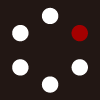
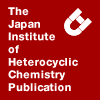
HETEROCYCLES
An International Journal for Reviews and Communications in Heterocyclic ChemistryWeb Edition ISSN: 1881-0942
Published online by The Japan Institute of Heterocyclic Chemistry
e-Journal
Full Text HTML
Received, 30th June, 2011, Accepted, 1st August, 2011, Published online, 11th August, 2011.
DOI: 10.3987/COM-11-S(P)64
■ Synthesis of Ruthenium(II) 2,6-Bis(imino)pyridyl Complexes for C-H Amination of Sulfamate Esters
Jennifer L. Bon and Simon B. Blakey*
Department of Chemistry, Emory University, 1515 Dickey Drive, Atlanta, GA 30322, U.S.A.
Abstract
A family of ruthenium(II) 2,6-bis(imino)pyridyl complexes have been developed as a novel catalyst framework for C-H amination. Their synthesis and evaluation is herein described. The reactivity of these catalysts is heavily dependent upon the electronics of the ligand. These complexes are capable of functionalizing the benzylic protons of sulfamate esters with good conversion.C-H amination has emerged as an attractive strategy for the synthesis of complex nitrogen containing heterocycles.1-6 Despite recent advances in this area, many challenges remain, particularly with respect to substrate generality and intermolecular amination. In an attempt to address these challenges, our group has focused on the development of novel catalyst frameworks for these reactions.7 We have previously established the efficacy of ruthenium(II) pyridinebisoxazoline (pybox) complexes for the formation of carbon-nitrogen bonds and were intrigued by the role of the ligand in the transformation. 2,6-Bis(imino)pyridyl ligands are isoelectronic with pybox ligands, but the ligands themselves have been shown to participate in redox events and therefore may help stabilize the reactive imido intermediate.8 While a small number of ruthenium(II) 2,6-bis(imino)pyridyl complexes are known,9-11 their potential for C-H amination has not been reported. Herein we report the synthesis and evaluation of a family of ruthenium(II) 2,6-bis(imino)pyridyl complexes as new catalysts for amination of benzylic C-H bonds.
A series of 2,6-bis(imino)pyridyl ligands were synthesized to study the effect of both steric and electronic variations around the aniline ring on the catalysts activity towards C-H amination. All ligands were generated from the condensation of 2,6-diacetylpyridine with the corresponding commercially available aniline.12 Ligands lacking ortho substitution (1, 4, and 5), as well as ligands with methyl (2) and isopropyl (3) substituents at both ortho positions were all prepared to give three distinct levels of steric inhibition around the metal. To study the effect of the electronic environment, the p-methoxy (4), and p-fluoro (5) derivatives were also synthesized (Figure 1).
The bis(imino)pyridyl ligands were then metalated in refluxing dichloromethane over 8 hours using either dichloro(p-cymene)ruthenium(II) dimer or di-µ-bromobis[(benzene)bromoruthenium(II)] (Figure 2). The two more sterically congested ligands (2 and 3) required higher temperature and pressure, and longer reaction time to ensure metalation. In addition to investigating electronic and steric variation on the bisiminopyridyl ligand (6, 10-13), we synthesized complexes from both the chloride (6) and bromide (7) Ru(II) salts, and with a variety of ancillary ligands (CO, ethylene (8), and MeCN (9)) around the metal. Complexes were generally obtained in good yields and were easily isolated by recrystallization from dichloromethane/ether.
With these new catalysts in hand, they were then tested for their reactivity towards C-H amination using sulfamate ester 14 under conditions previously found to be successful in other Ru(II) catalyst systems (Table 1).7 Exchanging chloride ligands for bromide ligands was found to have little effect on the C-H amination reaction (26% versus 24% conversion, entries 1 and 2). Using acetonitrile as the ancillary ligand (32%) led to a small improvement with respect to ethylene (27%) and CO (24%). Substitution at the ortho position of the aniline ring was detrimental to conversion (entries 5 and 6). The biggest improvements in catalytic activity were found with the electronic variations to the aniline ring; an electron withdrawing group at the para position gave significantly improved conversion (48%, entry 8), while an electron donating group at the same position led to only 16% conversion (entry 7).
Based on these results, we hypothesized that a more reactive catalyst would include both the electron withdrawing bisimino ligand and the acetonitrile ancillary ligand, and as such, catalyst 16 was prepared (Equation 1).
Catalyst 16 was tested in conjunction with a series of solvents (Table 2). Non-polar and ethereal solvents effectively shut down the reaction (entries 2-4). Trifluorotoluene gave the best conversion at 51%. However, in dichloromethane 16 gave a slightly lower conversion than 13 had previously. This led us to test 13 in trifluorotoluene, and we found that it gave significantly better conversion (73%, entry 8) than 16 (51%, entry 7). These results suggests that there is a complex interplay between bisiminopyridyl ligand electronics and ancillary ligands, and that their effects are not merely additive. Catalyst 13 was therefore used in subsequent optimization studies.
As electronics were observed to have the largest influence on conversion, with electron withdrawing substitutents leading to superior catalysts, the p-nitro derivative of the catalyst was prepared (17, Scheme 1). Again, 2,6-bis[1-(4-nitrophenylimino)ethyl]pyridine was generated from the p-nitroaniline and 2,6-diacetylpyridine. Due to the strongly electron withdrawing nature of the nitro group, the synthesis required forcing conditions (140 oC, 5 days) and had a much lower yield (10%). Fortunately metalation proceeded under standard conditions.
Catalyst 17 was tested using the previously described conditions (Table 3). However, it gave a lower conversion than 13 (35% versus 73%) suggesting that there may be a limit to which electron with drawing groups on the aniline ring are beneficial (Table 3, entry 1).
In further optimization of the amination reaction, the role of the additives was briefly examined (Table 3). MgO was found to improve conversion but not necessary for the reaction to proceed, as the reaction still gave 56% conversion without it (entry 2). The identity of the oxidant seemed to have a greater impact. The conversion dropped upon switching from iodobenzene diacetate to iodobenzene dipivalate and dropped even further with iodobenzene bis(triphenylacetate) (entries 3-5).13 Increasing the equivalents of iodobenzene diacetate from 1.1 to 2.0 also negatively affected conversions (entry 6).
In conclusion, we have synthesized a family of ruthenium(II) bisimino complexes which are effective for the amination of C-H bonds of sulfamate esters. Electron withdrawing groups in the para position of the ligand’s aniline ring gave the best conversions, while electron donating groups and sterically demanding groups inhibit the reaction.
EXPERIMENTAL
General Information. All reactions were carried out under an atmosphere of nitrogen unless otherwise stated. All chemicals were purchased from Aldrich Chemical Company and used without further purification unless otherwise stated. Anhydrous solvents were purified by passage through activated alumina using a Glass Contours solvent purification system unless otherwise noted. Trifluorotoluene was dried over activated molecular sieves. Solvents used in workup, extraction and column chromatography were used as received from commercial suppliers without prior purification. Analytical thin layer chromatography (TLC) was performed on precoated glass backed EMD 0.25 mm silica gel 60 plates. Visualization was accomplished with UV light or KMnO4. Flash column chromatography was carried out using Silicycle Silia Flash® F60 silica gel (40-63 μm). High resolution mass spectra were obtained using a Thermo Electron Corporation Finigan LTQFTMS (at the Mass Spectrometry Facility, Emory University). 1H and 13C NMR spectra were obtained on a Varian Inova 600 spectrometer (600 MHz 1H, 150 MHz 13C), a Varian Inova 400 spectrometer (400 MHz 1H, 100 MHz 13C), or a Unity 400 spectrometer (400 MHz 1H, 100 MHz 13C) at room temperature in CDCl3 with internal CHCl3 as the reference (7.26 ppm for 1H and 77.23 ppm for 13C), unless otherwise stated. Chemical shifts (δ values) were reported in parts per million (ppm) and coupling constants (J values) in Hz. Infrared (IR) spectra were recorded using Thermo Electron Corporation Nicolet 380 FT-IR spectrometer.
General procedure for the synthesis of bisimino ligands:12
A round bottomed flask was charged with the aniline (3 equiv), 2,6-diacetylpyridine (1 equiv), formic acid (5 mol %), and MeOH (0.8 molar). The reaction mixture was refluxed overnight (14 h). The yellow solid was purified by recrystallization from hot MeOH to afford the corresponding bisimino ligand.
2,6-Bis(1-phenyliminoethyl)pyridine (1)
Prepared according to the general procedure from aniline (3.4 g, 36 mmol) and 2,6-diacetylpyridine (2.0 g, 12 mmol) in MeOH gave 1 as a yellow solid, (1.11 g, 29%). The spectroscopic data are identical to literature.12 1H NMR (400 MHz, CDCl3): 8.33 (d, 2H, J = 7.9 Hz), 7.87 (t, 1H, J = 7.9 Hz) 7.37 (t, 4H, J = 8.2 Hz), 7.11 (t, 2H, J = 7.3 Hz), 6.84 (d, 4H, J = 7.3 Hz), 2.39 (s, 6H).
2,6-Bis[1-(2,4,6-trimethylphenylimino)ethyl]pyridine (2)
Prepared according to the general procedure from 2,4,6-trimethylaniline (2.052 g, 15.2 mmol) and 2,6-diacetylpyridine (850 mg, 5.2 mmol) in MeOH gave 2 as a yellow solid, (1.32 g, 64%). The spectroscopic data are identical to literature.14 1H NMR (400 MHz, CDCl3): 8.49 (d, 2H, J = 7.9 Hz), 7.93 (t, 1H, J = 7.9 Hz), 7.17 (d, 4H, J = 7.0 Hz), 7.11 (t, 2H, J = 6.8 Hz), 2.77 (sep, 2H, J = 6.8 Hz), 2.27 (s, 6H), 1.16 (d, 12H, J = 6.7 Hz), 1.16 (d, 12H, J = 7.0 Hz).
2,6-Bis[1-(2,6-diisopropylphenylimino)ethyl]pyridine (3)
Prepared according to the general procedure from 2,6-diisopropylaniline (815 mg, 4.60 mmol) and 2,6-diacetylpyridine (250 mg, 1.53 mmol) in MeOH gave 3 as a solid, (608 mg, 92%). The spectroscopic data are identical to literature.12 1H NMR (400 MHz, CDCl3): 8.47 (d, 2H, J = 7.6 Hz), 7.91 (t, 1H, J = 7.8 Hz), 6.91 (s, 4H), 2.30 (s, 6H), 2.24 (s, 6H), 2.02 (s, 12H).
2,6-Bis[1-(4-methoxyphenylimino)ethyl]pyridine (4)
Prepared according to the general procedure from 4-methoxyaniline (453 mg, 3.86 mmol) and 2,6-diacetylpyridine (200 mg, 1.23 mmol) in MeOH gave 4 as a yellow solid, (334 mg, 72%). The spectroscopic data are identical to literature.15 1H NMR (400 MHz, CDCl3): 8.32 (d, 2H, J = 6.3 Hz), 7.78 (t, 1H, J = 7.8 Hz), 6.93 (d, 4H, J = 8.5 Hz), 6.82 (d, 4H, J = 8.8 Hz), 3.82 (s, 6H), 2.43 (s, 6H).
2,6-Bis[1-(4-fluorophenylimino)ethyl]pyridine (5)
Prepared according to the general procedure from 4-fluoroaniline (409 mg, 3.69 mmol) and 2,6-diacetylpyridine (200 mg, 1.23 mmol) in MeOH gave 5 as a yellow solid, (265 mg, 62%). The spectroscopic data are identical to literature.10 1H NMR (400 MHz, CDCl3): 8.31 (d, 2H, J = 7.8 Hz), 7.87 (t, 1H, J = 7.8 Hz), 7.14-6.69 (m, 4H), 6.88-6.75 (m, 4H), 2.40 (s, 6H).
2,6-Bis[1-(4-nitrophenylimino)ethyl]pyridine
2,6-Diacetylpyridine (1.18 g, 7.2 mmol) was added to 4-nitroaniline (3.00 g, 21.7 mmol, 3 equiv) in 50 mL of xylenes and 50 mg of p-TSA was added. The flask was fitted with a Dean-Stark trap and was refluxed for 5 days. The reaction mixture was concentrated to half its original volume and solid was collected to give the title compound as a yellow solid (284 mg, 10%). The spectroscopic data are identical to literature.16 1H NMR (400 MHz, CDCl3): 8.35 (d, 2H, J = 7.8 Hz), 8.27 (d, 4H, J = 8.6 Hz), 7.93 (t, 1H, J = 7.2 Hz), 6.93 (d, 4H, J = 9.0 Hz), 2.40 (s, 6H).
(2,6-Bis(1-phenyliminoethyl)pyridine)(carbonyl)dichlororuthenium(II) (6)
A 50 mL round bottomed flask was charged with bisimino ligand 1 (300 mg, 0.958 mmol) and dichloro(p-cymene)ruthenium(II) dimer (351 mg, 0.575 mmol). The flask was purged with CO and 20 mL of DCM (saturated with CO) was added. The reaction mixture was allowed to reflux under an atmosphere of CO for 8 h after which the solvent was removed under reduced pressure. The residue was dissolved in a minimum of DCM and recrystallized out of Et2O/DCM to give 6 as a brown solid (480mg, 98%). 1H NMR (400 MHz, CDCl3): 8.06 (t, 1H, J = 8.1 Hz), 7.88 (d, 2H, J = 8.1 Hz) 7.40 (t, 4H, J = 7.2 Hz), 7.28 (t, 2H, J = 5.1 Hz), 7.21 (d, 4H, J = 8.6 Hz), 2.56 (s, 6H). 13C NMR (100 MHz, CDCl3): 172.0, 157.5, 151.5, 129.6, 127.4, 124.05, 120.5, 18.4. IR (thin film, cm-1) ν = 1980, 1591, 1484, 1272. HRMS [- ESI] calculated for 511.98693, found 511.98759 [M-H+].
(2,6-Bis(1-phenyliminoethyl)pyridine)(carbonyl)dibromoruthenium(II) (7)
A 25 mL round bottomed flask was charged with bisimino ligand 1 (130 mg, 0.42 mmol) and di-µ-bromobis[(benzene)bromoruthenium(II)]7 (169 mg, 0.25 mmol, 0.6 equiv). The flask was purged with CO and 10 mL of CO purged DCM was added. The reaction mixture was allowed to reflux under an atmosphere of CO for 7 h after which the solvent was removed under reduced pressure. The residue was dissolved in a minimum of DCM and recrystallized from benzene/DCM. (162 mg, 64%). 1H NMR (400 MHz, CDCl3): 8.05 (t, 2H, J = 8.6 Hz), 7.91 (d, 1H, J = 8.2 Hz), 7.37 (t, 4H, J = 7.4 Hz), 7.22-7.27 (m, 6H), 2.54 (s, 6H). 13C NMR (100 MHz, CDCl3): 157.3, 151.6, 129.5, 128.6, 127.4, 124.0, 120.7, 94.9, 18.4 (C≡O shift not observed). IR (thin film, cm-1) ν = 2025, 1982, 1955, 1431. HRMS [- ESI] calculated for 601.88385, found 601.88420 [M-H+].
(2,6-Bis(1-phenyliminoethyl)pyridine)(dichloro)ethyleneruthenium(II) (8)
A 50 mL round bottomed flask was charged with bisimino ligand 1 (300 mg, 0.958 mmol) and dichloro(p-cymene)ruthenium(II) dimer (351 mg, 0.575 mmol). The flask was purged with ethylene and 24 mL of ethylene saturated DCM was added. The reaction mixture was allowed to reflux under an atmosphere of ethylene for 8 h after which the solvent was removed under reduced pressure. The residue was dissolved in a minimum of DCM and recrystallized from Et2O/DCM to give 8 as a deep red solid (301 mg, 57%). 1H NMR (400 MHz, CDCl3): 8.01 (d, 2H, J = 7.8 Hz), 7.89 (t, 1H, J = 7.8 Hz), 7.41 (t, 4H, J = 7.4 Hz), 7.30 (t, 2H, J = 7.0 Hz), 7.03 (d, 4H, J = 7.4 Hz), 4.23 (s, 4H), 2.67 (s, 6H). 13C NMR (100 MHz, CDCl3): 173.2, 157.4, 150.9, 132.0, 129.1, 126.6, 124.1, 121.2, 86.5, 19.2. IR (thin film, cm-1) ν = 1590, 1481, 1448, 1313. HRMS [- ESI] calculated 519.96881, found 519.96953 [M - ethylene + Cl-].
Acetonitrile(2,6-bis(1-phenyliminoethyl)pyridine)dichlororuthenium(II) (9)
A 25 mL round bottomed flask was charged with bisimino ligand 1 (135 mg, 0.432 mmol) and dichloro(p-cymene)ruthenium(II) dimer (158 mg, 0.259 mmol). Degassed DCM (20 mL) was added. The reaction mixture was allowed to reflux under an atmosphere of N2 for 8 h after which the solvent was removed under reduced pressure. The residue was dissolved in a minimum of 1:1 MeCN/DCM solution and recrystallized from Et2O/DCM to give 9 as a deep purple solid (143 mg, 63%). The spectroscopic data are identical to literature.10 1H NMR (400 MHz, CDCl3): 7.79 (d, 2H, J = 7.8 Hz), 7.57 (t, 1H, J = 7.8 Hz) 7.43-7.28 (m, 6H), 7.28-7.20 (m, 4H), 2.72 (s, 6H), 1.99 (s, 3H).
(2,6-Bis[1-(2,4,6-trimethylphenylimino)ethyl]pyridine)(carbonyl)dichlororuthenium(II) (10)
A 50 mL Schlenk tube was charged with bisimino ligand 2 (150 mg, 0.42 mmol) and di-µ-bromobis[(benzene)bromoruthenium(II)] (169 mg, 0.25 mmol, 0.6 equiv). THF (8 mL) was added and the flask was sealed under an atmosphere of N2. The reaction mixture was allowed to reflux for 5 days after which the reaction mixture was transferred to a round bottomed flask and stirred under an atmosphere of CO for 4 h at room temperature. The solvent was then removed under reduced pressure and the residue dissolved in a minimum of DCM and recrystallized from Et2O/DCM. 286 mg, 83%. The spectroscopic data are identical to literature.17 1H NMR (400 MHz, CDCl3): 8.09 (t, 1H, J = 8.1 Hz), 7.93 (d, 1H, J = 8.1 Hz), 6.86 (s, 4H), 2.47 (s, 6H), 2.26 (s, 12H), 2.25 (s, 6H).
(2,6-Bis[1-(2,6-diisopropylphenylimino)ethyl]pyridine)(carbonyl)dichlororuthenium(II) (11)
A 50 mL Schlenk tube was charged with bisimino ligand 3 (60 mg, 0.13 mmol) and di-µ-bromobis[(benzene)bromoruthenium(II)] (46 mg, 0.075 mmol, 0.6 equiv). THF (6 mL) was added and the flask was sealed under a stream of N2. The reaction mixture was allowed to reflux for 4 days after which the reaction mixture was transferred to a round bottomed flask and stirred under an atmosphere of CO for 6 h at room temperature. The solvent was then removed under reduced pressure and the residue dissolved in a minimum of DCM and recrystallized from Et2O/DCM. 18 mg, 11%. The spectroscopic data are identical to literature.17 1H NMR (400 MHz, CDCl3): 8.12 (t, 1H, J = 8.0 Hz), 7.94 (d, 2H, J = 6.8 Hz), 7.37-7.10 (m, 6H), 3.35 (m, 4H), 2.50 (s, 6H), 1.25 (d, 12H, J = 6.7 Hz), 0.96 (d, 12H, J = 6.7).
(2,6-Bis[1-(4-methoxyphenylimino)ethyl]pyridine)(carbonyl)dichlororuthenium(II) (12)
A 25 mL round bottomed flask was charged with bisimino ligand 4 (100 mg, 0.268 mmol) and di-µ-bromobis[(benzene)bromoruthenium(II)] (98 mg, 0.161 mmol, 0.6 equiv). The flask was purged with CO and 10 mL of CO purged DCM were added. The reaction mixture was allowed to reflux under an atmosphere of CO for 8 h after which the solvent was removed under reduced pressure. The residue was dissolved in a minimum of DCM and recrystallized from benzene/DCM. 142 mg, 93%. 1H NMR (400 MHz, CDCl3): 8.03 (t, 1H, J = 8.1 Hz), 7.84 (d, 2H, J = 8.1 Hz), 7.17 (d, 4H, J = 9.1 Hz), 6.90 (d, 4H, J = 9.1 Hz), 3.83 (s, 6H), 2.57 (s, 6H). 13C NMR (100 MHz, CDCl3): 171.6, 158.7, 157.6, 145.0, 138.1, 123.8, 122.2, 114.5, 55.7, 18.4, (C≡O shift not observed). IR (thin film, cm-1) ν = 1956, 1603, 1502, 1249. HRMS [- ESI] calculated 572.00817, found 572.00852 [M-H+].
(2,6-Bis[1-(4-fluorophenylimino)ethyl]pyridine)(carbonyl)dichlororuthenium(II) (13)
A 25 mL round bottomed flask was charged with bisimino ligand 5 (100 mg, 0.287 mmol) and di-µ-bromobis[(benzene)bromoruthenium(II)] (105 mg, 0.172 mmol, 0.6 equiv). The flask was purged with CO and 10 mL of CO purged DCM were added. The reaction mixture was allowed to reflux under an atmosphere of CO for 7 h after which the solvent was removed under reduced pressure. The residue was dissolved in a minimum of DCM and recrystallized from benzene/DCM. 157 mg, 73%. 1H NMR (400 MHz, CDCl3): 8.07 (t, 1H, J = 8.5 Hz), 7.89 (d, 2H, J = 7.93 Hz) 7.18 (dd, 4H, J = 8.9, 4.8 Hz), 7.08 (t, 4H, J = 8.70 Hz), 2.55 (s, 6H). 13C NMR (100 MHz, CDCl3): 199.8, 172.4, 162.6, 157.2, 147.3, 138.1, 124.0, 122.1, 116.2, 18.1. IR (thin film, cm-1) ν = 1954, 1499, 1215. HRMS [- ESI] calculated 547.96820, found 547.96840 [M-H+].
Acetonitrile(2,6-Bis[1-(4-fluorophenylimino)ethyl]pyridine)dichlororuthenium(II) (16)
Procedure adapted from the literature.10 A 25 mL round bottomed flask was charged with bisimino ligand 5 (160 mg, 0.472 mmol) and dichloro(p-cymene)ruthenium(II) dimer (173 mg, 0.283 mmol, 0.6 equiv). Degassed DCM (20 mL) was added. The reaction mixture was allowed to reflux under an atmosphere of N2 for 7 h after which the solvent was removed under reduced pressure. The residue was dissolved in a minimum of 1:1 MeCN/DCM solution and recrystallized from Et2O. 226 mg, 85%. 1H NMR (400 MHz, CDCl3): 7.81 (d, 2H, J = 7.9 Hz), 7.59 (t, 1H, J = 7.9 Hz), 7.31 (dd, 4H, J = 8.8, 4.9 Hz), 7.06 (t, 4H, J = 8.7 Hz), 2.72 (s, 6Hz), 2.10 (s, 3H). 13C NMR (100 MHz, CDCl3): 170.8, 163.0, 159.7, 146.0, 127.9, 125.6, 123.8, 122.7, 115.3, 17.4, 3.6. IR (thin film, cm-1) ν = 1496, 1391, 1211, 1092. HRMS [- ESI] calculated 555.94996, found 555.95039 [M-H+].
(2,6-Bis[1-(4-nitrophenylimino)ethyl]pyridine)(carbonyl)dichlororuthenium(II) (17)
A 50 mL round bottomed flask was charged with 2,6-bis(1[4-nitrophenylimino]ethyl)pyridine (200 mg, 0.496 mmol) and dichloro(p-cymene)ruthenium(II) dimer (182 mg, 0.298 mmol, 0.6 equiv). The flask was purged with CO and 20 mL of CO purged DCM was added. The reaction mixture was allowed to reflux under an atmosphere of CO for 16 h after which the solvent was removed under reduced pressure. The residue was dissolved in a minimum of DCM and recrystallized from benzene/DCM. 61 mg, 34%. 1H NMR (400 MHz, CDCl3): 8.32 (d, 4H, J = 8.6 Hz), 8.17 (t, 1H, J = 8.1 Hz), 8.01 (d, 2H, J = 8.1 Hz), 7.36 (d, 4H, J = 9.1 Hz), 2.61 (s, 6H). IR (thin film, cm-1) ν = 1974, 1588, 1522, 1311. HRMS [- ESI] calculated 601.95720, found 601.95742 [M-H+].
General Procedure for the cyclization of phenyl sulfamate ester:
Oxathiazinane (15)
A 7 mL vial was charges with phenyl sulfamate ester 14 (17.5 mg, 0.08 mmol), catalyst (0.004 mmol, 0.05 equiv), additive (7.4 mg, 0.18 mmol, 2.3 equiv), and oxidant (0.088 mmol, 1.1 equiv). The vial was purged with nitrogen and 1 mL (0.08M) of dry solvent was added. The suspension was stirred at room temperature for 24 h and then quenched with 1 mL of MeOH. The reaction mixture was filtered through a celite plug, the plug was washed with DCM (3 x 5 mL) and EtOAc (3 x 5 mL). The conversion was then determined by GC. The spectroscopic data are identical to literature.7 1H NMR (400 MHz, CDCl3): 7.30-7.43 (m, 4H), 4.78-4.92 (m, 2H), 4.65 (d, 2H, J = 11.6, 4.9, 1.5 Hz), 4.21 (d, 1H, J = 8.9 Hz), 2.24 (dtd, 1H, J = 14.5, 12.6, 4.9 Hz), 2.03 (dq, 1H, J = 14.7, 2.2 Hz). GC (CHIRASIL-DEX CB column) tr = 16.6 min.
Phenyliodine Bis(triphenylacetate)
Phenyliodine Bis(triphenylacetate) was made according to the literature procedure.18 Phenyliodine diacetate (322 mg, 1 mmol) was added to a mixture of triphenylacetic acid (576 mg, 2.0 mmol) in 15 mL diethylether. The reaction mixture was stirred at room temperature for 6 h. The mixture was then filtered and the white solid recrystallized from CHCl3 and Et2O. 538 mg, 75%. IR (thin film, cm-1) ν = 1645, 1223, 1192. HRMS [+ ESI] calculated 801.14777, found 801.14614 [M+Na+].
References
1. F. Collet, R. H. Dodd, and P. Dauban, Chem. Commun., 2009, 5061. CrossRef
2. F. Collet, C. Lescot, and P. Dauban, Chem. Soc. Rev., 2011, 40, 1926. CrossRef
3. H. J. Lu and X. P. Zhang, Chem. Soc. Rev., 2011, 40, 1899. CrossRef
4. T. G. Driver, Org. Biomol. Chem., 2010, 8, 3831. CrossRef
5. H. M. L. Davies, and M. S. Long, Angew. Chem. Int. Ed., 2005, 44, 3518. CrossRef
6. J. Du Bois, Org. Proces. Res. Dev., 2011, DOI: 10.1021/op200046v. CrossRef
7. E. Milczek, N. Boudet, and S. Blakey, Angew. Chem. Int. Ed., 2008, 47, 6825. CrossRef
8. V. C. Gibson, C. Redshaw, and G. A. Solan, Chem. Rev., 2007, 107, 1745. CrossRef
9. M. Gallagher, N. L. Wieder, V. K. Dioumaev, P. J. Carroll, and D. H. Berry, Organometallics, 2010, 29, 591. CrossRef
10. O. Dayan and B. Cetinkaya, J. Mol. Cat. A - Chem., 2007, 271, 134.
11. C. Bianchini, H. M. Lee, Organometallics, 2000, 19, 1833. CrossRef
12. R.-Q. Fan, D.-S. Zhu, Y. Mu, G.-H. Li, Y.-L. Yang, Q. Su, and S.-H. Feng, Eur. J. Inorg. Chem., 2004, 24, 4891. CrossRef
13. D. N. Zalatan and J. Du Bois, J. Am. Chem. Soc., 2009, 131, 7558. CrossRef
14. O. Dayan, F. Dogan, I. Kaya, and B. Cetinkaya, Synth. React. Inorg. M., 2010, 40, 337.
15. A. L. Vance, N. W. Alcock, J. A. Heppert, and D. H. Busch, Inorg. Chem., 1998, 37, 6912. CrossRef
16. A. S. Ionkin, W. J. Marshall, D. J. Adelman, A. L. Shoe, R. E. Spence, and T. Y. Xie, J. Polym. Sci. Pol. Chem., 2006, 44, 2615. CrossRef
17. N. L. Wieder, P. J. Carroll, and D. H. Berry, Organometallics, 2011, 30, 2125. CrossRef
18. J. E. Leffler, D. C. Ward, and A. Burdurog, J. Am. Chem. Soc., 1972, 94, 5339. CrossRef