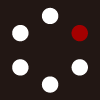
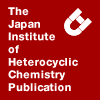
HETEROCYCLES
An International Journal for Reviews and Communications in Heterocyclic ChemistryWeb Edition ISSN: 1881-0942
Published online by The Japan Institute of Heterocyclic Chemistry
e-Journal
Full Text HTML
Received, 12th July, 2011, Accepted, 26th September, 2011, Published online, 30th September, 2011.
DOI: 10.3987/COM-11-S(P)81
■ Multicomponent Click Synthesis of Potentially Biologically Active Triazoles Catalysed by Copper Nanoparticles on Activated Carbon in Water
Francisco Alonso,* Yanina Moglie, Gabriel Radivoy, and Miguel Yus*
Department of Organic Chemistry, Faculty of Sciences and Institute of Organic Synthesis (ISO), University of Alicante, P.O. Box 99, 03080 Alicante, Spain
Abstract
A variety of potentially biologically active 1,2,3-triazoles, derived from (–)-menthol, lactic acid, D-glucose, oestrone, cholesterol, and phenacetin, have been synthesised through the multicomponent alkyne-azide 1,3-dipolar cycloaddition catalysed by copper nanoparticles on activated carbon in neat water.INTRODUCTION
Heterocycles play an important role in biochemical processes and, therefore, they are very frequently found as substructures in numerous pharmaceutical products.1 Among them, 1,2,3- and 1,2,4-triazoles are known to possess remarkable biological properties as antitumor, antiviral, anti-inflammatory, analgesic, antifungal or antibacterial agents.2 Nowadays, click chemistry3 represents a pivotal tool in the discovery of new therapeutic compounds and in medicinal chemistry since allows molecular diversity in a direct, precise and selective manner.4 In particular, the Huisgen 1,3-dipolar cycloaddition of azides and alkynes5 has become a synthetic cornerstone since the paramount discovery by the groups of Meldal6 and Sharpless7 of its copper(I)-catalysed version (CuAAC).8 This powerful methodology, which is considered the paradigm of a click reaction, gives a straight access into the 1,2,3-triazole moiety with high reliability and selectivity. This particular nucleus is used as a robust linker of complex and varied molecular architectures of significance in different fields, and especially in synthetic routes to bioactive molecules.
On the other hand, owing to our dedication to study and understand the reactivity of active metals and nanoparticles,9 we found out that active copper [obtained from CuCl2·2H2O, metal lithium, and a catalytic amount of 4,4'-di-tert-butylbiphenyl (DTBB) in THF at room temperature] was able to reduce various functional groups under very mild conditions.10 We discovered that copper nanoparticles (CuNPs) were formed either from CuCl2·2H2O or anhydrous CuCl2 under the aforementioned conditions. These CuNPs (10 mol%) effectively catalysed the 1,3-dipolar cycloaddition of organic azides and terminal alkynes in remarkably short reaction times (10–120 min).11 Notwithstanding the superior catalytic activity when compared with other commercially available copper sources, the CuNPs underwent dissolution under the reaction conditions (Et3N, THF, 65 ºC) and could not be reused. In order to overcome this inconvenience, we turned our attention to the development of supported catalysts based on CuNPs, which might be endowed of better recyclability and stability than the unsupported counterparts.12 We also tried to take advantage of the multicomponent domino synthesis of heterocycles, as an atom- and time-efficient method to construct valuable molecules in a one-pot operation.13 In this sense, we introduced a catalyst consisting of oxidized copper nanoparticles on activated carbon, readily prepared under mild conditions, which manifested a high versatility in the multicomponent Huisgen 1,3-dipolar cycloaddition in water.14 Not only organic halides, but some other azide precursors, such as aryldiazonium salts, anilines, epoxides and one alkene, were proven to be appropriate substrates in this process. In order to expand the applicability of this methodology, we wish to present herein our results on the multicomponent synthesis of an array of 1,2,3-triazoles, derived from some natural products and a synthetic one, with potential biological activity.
RESULTS AND DISCUSSION
The starting materials selected include the monoterpene alcohol (–)-menthol, methyl lactate, D-glucose pentaacetate, oestrone, cholesterol, and phenacetin. These compounds were properly derivatised prior to the click reaction by the introduction of a propargyl moiety or, in the case of D-glucose pentaacetate, by transformation into the bromo derivative. Except in the latter case, all the multicomponent reactions were carried out with sodium azide and benzyl bromide in water, using CuNPs/C as the catalyst, at a low metal loading (0.5 mol%).
Menthol is the major component of peppermint and cornmint oils. The mint oils can be considered as a kind of life elixirs according to their endless list of applications as well as their pharmacological and biological activities including:15 (a) in vitro antimicrobial, antimalarial and antigiradial activities; (b) antiviral activities against Newcastle disease, herpes simplex, vaccinia, Semliki Forest, and West Nile viruses in egg and cell culture systems; (c) inhibitory activity against HIV-1 reverse transcriptase; (d) spasmolytic activity on smooth muscles of experimental animals; (e) effective in alleviating the symptoms of irritable bowel syndrome; (f) as a spasmolytic in non-ulcer dyspepsia; (g) acetylcholinesterase inhibition and reversible antifertility. Peppermint oil is used as carminative, antiseptic and local anesthetic in cold, cough, and other preparations. It has been recommended as an adjunct to colonoscopy to reduce the colonic spasm. In traditional medicine, it has been applied as analgesic, stomachic, stimulant, antiseptic, local anesthetic, antispasmodic (in treating indigestion, nausea, sore throat, diarrhea, colds, headaches, toothaches, and cramps), and as an antitumor agent. One of the most important applications of mints is as flavouring agents in food products; e.g. chewing gums, alcoholic and non-alcoholic beverages, desserts, meat, etc. More recently, it has been discovered that menthol inhibits melanoma growth and that, due to its low toxicity, might be used with benefit in the treatment of this devastating disease.16
(–)-Menthol was the first substrate which was subjected to study under the conditions of our methodology.14 The multicomponent click reaction of propargyl menthyl ether 1 proceeded at 65 ºC to give, exclusively, the corresponding 1,2,3-triazole in good isolated yield (Scheme 1). The outcome of this reaction was similar to that previously found for phenyl propargyl ether, though rather slower.14 This heterocycle is new and it would be interesting to explore how the presence of the triazole unit can affect some of the multiple properties described above.
Lactic acid occurs in sour milk as a result of lactic acid bacteria. It can be also found in molasses due to the partial conversion of sugars, in apples and other fruits, tomato juice, beer, wines, opium, ergot, foxglove, and several higher plants, especially during germination.17 L-lactate is produced in muscles during exertion and is a common additive in animal nutrition since its health promoting properties enhance the performance of farm animals. It is a good descaler, soap-scum remover, and is widely applied in household cleaning products. Lactic acid is used as a natural and environmentally safe anti-bacterial agent in disinfecting products as well as an additive in food and drinking water. We prepared the propargyl ether of racemic methyl lactate which nicely underwent the alkyne-azide cycloaddition in water, giving rise to triazole 4 in excellent yield (Scheme 2). Despite the CuAAC has been widely studied in proteomics and peptidomimetics, its application to hydroxy acids has been neglected.18 In fact, and to the best of our knowledge, lactic acid derivatives (either acids or esters) of the type 4, bearing the triazolylmethyl moiety, have not been reported hitherto.
Glucose is a ubiquitous fuel in biology. It is used as an energy source in most organisms, from bacteria to humans. In the latter, glucose is a primary source of energy for the brain and hence its availability influences psychological processes. Glucose derivatives can also develop some patterns of biological action. For instance, it has been found that the two anomers of L-glucose pentaacetate stimulate insulin release and, therefore they could be used as novel insulinotropic tools in the treatment of non-insulin-dependent diabetes mellitus.19 The copper(I)-catalysed Huisgen cycloaddition has attracted the attention of carbohydrate synthetic chemists and glycobiologists in their efforts to clarify the role of carbohydrates in biological processes, as well as in the development of new therapeutics and vaccines.20 Pentacetylated D-glucose modified at C-4 with a 1,2,3-triazoyl unit has recently displayed herbicidal activity in pre-emergence tests.21 In order to prepare a triazole derived from glucose, α-D-glucose pentaacetate was transformed into the α-D-glucopyranosyl bromide 5. The domino nucleophilic substitution at the anomeric position and dipolar cycloaddition required prolonged heating at a higher temperature, leading to the expected β-D-glucopyranosyl triazole 6 in 70% isolated yield with inversion of the configuration (Scheme 3). To the best of our knowledge, there is only one method in the literature that reports the one-pot synthesis of 6.22 This method, which is fast and mild, cannot be considered a multicomponent but a sequential process, as the azide is generated prior to the 1,3-dipolar cycloaddition.
Oestrone is an oestrogenic hormone secreted by the ovary as well as by the adipose tissue. It occurs in pregnancy, postmenopausal women, urine of women and mares, in follicular liquor of many animals, in human placenta, in the urine of bulls and in palm-kernel oil.23 Oestrogens have key roles in the development and maintenance of normal sexual and reproductive function. Although traditionally presumed to be a female hormone, oestrone also exerts a vast range of biological effects in the cardiovascular, skeletal, immune, and central nervous systems.24 In addition to the physiological role of oestrone, it can induce tumors in various organs of several laboratory animal species. The aryl propargyl ether moiety in modified oestrone 7 exhibited very near behaviour to that of the simpler phenyl propargyl ether in the CuNPs/C-catalysed dipolar cycloaddition, as regards both reaction time (8 vs. 7 h) and isolated yield (76 vs. 81%) (Scheme 4). In contrast with the oestrogen 17α-ethynyloestradiol, for which the synthesis of the corresponding triazoles was accomplished by us11 and other groups, compound 8 represents the first triazolyl derivative of oestrone ever synthesised.
Cholesterol is the principal sterol in the higher animals. It can be found in all body tissues, especially in the brain, spinal cord, and in animal fats or oils. It is used to produce hormones, bile acids, vitamin D, cell membranes and is transported in the blood plasma of all mammals.25 Although cholesterol is important and necessary for mammals, high levels of cholesterol in the blood can damage arteries and are potentially linked to diseases such as those associated with the cardiovascular system. It has also been found to play an important role in myelin biogenesis and myelin disease.26 A very recent study reveals that 2'-triazolyl-2-deoxyuridines with a cholesterol moiety anchor in biocompatible phospholipid membranes without affecting the order in the double layers, thus demonstrating that the membranes can be equipped with nucleobases through this approach.27 Following our multicomponent approach, a new cholesterol derivative 10, with a pendant triazolylmethyl unit, was synthesised in good yield under the standard conditions (Scheme 5). It is noteworthy that, both the reaction time and yield are akin to those noted for (–)-menthol, as in these two examples a cyclohexyl propargyl ether moiety is involved.
Phenacetin is a synthetic analgesic and antipyretic introduced in 1887, and widely used until the third quarter of the twentieth century.28 However, it is no longer used in therapy because of its toxicity. The FDA ordered the withdrawal of drugs containing phenacetin in November 1983, owing to its carcinogenic and kidney-damaging properties.29 In this case, we modified the starting material by anchoring the propargyl substituent to the nitrogen of the acetamido group. Under the CuNPs/C catalysis, a new triazole 12 was produced in high isolated yield after 8 h (Scheme 6). We must point out that the reactivity shown by 11 was near identical to that observed for N-propargylphthalimide in our previous study (i.e., 8 h, 84% yield), under the same reaction conditions.14 Only the corresponding biological/pharmacological studies will unveil whether compound 12 has the same deleterious effects as phenacetin or improved therapeutical properties.
In conclusion, we have demonstrated that the multicomponent click synthesis of 1,2,3-triazoles, catalysed by copper nanoparticles on activated carbon in water, is an adequate and direct method to prepare potentially biologically active compounds. A series of new heterocyclic compounds, derived from the natural products (–)-menthol, lactic acid, D-glucose, oestrone, and cholesterol, and from the synthetic compound phenacetin, have been prepared in 70–95% yield. The reactivity of the starting materials resembles that of simpler propargyl derivatives possessing the same structural features. The possible biological activity of the new triazoles will be tested in a near future.
EXPERIMENTAL
Melting points were obtained with a Reichert Thermovar apparatus. Optical rotations were measured with a Perkin-Elmer 341 polarimeter with a thermally jacketted 5 cm cell at approximately 20 ºC. Concentrations (c) are given in g/100 ml and [α] values are given in units of 10–1 deg cm2 g–1. Infrared analysis was performed with a Jasco 4100LE (Pike MIRacle ATR) spectrophotometer; wavenumbers are given in cm–1. NMR spectra were recorded on Bruker Avance 300 and 400 spectrometers (300 and 400 MHz for 1H NMR; 75 and 100 MHz for 13C NMR); chemical shifts are given in (δ) parts per million and coupling constants (J) in Hertz. The retention times and mass spectra (EI) were obtained at 70 eV on an Agilent 5973 spectrometer connected to a HP 6890 gas chromatograph, equipped with a HP-5MS 30 m capillary column (0.25 mm diameter, 0.25 µm film thickness), using helium (1 mL/min) as carrier gas, Tinjector = 270 ºC, Tcolumn = 60 ºC (3 min) and 60–270 ºC (15 ºC/min); retention times (tR) are given in min, fragment ions in m/z with relative intensities (%) in parenthesis. HRMS analyses were carried out on a Finnigan MAT95S spectrometer.
Starting materials. Anhydrous copper(II) chloride (Aldrich), lithium powder (MEDALCHEMY S. L.), DTBB (4,4'-di-tert-butylbiphenyl, Aldrich), activated charcoal (Norit CA1, Aldrich), and sodium azide (Across) were commercially available. All the starting materials and other reagents were commercially available of the best grade (Aldrich, Acros, Alfa Aesar) and were used without further purification. THF was dried in a Sharlab PS-400-3MD solvent purification system using an alumina column. Propargylation of the substrates was done following a literature procedure.30 Tetra-O-acetyl-α-D-glucopyranosyl bromide 5 was synthesised from D-glucose pentaacetate according to a literature procedure.31
(1S,2S,5R)-Menthyl propargyl ether (1): All the physical and spectroscopic data were in agreement with those reported in the literature.30
(±)-Methyl 2-(prop-2-ynyloxy)propanoate (3): yellow liquid; tR 5.00; Rf 0.85 (hexane/EtOAc 4:1); IR 3274, 2928, 2857, 1732, 1456, 1275, 1202, 1125, 1072, 1031 cm–1; 1H NMR (300 MHz, CDCl3) δ 1.44 (d, J = 6.9 Hz, 3H), 2.47 (t, J = 2.4 Hz, 1H), 3.76 (s, 3H), 4.19, 4.34 (2dd, J = 16.1, 2.4 Hz, 2H), 4.27 (q, J = 6.9 Hz, 1H); 13C NMR (CDCl3) δ 18.35, 51.89, 56.96, 72.87, 74.98, 78.77, 172.98; MS m/z 142 (M+, 1), 88 (16), 83 (100), 59 (11), 55 (17). HRMS (EI) m/z Calcd for C7H10O3: 142.0630. Found: 142.0638.
Tetra-O-acetyl-α-D-glucopyranosyl bromide (5): All the physical and spectroscopic data were in agreement with those reported in the literature.31
O-Propargyloestrone (7): white solid; mp 140.8–143.0 ºC; tR 23.62; Rf 0.57 (hexane/EtOAc 4:1); IR 3295, 2916, 2865, 2160, 1732, 1604, 1497, 1308, 1286, 1260, 1223, 1024, 819, 694, 644 cm–1; 1H NMR (300 MHz, CDCl3) δ 0.91 (s, 3H), 1.35–1.72 (m, 6H), 1.90–2.58 (m, 8H), 2.85–2.96 (m, 2H), 4.66 (d, J = 2.4 Hz, 2H), 6.71 (d, J = 2.4 Hz, 1H), 6.79 (dd, J = 8.4, 2.7 Hz, 1H), 7.22 (d, J = 8.4 Hz, 1H); 13C NMR (CDCl3) δ 13.82, 21.56, 25.85, 26.48, 29.62, 30.91, 31.55, 35.84, 38.26, 43.96, 50.38, 55.70, 75.31, 78.77, 112.32, 114.93, 126.34, 132.96, 137.85, 155.52, 220.85; MS m/z 309 (M++1, 22), 308 (M+, 100), 307 (48), 223 (17), 210 (12), 209 (11), 207 (19), 197 (12), 184 (20), 183 (22), 181 (11), 169 (12), 152 (10), 128 (15), 115 (14), 55 (11). HRMS (EI) m/z Calcd for C21H24O2: 308.1776. Found: 308.1778.
O-Propargylcholesterol (9): All the physical and spectroscopic data were in agreement with those reported in the literature.32
N-(4-Ethoxyphenyl)-N-(prop-2-yn-1-yl)acetamide (11): yellow liquid; tR 13.47; Rf 0.58 (hexane/EtOAc 1:1); IR 3290, 2980, 2931, 1657, 1508, 1477, 1422, 1389, 1288, 1244, 1222, 1044, 922, 840, 738, 642, 623 cm–1; 1H NMR (300 MHz, CDCl3) δ 1.44 (t, J = 6.9 Hz, 3H), 1.85 (s, 3H), 2.21 (t, J = 2.5 Hz, 1H), 4.06 (q, J = 6.9 Hz, 2H), 4.44 (d, J = 2.5 Hz, 2H), 6.93, 7.19 (AA'BB' system, J = 8.7, 4H); 13C NMR (CDCl3) δ 14.60, 22.15, 38.14, 63.55, 71.80, 79.15, 115.08, 128.97, 134.62, 158.58, 170.32; MS m/z 217 (M+, 60), 175 (59), 174 (10), 147 (21), 146 (100), 136 (10), 108 (28), 91 (10), 80 (16). HRMS (EI) m/z Calcd for C13H15NO2: 217.1103. Found: 217.1097.
Typical procedure for the preparation of CuNPs/C: Anhydrous copper(II) chloride (135 mg, 1 mmol) was added to a suspension of lithium (14 mg, 2 mmol) and 4,4'-di-tert-butylbiphenyl (DTBB, 27 mg, 0.1 mmol) in THF (2 mL) at room temperature under an argon atmosphere. The reaction mixture, which was initially dark blue, rapidly changed to black, indicating that the suspension of CuNPs was formed. This suspension was diluted with THF (18 mL) followed by the addition of the activated carbon (1.28 g). The resulting mixture was stirred for 1 h at room temperature, filtered, and the solid successively washed with water (20 mL), THF (20 mL) and dried under vacuum.
General procedure for the CuNPs/C-catalysed click reaction. NaN3 (72 mg, 1.1 mmol), the bromide (1 mmol), and the alkyne (1 mmol) were added to a suspension of CuNPs/C (20 mg, 0.5 mol% Cu) in H2O (2 mL). The reaction mixture was warmed to 70 ºC and monitored by TLC and/or GLC until total conversion of the starting materials. Water (30 mL) was added to the resulting mixture, followed by extraction with EtOAc (3 × 10 mL). The collected organic phases were dried with anhydrous MgSO4 and the solvent was removed in vacuo to give the corresponding triazoles, which were purified by recrystallisation in EtOAc.
1-Benzyl-4-[(1R,2S,5R)-menthyloxymethyl]-1H-1,2,3-triazole (2): white solid; mp 46.4–48.0 ºC; tR 19.95; Rf 0.66 (hexane/EtOAc 3:2); [α]D20 –64.5 (c 1.0, CHCl3); IR 3056, 2911, 2867, 2845, 1455, 1218, 1123, 1085, 1070, 1052, 762, 726, 718, 698 cm–1; 1H NMR (400 MHz, CDCl3) δ 0.60 (d, J = 7.2 Hz, 3H), 0.84, 0.91 (2d, J = 6.6 Hz, 6H), 0.79–0.96 (m, 2H), 1.14–1.42 (m, 3H), 1.54–1.68 (m, 2H), 2.05–2.19 (m, 2H), 3.17 (dt, J = 10.4, 4.4 Hz, 1H), 4.55, 4.74 (2d, J = 12.6 Hz, 2H), 5.51 (s, 2H), 7.23–7.30 (m, 2H), 7.32–7.41 (m, 3H), 7.44 (s, 1H); 13C NMR (CDCl3) δ 16.02, 20.90, 22.25, 23.20, 25.22, 31.41, 34.43, 40.16, 48.09, 54.06, 61.88, 78.66, 122.16, 128.03, 128.64, 129.00, 134.61, 146.58; MS m/z 327 (M+, 1), 190 (16), 188 (23), 174 (11), 173 (72), 144 (30), 95 (21), 91 (100), 83 (10), 81 (19), 69 (11), 67 (12), 55 (17). HRMS (EI) m/z Calcd for C20H29N3O: 327.2311. Found: 327.2315.
(±)-Methyl 2-[(1-benzyl-1H-1,2,3-triazol-4-yl)methoxy]propanoate (4): yellow liquid; tR 16.95; Rf 0.23 (hexane/EtOAc 3:2); IR 2987, 2951, 1742, 1497, 1455, 1208, 1120, 1066, 1048, 1030, 755, 720, 698 cm–1; 1H NMR (300 MHz, CDCl3) δ 1.40 (d, J = 6.9 Hz, 3H), 3.71 (s, 3H), 4.13 (q, J = 6.9 Hz, 1H), 4.61, 4.74 (2d, J = 12.3 Hz, 2H), 5.51 (s, 2H), 7.22–7.30 (m, 2H), 7.32–7.41 (m, 3H), 7.56 (s, 1H); 13C NMR (CDCl3) δ 18.33, 51.70, 53.85, 63.19, 73.94, 122.58, 127.88, 128.46, 128.82, 134.34, 144.69, 173.15; MS m/z 275 (M+, 1), 188 (55), 144 (25), 91 (100), 65 (10). HRMS (EI) m/z Calcd for C14H17N3O3: 275.1270. Found: 275.1286.
1-(2,3,4,6-Tetra-O-acetyl-β-D-glucopyranosyl)-4-phenyl-1H-1,2,3-triazole (6): All the physical and spectroscopic data were in agreement with those reported in the literature.22
O-[(1-Benzyl-1H-1,2,3-triazol-4-yl)methyl]oestrone (8): white solid; mp 151.9–154.9 ºC; Rf 0.51 (hexane/EtOAc 1:1); [α]D20 +88.0 (c 1.0, CHCl3); IR 2924, 1734, 1612, 1578, 1453, 1279, 1256, 1245, 1160, 1065, 1048, 1005, 818, 731, 722, 710, 691 cm–1; 1H NMR (300 MHz, CDCl3) δ 0.90 (s, 3H), 1.35–1.74 (m, 6H), 1.89–2.29 (m, 5H), 2.33–2.59 (m, 2H), 2.80–2.95 (m, 2H), 5.16 (s, 2H), 5.53 (s, 2H), 6.71 (d, J = 2.7 Hz, 1H), 6.77 (dd, J = 8.7, 2.7 Hz, 1H), 7.24–7.31 (m, 3H), 7.34–7.40 (m, 3H), 7.51 (s, 1H); 13C NMR (CDCl3) δ 13.82, 21.55, 25.85, 26.47, 29.60, 31.53, 35.84, 38.27, 43.94, 47.97, 50.37, 54.21, 62.11, 112.35, 114.74, 122.47, 126.36, 128.10, 128.77, 129.11, 132.62, 134.43, 137.84, 144.85, 156.22, 220.92; MS m/z 441 (M+, 27), 270 (14), 145 (13), 144 (91), 115 (10), 91 (100). HRMS (EI) m/z Calcd for C28H31N3O2: 441.2416. Found: 441.2418.
O-[(1-Benzyl-1H-1,2,3-triazol-4-yl)methyl]cholesterol (10): white solid; mp 110.1–113.4 ºC; Rf 0.38 (hexane/EtOAc 7:3); [α]D20 –17.1 (c 1.0, CHCl3); IR 3087, 2933, 2864, 1457, 1438, 1377, 1365, 1332, 1220, 1985, 1052, 1023, 799, 721, 700 cm–1; 1H NMR (300 MHz, CDCl3) δ 0.70 (s, 3H), 0.89 (dd, J = 6.6, 1.2 Hz, 6H), 0.94 (d, J = 6.6 Hz, 3H), 1.01 (s, 3H), 0.83–1.72 (m, 22H), 1.79–2.08 (m, 5H), 2.21–2.31 (m, 1H), 2.35–2.47 (m, 1H), 3.23–3.41 (m, 1H), 4.68 (s, 2H), 5.36 (d, J = 5.7 Hz, 1H), 5.53 (s, 2H), 7.27–7.33 (m, 2H), 7.36–7.42 (m, 2H), 7.47 (s, 1H); 13C NMR (CDCl3) δ 11.83, 18.69, 19.33, 21.03, 22.54, 22.80, 23.79, 24.26, 27.99, 28.21, 31.86, 31.91, 35.76, 36.16, 36.81, 37.14, 38.99, 39.50, 39.75, 42.29, 50.13, 54.14, 56.12, 56.73, 61.69, 77.20, 78.97, 121.79, 122.15, 128.16, 128.72, 129.08, 134.57, 140.65, 146.40; MS m/z 557 (M+, 1), 368 (13), 191 (12), 190 (100), 91 (35). HRMS (EI) m/z Calcd for C37H55N3O2: 557.4345. Found: 557.4337.
N-[(1-Benzyl-1H-1,2,3-triazol-4-yl)methyl]-N-(4-ethoxyphenyl)acetamide (12): pale yellow solid; mp 83.6–85.6 ºC; tR 21.17; Rf 0.30 (hexane/EtOAc 3:7); IR 3065, 2979, 2940, 1649, 1509, 1395, 1288, 1243, 1224, 1046, 840, 736, 723, 704 cm–1; 1H NMR (300 MHz, CDCl3) δ 1.41 (t, J = 6.9 Hz, 3H), 1.82 (s, 3H), 4.00 (q, J = 7.2 Hz, 2H), 4.85 (s, 2H), 5.49 (s, 2H), 6.83, 6.99 (AA'BB' system, J = 8.7, 4H), 7.19–7.26 (m, 2H), 7.31–7.40 (m, 3H), 7.55 (s, 1H); 13C NMR (CDCl3) δ 14.60, 22.38, 44.70, 53.89, 60.19, 115.05, 123.25, 127.81, 128.47, 128.80, 128.87, 134.61, 135.45, 144.57, 158.25, 170.66; MS m/z 350 (M+, 21), 309 (11), 308 (50), 109 (14), 108 (17), 91 (100), 65 (15). HRMS (EI) m/z Calcd for C20H22N4O2: 350.1743. Found: 350.1733.
ACKNOWLEDGEMENTS
This work was generously supported by the Spanish Ministry of Science and Innovation (MICINN; CTQ2007-65218 and Consolider Ingenio 2010-CSD2007-00006), the Generalitat Valenciana (GV; PROMETEO/2009/039), and FEDER. Y. M. thanks the ISO of the University of Alicante for a postdoctoral grant.
References
1. For recent reviews, see for instance: A. Pace and P. Pierro, Org. Biomol. Chem., 2009, 7, 4337 (oxadiazoles); CrossRef M. S. Kedar, N. S. Dighe, S. R. Pattan, D. S. Musmade, D. Thakur, M. Bhosale, and V. M. Gaware, Pharma Chemica, 2010, 2, 249 (benzimidazoles); G. Palmisano, A. Penoni, M. Sisti, F. Tibiletti, S. Tollari, and K. M. Nicholas, Curr. Org. Chem., 2010, 14, 2409 (indoles); CrossRef R. Mishra and I. Tomar, Int. J. Pharm. Sci. Res., 2011, 2, 758 (pyrimidines); A. Schmidt and A. Dreger, Curr. Org. Chem., 2011, 15, 1423 (pyrazoles). CrossRef
2. For recent reviews, see for instance: C. Nájera and J. M. Sansano, Org. Biomol. Chem., 2009, 7, 4567; P. P. Gadhave, N. S. Dighe, S. R. Pattan, P. Deotarse, D. S. Musmade, and R. V. Shete, Ann. Biol. Res., 2010, 1, 82; I. Pibiri and S. Buscemi, Curr. Bioact. Comp., 2010, 6, 208. CrossRef
3. For a review, see: H. C. Kolb, M. G. Finn, and K. B. Sharpless, Angew. Chem. Int. Ed., 2001, 40, 2004. CrossRef
4. For recent reviews and monographs, see: A. D. Moorhouse and J. E. Moses, ChemMedChem, 2008, 3, 715; CrossRef J.-F. Lutz and Z. Zarafshani, Adv. Drug Delivery Rev., 2008, 60, 958; CrossRef 'Click Chemistry for Biotechnology and Materials Science,' ed. by J. Lahann, John Wiley & Sons, Hoboken, 2009; 'Applications of click chemistry themed issue', ed. by M. G. Finn and V. Fokin, Chem. Soc. Rev., 2010, 39, issue nº 4.
5. R. Huisgen, G. Szeimies, and L. Moebius, Chem. Ber., 1965, 98, 4014; CrossRef R. Huisgen, Pure Appl. Chem., 1989, 61, 613. CrossRef
6. C. W. Tornøe, C. Christensen, and M. Meldal, J. Org. Chem., 2002, 67, 3057.
7. V. V. Rostovtsev, L. G. Green, V. V. Fokin, and K. B. Sharpless, Angew. Chem. Int. Ed., 2002, 41, 2596. CrossRef
8. For reviews, see: V. D. Bock, H. Hiemstra, and J. H. van Maarseveen, Eur. J. Org. Chem., 2006, 51; CrossRef M. Meldal and C. W. Tornøe, Chem. Rev., 2008, 108, 2952; CrossRef P. Appukkuttan and E. Van der Eycken, Eur. J. Org. Chem., 2008, 1133; CrossRef C. O. Kappe and E. Van der Eycken, Chem. Soc. Rev., 2010, 39, 1280; CrossRef M. G. Finn and V. V. Fokin, 'Catalysis Without Precious Metals,' ed. by R. Morris Bullock, Wiley-VCH, Weinheim, 2010, pp. 235–26.
9. For reviews, see: F. Alonso, G. Radivoy, and M. Yus, Russ. Chem. Bull., Int. Ed., 2003, 52, 2563; F. Alonso and M. Yus, Chem. Soc. Rev., 2004, 33, 284; CrossRef F. Alonso and M. Yus, Pure Appl. Chem., 2008, 80, 1005; CrossRef F. Alonso, P. Riente, and M. Yus, Acc. Chem. Res., 2011, 44, 379. CrossRef
10. F. Alonso, C. Vitale, G. Radivoy, and M. Yus, Synthesis, 2003, 443; CrossRef F. Alonso, Y. Moglie, G. Radivoy, C. Vitale, and M. Yus, Appl. Catal. A: Gen., 2004, 271, 171; CrossRef G. Radivoy, F. Alonso, Y. Moglie, C. Vitale, and M. Yus, Tetrahedron, 2005, 61, 3859. CrossRef
11. F. Alonso, Y. Moglie, G. Radivoy, and M. Yus, Tetrahedron Lett., 2009, 50, 2358; CrossRef F. Alonso, Y. Moglie, G. Radivoy, and M. Yus, Eur. J. Org. Chem., 2010, 1875. CrossRef
12. For a recent contribution, see: F. Alonso, T. Melkonian, Y. Moglie, and M. Yus, Eur. J. Org. Chem., 2011, 2524. CrossRef
13. For recent reviews on the multicomponent synthesis of heterocycles, see: B. A. Arndtsen, Chem. Eur. J., 2009, 15, 302; CrossRef I. Akritopoulou-Zanze and S. W. Djuric, Top. Heterocycl. Chem., 2010, 25, 231; CrossRef B. Jiang, T. Rajale, W. Wever, S.-J. Tu, and G. Li, Chem. Asian J., 2010, 5, 2318. CrossRef
14. F. Alonso, Y. Moglie, G. Radivoy, and M. Yus, Adv. Synth. Catal., 2010, 352, 3208; CrossRef F. Alonso, Y. Moglie, G. Radivoy, and M. Yus, Org. Biomol. Chem., 2011, 9, 6385; CrossRef F. Alonso, Y. Moglie, G. Radivoy, and M. Yus, J. Org. Chem., in press, doi: org/10.1021/jo2016339. CrossRef
15. I. A. Khan and E. A. Abourashed, 'Leung’s Encyclopedia of Common Natural Ingredients', 3rd edn., John Wiley & Sons, Hoboken, 2010, pp. 443–448.
16. A. Slominski, Am. J. Physiol. Cell Physiol., 2008, 295, C293. CrossRef
17. 'The Merck Index', 13th edn., Merck & Co., Inc., Whitehouse Station, NJ, USA, 2001, p. 5353.
18. For reviews, see: Y. L. Angell and K. Burgess, Chem. Soc. Rev., 2007, 36, 1674; CrossRef K. A. Kalesh, H. Shi, J. Ge, and S. Q. Yao, Org. Biomol. Chem., 2010, 8, 1749. CrossRef
19. For a review, see: W. J. Malaisse, Int. J. Mol. Med., 1998, 2, 383.
20. For reviews, see: S. Dedola, S. A. Nepododiev, and R. A. Field, Org. Biomol. Chem., 2007, 5, 1006; CrossRef A. Dondoni, Chem. Asian J., 2007, 2, 700; CrossRef A. Dondoni, Org. Biomol. Chem., 2010, 8, 3366; CrossRef V. Aragão-Leoneti, V. L. Campo, A. S. Gomes, R. A. Field, and I. Carvalho, Tetrahedron, 2010, 66, 9475. CrossRef
21. E. van Dijkum, R. Danac, D. J. Hughes, R. Wood, A. Rees, B. L. Wilkinson, and A. J. Fairbanks, Org. Biomol. Chem., 2009, 7, 1097. CrossRef
22. R. Kumar, P. R. Maulik, and A. K. Misra, Glycoconj. J., 2008, 25, 595. CrossRef
23. The Merck Index', 13th edn., Merck & Co., Inc., Whitehouse Station, NJ, USA, 2001, p. 3746.
24. J. Matthews, 'Endogenous Toxins: Targets for Disease Treatment and Prevention,' Vol. 1, ed. by P. O'Brien and R. W. Bruce, Wiley-VCH, Weinheim, 2010, pp. 227–248.
25. The Merck Index', 13th edn., Merck & Co., Inc., Whitehouse Station, NJ, USA, 2001, p. 2223.
26. For a recent, review, see: G. Saher, S. Quintes, and K.-A. Nave, Neuroscientist, 2011, 17, 79. CrossRef
27. O. Kaczmarek, H. A. Scheidt, A. Bunge, D. Föse, S. Karsten, A. Arbuzova, D. Huster, and J. Liebscher, Eur. J. Org. Chem., 2010, 1579. CrossRef
28. For a review on antipyretic therapy including phenacetin, see: R. Botting, Front. Biosci., 2004, 9, 956. CrossRef
29. 'Ninth Report on Carcinogens,' PB2000-107509, 2000, pp. III-4, III-180.
30. W. F. J. Hogendorf, C. P. Verhagen, E. Malta, N. Goosen, H. S. Overkleeft, D. V. Filippov, and G. A. Van der Marel, Tetrahedron, 2009, 65, 10430. CrossRef
31. Z. Hakki, B. Cao, A. M. Heskes, J. Q. D. Goodger, I. E. Woodrow, and S. J. Williams, Carbohydr. Res., 2010, 345, 2079. CrossRef
32. M. Ohkubo, S. Mochizuki, T. Sano, Y. Kawaguchi, and S. Okamoto, Org. Lett., 2007, 9, 773. CrossRef