the subsequent reductive aldol reaction with chloral proceeded in the presence of Pd(O2CCF3)2 to give the syn-aldol adduct in moderate yield.
References
1. For a review of the chemistry of azetidin-3-ones, see: Y. Dejaegher, N. M. Kuz’menok, A. M. Zvonok, and N. De Kimpe, Chem. Rev., 2002, 102, 29. CrossRef
2. For examples, see: (a) Y. K. Ramtohul, M. N. G. James, and J. C. Vederas, J. Org. Chem., 2002, 67, 3169; CrossRef (b) A. Salgado, M. Boeykens, C. Gauthier, J.-P. Declercq, and N. De Kimpe, Tetrahedron, 2002, 58, 2763; CrossRef (c) A. Salgado, Y. Dejaegher, G. Verniest, M. Boeykens, C. Gauthier, C. Lopin, K. A. Tehrani, and N. De Kimpe, Tetrahedron, 2003, 59, 2231; CrossRef (d) A. C. B. Burtoloso and C. R. D. Correia, Synlett, 2005, 1559; CrossRef (e) A. C. B. Burtoloso and C. R. D. Correia, Tetrahedron, 2008, 64, 9928. CrossRef
3. M. Shimizu, H. Kurokawa, S. Nishiura, and I. Hachiya, Heterocycles, 2006, 70, 57. CrossRef
4. M. Shimizu, S. Nishiura, and I. Hachiya, Heterocycles, 2007, 74, 177. CrossRef
5. S. Hata, D. Fukuda, I. Hachiya, and M. Shimizu, Chem. Asian J., 2010, 5, 473. CrossRef
6. Although the roles of AlCl3, AlBr3, and EtAlCl2 are not yet clear, we presume a mechanism as shown in Scheme 3. First, the reaction of TiI4 with aluminum salts may produce a more Lewis acidic titanium species A, which in turn activates the tosylamide nitrogen. An SN2-like reaction occurs at the carbon having a partially positive charge to generate the iodo ketone C and the subsequent reaction with another iodide anion effects formation of a titanium enolate species D, and the protonation with water to quench the reaction would give ketone 9. On the other hand, AlI3 and Et2AlCl were not effective because they are less Lewis acidic compared to the above aluminum salts and would not generate any complex like A.
7. Typical procedure (Table 2, entry 8): To TiI4 (222 mg, 0.40 mmol) was added TiCl4 (0.04 mL, 0.4 mmol) and CH2Cl2 (1.0 mL) at room temperature. The mixture was cooled to –78 oC and to it was added a solution of (S)-2-isobutyl-N-p-tosylazetidin-3-one (11) (56.2 mg, 0.20 mmol) in CH2Cl2 (1.0 mL) at –78 oC. The resulting mixture was gradually warmed up to room temperature during 15 h and sat. aq. NaHCO3, EtOAc (5.0 mL), and 10% aq. NaHSO3 were added to quench the reaction. The mixture was filtrated through a Celite pad. The layers were separated and the aqueous layer was extracted with EtOAc (20 mL x 3). The combined organic extracts were washed with brine, dried over anhydrous Na2SO4, and concentrated in vacuo to give a crude product. Purification on silica gel TLC (n-hexane/CH2Cl2/Et2O = 5/3/2) gave 5-methyl-1-(tosylamino)hexan-2-one (13) (24.9 mg, 88%). White solid. Mp 82-83 oC. 1H NMR (400 MHz, CDCl3): δ = 0.83 (d, J = 6.4 Hz, 6H), 1.34-1.49 (m, 3H), 2.33 (t, J = 7.8 Hz, 2H), 2.42 (s, 3H), 3.83 (d, J = 4.5 Hz, 2H), 5.39 (t, J = 4.5 Hz, 1H), 7.28-7.31 (m, 2H), 7.72-7.75 (m, 2H). 13C NMR (100 MHz, CDCl3): δ = 21.5, 22.1, 27.5, 32.2, 38.1, 51.2, 127.2, 129.7, 136.0, 143.7, 204.0. IR (KBr): 3464, 2953, 2871, 1719, 1495, 1467, 1405, 1370, 1346, 1323, 1159, 1119, 1092, 1064, 1019, 849, 812, 674 cm-1. HRMS (EI): Calcd for C14H21NO3S (M)+ 283.1242, found 283.1246.
8. Typical procedure (Table 4, entry 12): To a mixture of TiI4 (111 mg, 0.20 mmol), TiCl4 (0.02 mL, 0.2 mmol), and Pd(O2CCF3)2 (33.2 mg, 0.10 mmol) was added CH2Cl2 (1.0 mL) at room temperature. The mixture was cooled to 0 oC and to it was added successively a solution of (S)-2-isobutyl-N-p-tosylazetidin-3-one (11) (28.1 mg, 0.10 mmol) in CH2Cl2 (1.0 mL) and that of chloral (29.4 mg, 0.20 mmol) in CH2Cl2 (1.0 mL) at 0 oC. The resulting mixture was gradually warmed up to room temperature during 15 h and sat. aq. NaHCO3, EtOAc (5.0 mL), and 10% aq. NaHSO3 were added successively to quench the reaction. The mixture was filtrated through a Celite pad. The layers were separated and the aqueous layer was extracted with EtOAc (20 mL x 3). The combined organic extracts were washed with brine, dried over anhydrous Na2SO4, and concentrated in vacuo to give a crude product. Purification on silica gel TLC (n-hexane/CH2Cl2/Et2O = 2/3/2, twice) gave (R*)-3-((R*)-2,2,2-trichloro-1-hydroxyethyl)-5-methyl-1-(tosylamino)hexan-2-one (18) (28.5 mg, 66%). White solid. Mp 99-100 oC. 1H NMR (400 MHz, CDCl3): δ = 0.88 (d, J = 6.4 Hz, 3H), 0.92 (d, J = 6.6 Hz, 3H), 1.31-1.37 (m, 1H), 1.40-1.50 (m, 1H), 1.58-1.65 (m, 1H), 2.43 (s, 3H), 3.31 (ddd, J = 1.7, 6.3, 8.0 Hz, 1H), 3.98 (dd, J = 4.4, 20.0 Hz, 1H), 4.04 (dd, J = 4.4, 20.0 Hz, 1H), 4.11 (dd, J = 1.7, 9.0 Hz, 1H), 4.79 (d, J = 9.0 Hz, 1H), 5.28 (t, J = 4.4, Hz, 1H), 7.29-7.31 (m, 2H), 7.72-7.74 (m, 2H). 13C NMR (100 MHz, CDCl3): δ = 21.5, 21.9, 22.5, 25.4, 40.0, 44.8, 52.8, 85.2, 102.4, 127.3, 129.8, 135.9, 144.1, 209.0. IR (KBr): 3478, 3278, 2933, 2870, 1703, 1321, 1166, 1096, 934, 809, 782, 662, 588 cm-1. HRMS (EI): Calcd for C16H22Cl3NO4S (M)+ 429.0335, found 429.0346.