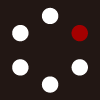
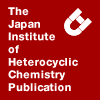
HETEROCYCLES
An International Journal for Reviews and Communications in Heterocyclic ChemistryWeb Edition ISSN: 1881-0942
Published online by The Japan Institute of Heterocyclic Chemistry
e-Journal
Full Text HTML
Received, 10th May, 2011, Accepted, 6th June, 2011, Published online, 16th June, 2011.
DOI: 10.3987/COM-11-S(P)21
■ Synthesis of Octahydroquinolizinones Aza-Analogues of Terpenoid Skeletons
Alberto Brandi,* Franca M. Cordero, Carolina Vurchio, Massimo Lucentini, and Stefano Cicchi
Department of Chemistry "Ugo Schiff", University of Florence, Via della Lastruccia 13, 50019 Sesto Fiorentino (FI), Italy
Abstract
Two new ketones, 6,6,9a-trimethyl-octahydro-quinolizin-2-one and 4,4,9a-trimethyl-octahydro-quinolizin-2-one, were synthesized by regioselective 1,3-dipolar cycloaddition of tetrahydropyridine nitrones with methylenecyclopropanes followed by thermal rearrangement. The new ketones feature a structural analogy with the 5,5,8a-trimethyl-octahydro-2-naphthalene skeleton typical of terpenoid structures.The replacement of a carbon or oxygen atom in a natural compound by a nitrogen often goes along with a selective biological activity, that in many cases has been used for the set up of new classes of bioactive molecules. The finding of the peculiar bioactivity of azasteroids,1 or of iminosugars2 is a fair example of the importance of the aza–analogues in the discovery of new drugs, or pharmacological tools. The replacement of an sp3 carbon with a nitrogen atom is likely to induce higher flexibility in the molecule and modify its hydrophilic character and acid/base profile.
Our group is contributing to this research field since many years with the development of strategies for the stereoselective synthesis of such compounds, for ex. iminosugars,3 or with the discovery of new synthetic aza-analogues of natural products showing a similar activity, like aza-illudines.4
The 1,1,4a-trimethyl-octahydronaphthalene skeleton 1 (Figure 1) is present in natural products like labdane terpenoids characterized by an important biological activity,5 and is an interesting possible target for this kind of modification. We now report the synthesis of new ketones with trimethyl-octahydroquinolizin-2-one structure 2 and 3 which can be considered aza-analogues of terpenoid ketones, and can be the precursors of more complex compounds containing the 5-aza-octahydronaphtalene skeleton.
The ketones 2 and 3 can be straightforwardly derived from the [3+2]cycloaddition–rearrangement strategy developed in our group6 starting from tetrahydropyridine-N-oxides 4 and 6 and methylene cyclopropanes 5 and 7, respectively (Scheme 1).
The two strategies, that differ in the placement of the gem-dimethyl group on the nitrone moiety or on the methylenecyclopropane moiety, bring about experimental differences that will be discussed below.
Nitrone 6 is a known compound synthesized by oxidation of 2-methylpiperidine.7 Nitrone 4 can be easily synthesized from the known nitrone 87 by sequential methylation with MeMgCl and oxidation of the formed hydroxylamine with MnO2 (Scheme 2).
The new nitrone 4, at a first glance, is characterized by a clear steric hindrance exerted by the methyl groups around the dipole moiety that could hamper the approach of a dipolarophile. In fact, the cycloaddition with the sluggish methylenecyclopropane,6c carried out in a sealed vial along one week at 100 °C, gave, after flash chromatography, the pure isoxazolidine 10 as a sole regioisomer in only 28% yield (Scheme 3). The observed low yield is a possible consequence of the low stability of the dipole at the relative high temperatures necessary to induce the cycloaddition. Actually, apart from the adduct, only unidentified decomposition products from the nitrone were observed in the crude reaction mixture.8
The complete regioselectivity observed in the cycloaddition to methylenecyclopropane6 is well explained with the presence of the 2-methyl group on nitrone 4 which exerts a higher steric hindrance on the transition state leading to the 3’-spirocyclopropane regioisomer. As expected, a slightly better reactivity was observed with nitrone 6, which gave with methylenecyclopropane 7,9 under the same reaction conditions, the isoxazolidines 11a,b in 36% isolated yield as an equimolar mixture of diastereomers (Scheme 3). The formation of a mixture of diastereomers originates from the substitution of the methylenecyclopropane which undergoes two diastereomeric approaches to the dipole, but it does not affect the synthesis of the final product as both the diastereoisomers will give the same diradical intermediate in the rearrangement step.6 As in the previous case, the regioselectivity was complete in favor of the 3’-spiro fused adduct. The final picture of these cycloaddition reactions is that six-membered cyclic nitrones as 4 and 6 show a lower reactivity compared to their 5-membered counterparts.6,8
The stability of the isoxazolidines 10 and 11 is also higher than that generally observed for the counterparts derived from 5-membered nitrones, which rearrange in a range of temperature 100-150 °C. Therefore the rearrangement of 10 and 11 was carried out under FVT conditions, in standard equipment in a horizontal assembly connected with a cold trap,10 by distillation of the isoxazolidines at 10-2 mmHg through a hot quartz tube heated at 450 °C (Scheme 4). The vapors of the rearranged products, having a lower volatility than the starting materials, condense in the tube just outside of the oven causing partial decomposition of the products.
However, the rearrangement was highly selective,6 as the sole rearrangement products 2 and 3 were isolated, after short flash chromatography, in 35% and 39% not optimized yields, respectively. The rearrangement is likely to occur via a diradical intermediate11 (12 and 13, respectively) originated by the homolytic cleavage of the N-O bond followed by the opening of the cyclopropyloxy radical.
In conclusion, a synthesis of two new octahydroquinolizidine ketones has been carried out applying the 1,3-dipolar cycloaddition-thermal rearrangement strategy from tetrahydropyridine nitrones and methylenecyclopropanes. The process is characterized by moderate yields of isolated products that require further optimization, but by high selectivity both in the cycloaddition and the rearrangement step. These new ketones represent 4a-aza-analogues of the octahydronaphtalene skeleton found in many bioactive products of terpenoid origin, and suggest their exploitation for the synthesis of more complex terpenoid structures containing nitrogen instead of carbon at the bridgehead position.
EXPERIMENTAL
IR spectra were determined with a Perkin Elmer 881 spectrophotometer. The NMR spectra were determined in CDCl3 with a Varian Gemini FT NMR spectrometer operating at 200 MHz (1H) and 50 MHz (13C) and a Varian INOVA FT NMR spectrometer operating at 400 MHz (1H) and 100 MHz (13C). MS spectra (EI, 70 eV) were measured by 5792A Hewlett-Packard and QMD 1000 Carlo Erba instruments. Column chromatography was performed using Merck Kieselgel 60 (0.063–0.200 mm). Microanalyses were carried out with a Perkin-Elmer 240 C instrument. All of the organic solvents used in this study were dried over appropriate drying agents and distilled prior to use.
2,6,6-Tetrahydropyridine-N-oxide (4): A 1M solution of 2,6-tetrahydropyridine-N-oxide7 (3.5 g, 27,5 mmol) in anhydrous THF at 0 °C were added to 27 mL of a solution of MeMgCl (6 g, 80 mmol). After 4 h at 0 °C a saturated aqueous NH4Cl solution is added, the organic phase separated and the aqueous phase extracted with Et2O. The combined organic layers were dried and concentrated to give a yellow oil (3.27 g, 83%) of N-hydroxy-2, 6, 6-hexahydropyridine sufficiently pure to be used in the next step.
Rf 0.68 (5:1 CH2Cl2-MeOH 1% NH4OH);1H-NMR (CDCl3) δ 3.62-3.78 (m, IH), 2.66-2.91 (s, br, 1H), 1.75-1.89 (m, 2H), 1.28-1.57 (in, 4H), 1.15 (s, 3H), 1.10 (d, J=6.1 Hz, 3H), 1.04 (s, 3H); 13C-NMR (CDCl3) δ 65.3 (s), 55.3 (d), 36.9 (t), 31.5 (t), 26.7 (q, 3C), 19.7 (t); IR 3650-2400, 2953, 2890, 1446, 1367cm-1; MS m/z (%): 143 (M1, 7), 128 (100), 95 (14), 70 (16), 56 (25).
To a solution of N-hydroxy-2, 6, 6-hexahydropyridine (0.148 g, 1.03 mmol) in dry CH2Cl2 (3.5 mL) at 0 °C MnO212 was added in small portions (0.179 g, 2.06 mmol). The mixture was then allowed to stir at room temperature for 6 h. The suspension was then filtered on multiple layers of silica gel, celite, and Na2SO4, and concentrated to give a light yellow oil of nitrone 4 (0.109 g, 75%) sufficiently pure for further utilization. Flash column chromatography (20:1 CH2Cl2-MeOH) on a small portion was carried out to obtain a sample for elemental analysis.
Rf 0.14 (20:1 CH2Cl2-MeOH); 1H-NMR (CDCl3) δ 2.44 (t, J = 6.2 Hz, 2H), 2.06 (s, 3H), 1.91-1.85 (m, 2H), 1.76-1.67 (m, 2H), 1.46 (s, 6H); 13C-NMR (CDCl3) δ 144.5 (s), 66.4 (s), 37.3 (t), 31.6 (t), 27.0 (q, 2C), 19.4 (q), 15.7 (t); IR 2985, 2947, 2895, 1663, 1453, 1379, 1362 cm-1; MS m/z (%): 141 (M+, 77), 126 (23), 111 (1), 96 (5), 86 (17), 84 (14), 73 (100), 69 (96), 57 (13), 55 (79). Anal. Calcd for C8H15NO: C, 68.04; H, 10.71; N, 9.92. Found: C, 67.71; H, 10.56; N, 9.97.
Typical procedure for the preparation of 5-spirocyclopropanesoxazolidines. A solution of the nitrone and methylenecyclopropane in toluene 2 mL is heated in sealed vial at 100 °C for 7 days. Concentration of the solution and purification by flash column chromatography of the residue affords the product.
3’a,7’,7’-trimethyl-hexahydrospiro[cyclopropane-1,2'-2H-isoxazolo[2,3-a]pyridine] (10): nitrone 4 (0.425 g, 3.0 mmol), methylenecyclopropane 5 (0.75 g, 13.8 mmol); pale yellow oil 164 mg, 28%; Rf 0.29 (1:7 AcOEt-petroleum ether); 1H-NMR (CDCl3) δ 2.16 (br s, 2H), 2.06-1.86 (m, 1H), 1.80-1.48 (m, 5 H), 1.36 (s, 3H), 1.18 (s, 6H), 0.98-0.80 (m, 2H), 0.68-0.42 (m, 2H); 13C-NMR (CDCl3) δ 63.1 (s), 60.9 (s), 56.9 (s), 50.2 (t), 32.9 (t), 31.8 (t), 28.3 (q), 27.9 (q), 26.8 (q), 17.2 (t), 12.8 (t), 10.2 (t); IR 2935, 2873, 1450, 1348, 1258, l134 cm-1; MS m/z (%): 195 (M+, 7), 180 (100), 152 (4), 138 (6), 124 (16), 110 (13), 97 (20), 84 (4), 82 (40), 70 (27), 55 (41). Anal. Calcd for C12H21NO: C, 73.79; H, 10.83; N, 7.17. Found: C, 73.78; H, 10.84; N, 7.17.
2,2,3’a-trimethyl-hexahydrospiro[cyclopropane-1,2'-2H-isoxazolo[2,3-a]pyridine] and 3,3,3’a- trimethyl-hexahydrospiro[cyclopropane-1,2'-2H-isoxazolo[2,3-a]pyridine] (11a,b): nitrone 6 (0.4 g, 3.5 mmol), methylenecyclopropane 79 (0.29 g, 3.5 mmol); pale yellow oil, mixture of two diastereoisomers 1.1:1 ratio, 246 mg, 36%; Rf 0.24 (1:6 AcOEt-petroleum ether); 1H-NMR (CDCl3) δ major isomer 3.15-2.95 (m, 2H), 2.25 (AB system, J = 12.1 Hz, 1H), 1.93 (AB system, J = 11.7 Hz, 1H), 1.95–1.30 (m, 6H), 1.21 (s, 3H), 1.12 (s, 3H), 1.01 (s, 3H), 0.68 (d, J = 5.5 Hz, 1H), 0.35 (d, J = 5.5, 1H); minor isomer: 3.15–2.95 (m, 1H), 2.30–2.20 (m, 1H), 1.95–1.30 (m, 8H), 1.32 (s, 3H), 1.08 (s, 3H), 0.98 (s, 3H), 0.59 (d, J = 5.8 Hz, 1H), 0.40 (d, J = 5.8 Hz 1H); 13C-NMR (CDCl3) δ major isomer 69.3 (s), 63.9 (s), 51.3 (t), 40.2 (t), 33.0 (t), 31.0 (t), 27.8 (q), 24.0 (q), 23.8 (t), 23.5 (t), 19.8 (q), 19.0 (t); minor isomer 70.0 (s), 65.2 (s), 51.5 (t), 38.8 (t), 33.7 (t), 29.7 (q), 25.0 (t), 24.6 (t), 22.1 (t), 22.6 (q), 21.0 (q), 20.5 (t); IR 2942, 2866, 1442, 1372, 1246, 1207, 1110 cm-l; MS m/z (%): 195 (M+, 20), 180 (100), 152 (14), 138 (18), 124 (30), 98 (96), 97 (70), 82 (66), 55 (94). Anal. Calcd for C12H21NO: C, 73.79; H, 10.83; N, 7.17. Found: C, 74.24; H, 11.13; N, 6.75.
General procedure for the synthesis of ketones 2 and 3 by thermal rearrangement. FVT was carried out in a standard equipment in a horizontal assembly.10 5-Spirocyclopropaneisoxazolidines were placed in a flask connected to a quartz tube, connected with a cold trap, heated by a circular oven at 450 °C. The isoxazolidine was distilled (55-70 °C) under vacuum (10-2 mmHg) through the hot tube and the rearrangement products condensed as a brown oil in the cold tube just outside the oven. The tube was washed with CH2Cl2, the solution concentrated, and the residue purified by short flash column chromatography.
6,6,9a-trimethyl-octahydro-quinolizin-2-one (2): light yellow oil 35% yield; Rf 0.23 (1:3 AcOEt-petroleum ether); 1H-NMR (CDCl3) δ 3.24 (ddd, J1 = 2.2 Hz, J2 = 7.3 Hz, J3 = 12.1 Hz, IH), 2.74 (dt, J1 = 3.2 Hz, J2 = 11.8 Hz, 1H), 2.48 (ddt, J1 = 0.7 Hz, J2 = 8 Hz, J3 = 14.3 Hz, IH), 2.36 (m, 2H), 2.05 (dd, J1 = 2.6 Hz, J2 = 13.2 Hz, IH), 1.68–1.40 (m, 6H), 1,20 (s, 3H), 1.03 (s, 3H), 1.01 (s, 3H); 13C-NMR (CDCl3) δ 210.6 (s), 59.0 (t), 56.7 (s), 53.7 (s), 42.5 (t), 41.4 (t), 41.1 (t), 40.6 (t), 32.8 (q), 20.7 (q), 17.6 (t), 17.1 (q); IR 2990, 2934, 2873, 1710, 1442, 1368, 1255 cm-1; MS m/z (%): 195 (M+, 20), 180 (100), 152 (9), 138 (15), 124 (36), 70 (15), 56 (10), 55 (22). Anal. Calcd for C12H21NO: C, 73.8, H, 10.84, N, 7.17. Found: C, 74.38, H, 10.23, N, 6.52.
4,4,9a-trimethyl-octahydro-quinolizin-2-one (3): light yellow oil, 39% yield; Rf 0.18 (1:3 AcOEt-petroleum ether); 1H-NMR (CDCl3) δ 2.88-2.82 (m, 1H), 2.62–2.28 (m, 3H), 2.18–1.92 (m, 2H), 1.78–1.30 (m, 6H), 1.21 (s, 3H), 1.04 (s, 3H), 0.95 (s, 3H). 13C-NMR (CDCl3) δ 209.9 (s), 59.4 (s), 58.5 (s), 56.4 (t), 55.8 (t), 44.3 (t), 40.3 (t), 33.7 (q), 29.6 (t), 27.2 (t), 21.1 (q), 18.0 (q); IR 2995, 2936, 2856, 1704, 1443, 1374, 1246 cm-1; MS m/z (%): 195 (M+, 11), 180 (100), 152 (6), 138 (21), 124 (20), 98 (27), 82 (58), 70 (17), 68 (14), 56 (19), 55 (89.41). Anal. Calcd for C12H21NO: C, 73.8, H, 10.84, N, 7.17. Found: C, 74.31, H, 10.99, N, 6.58.
ACKNOWLEDGEMENTS
The authors thank Ministry of University and Research – Rome (Italy) for financial support (PRIN project 2008BRXNTY).
References
1. Most recent reviews on azasteroids: (a) S. Aggarwal, S. Thareja, A. Verma, T. R. Bhardwaj, and M. Kumar, Steroids, 2010, 75, 109; CrossRef (b) M. Ibrahim-Ouali and L. Rocheblave, Steroids, 2008, 73, 375; CrossRef (c) H. Surya Prakash Rao and S. P. Senthilkumar Curr. Org. Chem., 2004, 8, 1521; CrossRef (d) J. Burbiel and F. Bracher, Steroids, 2003, 68, 587. CrossRef
2. Selected recent reviews on iminosugars bioactivity: (a) G. Horne, F. X. Wilson, J. Tinsley, D. H. Williams, and R. Storer, Drug Disc. Today, 2011, 16, 107; CrossRef (b) B. G.Winchester, Tetrahedron: Asymmetry, 2009, 20, 645; CrossRef (c) E. Borges de Melo, A. da Silveira Gomes, and I. Carvalho, Tetrahedron, 2006, 62, 10277; CrossRef (d) T. M. Wrodnigg and F. K. Sprenger, Mini-Rev. Med. Chem., 2004, 4, 437; (f) N. Asano, Curr. Top. Med. Chem., 2003, 3, 471. CrossRef
3. (a) A. Brandi, F. Cardona, S. Cicchi, F. M. Cordero, and A. Goti, Chem. Eur. J., 2009, 15, 7808; CrossRef (b) F. Cardona, S. Valenza, S. Picasso, A. Goti, and A. Brandi, J. Org. Chem., 1998, 63, 7311. CrossRef
4. (a) C. Zorn, B. Anichini, A. Goti, A. Brandi, S. I. Kozhushkov, A. de Meijere, and L. Citti, J. Org. Chem., 1999, 64, 7846; CrossRef (b) B. Anichini, A. Goti, A. Brandi, S. I. Kozhushkov, and A. de Meijere, Synlett, 1997, 25; CrossRef (c) A. Goti, B. Anichini, A. Brandi, A. de Meijere, L. Citti, and S. Nevischi, Tetrahedron Lett., 1995, 36, 5811. CrossRef
5. For selected recent references see: (a) Y. Liu and M. G. Nair, Food Chemistry, 2011, 124, 527; R. J. Peters, Nat. Prod. Rep., 2010, 27, 1521; (b) N. Girón, E. Pérez-Sacau, R. López-Fontal, J. M. Amaro-Luis, S. Hortelano, A. Estevez-Braun, and B. De Las Heras, Eur. J. Med. Chem., 2010, 45, 3155; CrossRef (c) J. Villamizar, J. P. Pittelaud, J. R. Rodrigues, N. Gamboa, N. Canudas, E. Tropper, F. Salazar, and J. Fuentes, Nat. Prod. Res., 2009, 23, 891; CrossRef (d) P. Prabhakar Reddy, A. K. Tiwari, R. Ranga Rao, K. Madhusudhana, V. Rama Subba Rao, A. Z. Ali, K. Suresh Babu, and J. Madhusudana Rao, Bioorg. Med. Chem. Lett., 2009, 19, 2562; CrossRef (e) V. Samoylenko, D. C. Dunbar, Md. A. Gafur, S. I. Khan, S. A. Ross, J. S. Mossa, F. S. El-Feraly, B. L. Tekwani, J. Bosselaers, and I. Muhammad, Phytotherapy Res., 2008, 22, 1570; CrossRef (f) A. Karioti, M. Skopeliti, O. Tsitsilonis, J. Heilmann, and H. Skaltsa, Phytochemistry, 2007, 68, 1587; CrossRef (g) K. Dimas, S. Hatziantoniou, S. Tseleni, H. Khan, A. Georgopoulos, K. Alevizopoulos, J. H. Wyche, P. Pantazis, and C. Demetzos, Apoptosis, 2007, 12, 685; CrossRef (h) J. Uddin, K. Ueda, E. R. O. Siwu, M. Kita, and D. Uemura, Bioorg. Med. Chem., 2006, 14, 6954. CrossRef
6. (a) F. M. Cordero, F. De Sarlo, and A. Brandi, Monat. Chem., 2004, 135, 649; CrossRef (b) A. Brandi, S. Cicchi, F. M. Cordero, and A. Goti, Chem. Rev., 2003, 103, 1213; CrossRef (c) A. Goti, F. M. Cordero, and A. Brandi, Top. Curr. Chem., 1996, 178, 1; (c) A. Brandi, F. M. Cordero, F. De Sarlo, A. Goti, and A. Guarna, Synlett, 1993, 1. CrossRef
7. A. Goti and L. Nannelli, Tetrahedron Lett., 1996, 37, 6025. CrossRef
8. In general six-membered ring nitrones are less stable than their 5-membered homologous and tend to form dimers. See for example: (a) J. Thesing and H. Mayer, Chem. Ber., 1956, 89, 2159; CrossRef (b) A. Brandi, S. Cicchi, D. Gomez-Pardo, and J. Cossy, Tetrahedron Lett., 2002, 43, 9357. CrossRef
9. S. Arora and P. Binger, Synthesis, 1974, 801. CrossRef
10. R. F. C. Brown, ‘Pyrolytic Methods in Organic Chemistry,’ Organic Chemistry Series Vol. 41, Chapter II, Academic Press, New York, 1980.
11. E. Ochoa, M. Mann, D. Sperling, and J. Fabian, Eur. J. Org. Chem., 2001, 4223. CrossRef
12. S. Cicchi, M. Marradi, A. Goti, and A. Brandi, Tetrahedron Lett., 2001, 42, 6503. CrossRef