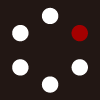
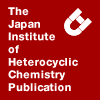
HETEROCYCLES
An International Journal for Reviews and Communications in Heterocyclic ChemistryWeb Edition ISSN: 1881-0942
Published online by The Japan Institute of Heterocyclic Chemistry
e-Journal
Full Text HTML
Received, 30th June, 2011, Accepted, 1st August, 2011, Published online, 10th August, 2011.
DOI: 10.3987/COM-11-S(P)72
■ C-[o-(p-Tolyl)sulfinyl]phenylnitrones. Synthesis and Reactivity in [3+2] Dipolar Cycloadditions
José Luis García Ruano,* Alberto Fraile,* Alberto Núñez, M. Rosario Martín, and Inés Alonso
Department of Organic Chemistry, Faculty of Sciences, Madrid Autonomous University, Madrid 28049, Spain
Abstract
The synthesis of the title compounds and the results obtained in their reactions with different dipolarophiles is presented. Alkylidene malonates afford the best conversions and stereoselectivities, providing enantiomerically pure isoxazolidines easily transformed into interesting tricyclic structures with t-BuLi.INTRODUCTION
Asymmetric [3+2] dipolar cycloadditions is one of the most powerful tools for the construction of enantiomerically pure five membered heterocycles.1 Among them, isoxazolidines are mainly prepared by inter1 or intramolecular2 reactions of nitrones. Intermolecular processes have been reported starting from chiral dipoles3 or dipolarophiles,1c,1d,3,4 or using asymmetric catalysis.5,6 The sulfinyl group has been used for controlling the stereochemistry of many reactions involving nitrones, but in most of cases it is located at the dipolarophile,7 with only three precedents when it is located at the nitrone.8 In the course of our research program devoted to asymmetric cycloadditions mediated on sulfoxides,9 we were interested in the preparation of C-arylnitrones bearing a p-tolylsulfinyl group at o-position, and the study of their cycloadition reactions, because 2-(p-tolylsulfinyl)benzyl fragment had provided excellent results in many other asymmetric processes previously studied.10,11 Results obtained in this study are here reported.
RESULTS AND DISCUSSION
Enantiomerically pure starting aldehyde 1, used for the synthesis of nitrones 2-5, was prepared following the procedure reported,12 as indicated in Scheme 1.
Condensation of 1 with N-alkylhydroxylamine hydrochloride or N-phenylhydroxylamine in the presence of Na2CO3 as base and molecular sieve or MgSO4, yielded the nitrones 2-5 (Scheme 2). Compounds 2 and 3 are obtained at room temperature with very high or quantitative yields. Reaction with N-phenylhydroxylamine is slower at room temperature, being so it was needed to heat at 50 ºC to obtain better results of 4 (65% yield in 1 day). N-t-butylhydroxylamine is even less reactive and the yield of 5 is only 10% after 3 days. These results indicate that condensation is sensitive to the steric effects.
The enantiomeric excess of 2, determined by chiral HPLC, resulted to be identical to that of the starting aldehyde, which indicates that reaction conditions used for preparing nitrones do not affect the optical purity of the sulfinyl centre. The structure of 2, determined by X-ray diffraction studies (Figure 1),13 shows the Re-face of the nitrone carbon hindered by the tolyl ring joined to the sulfinyl group, thus predicting a high diastereofacial selectivity in the approaches of the different reagents.
Reactions with olefins bearing alkyl or alkoxy groups (electron-rich double bonds) were unsuccessful. Cyclohexene and 1-hexene do not react with 2 after 6 days in refluxing toluene. The formation of isoxazolidines could be detected by NMR of the reaction mixtures of 2 with styrene, but they could not be isolated as pure compounds.
The reaction of 2 with ethyl vinyl ether affords, after 6 days at 110 ºC, a 3:1 mixture of isoxazolidines. Under these conditions conversion was not complete (see Scheme 3) and the aldehyde 1, resulting in the hydrolysis of 2, was also detected. Separation of adducts was not possible in our hands. We repeated the reaction at 50 ºC looking for a higher selectivity that made possible the isolation and characterization of the major isoxazolidine. However, the conversion was very low after 6 days and only traces of the cycloadducts were detected.
Electron poor olefins bearing only one electron-withdrawing group (EWG) provided poor results of stereoselectivity. Reaction of 2 with methyl vinyl ketone (excess) at 50 ºC for 3 days afforded complex crude mixture of products containing polymerization products and a 1:1 mixture of isoxazolidines whose separation was not possible. Under these conditions reaction of 2 with pent-3-en-2-one (excess), with lower tendency to polymerization, afforded a mixture in which the major components were 6a and 6b (1:1), that were isolated in 80% combined yield (Scheme 4). After separation by column chromatography, the relative stereochemistry of 6a and 6b was determined as (r-4,c-3,t-5) by NOESY experiments. The absolute configuration of these compounds could not be assigned.
A similar result was obtained from (E)-2-nitrostyrene (Scheme 4) which afforded a mixture of isoxazolidines 7a and 7b in 85% combined yield. Despite their separation was not possible, their structure and regiochemistry could be established from the NMR spectra of the mixture. Other nitroalkenes and vinyl sulfones were studied but the results were always unsuccessful.
The best results were obtained in reactions of nitrones with olefins gem-disubstituted by electron-withdrawing groups, and of special interest are the reactions with diethyl ethylidenemalonate because there are not many results in the literature concerning their reactions with nitrones.14 Reaction of nitrone 2 with diethyl ethylidenemalonate for 7 days in toluene at 50 ºC afforded a 83:17 mixture of isoxazolidines 8a and 8b (Scheme 5), which were separated by column chromatography and isolated with 85% of combined yield.
The use of higher temperatures reduces the reaction time but also the stereoselectivity, whereas the change of the solvent (CH2Cl2 instead of toluene) has not significant consequences in reactivity nor selectivity. Under the same conditions diethyl benzylidene malonates did not react. Despite the low reactivity of this dipolarophile with N-methyl α-phenylnitrone had been reported,4c we cannot discard that the different behavior of benzylidene and ethylidene malonates could be due to the easier cycloreversion of the first one due to the stabilization of the double bond exerted by the phenyl group.
The absolute configuration of the major isoxazolidine 8a has been unequivocally established by X-ray diffraction studies.13 NOESY experiments allowed establishing the trans relationship of the aryl (at C-3) and methyl (at C-5) in both diastereoisomers. As we know the absolute configuration of 8a (Figure 2), that of 8b should be that shown in Scheme 5.
We then studied the reactions of diethyl ethylidene malonate with nitrones 3-5 to check the influence of the substituent at nitrogen on the course of these cycloadditions. Compound 5 remained unaltered after 3 days at 50 ºC in toluene, indicating that the steric hindrance exerted by the t-butyl group preclude the approach of the dipolarophile. The easily hydrolysable N-benzylnitrone 3 afforded a low amount of the possible adducts, which could not be isolated after 3 days in similar conditions (Scheme 6), whereas N-phenylnitrone 4 provided the best result. After 3 days at 50 ºC, starting nitrone had been consumed and isoxazolidine 9a was obtained with 90% de, and pure diasteromer was isolated in 73% yield (Scheme 6).
The last dipolarophile we have studied was the 1,1-bis-phenylsulfonylethylene. After 5 days in toluene at 50 ºC, 2 had disappeared (TLC) and a 60:40 mixture of adducts 10a and 10b was isolated in almost quantitative combined yield (Scheme 7). Although the separation of both compounds was not possible in our hands, we could establish by 1H NMR of the mixture, they have the opposite regiochemistry to that observed from diethyl ethylidene malonate. The large size of the two sulfonyl groups must be responsible that the preferred attack of the dipole takes place with the oxygen approaching to the most hindered carbon at dipolarophile, thus minimizing the steric interactions.
We have also studied the reactions of 2 with many other gem-disubstituted cyclic and acyclic dipolarophiles, but the results were unsuccessful.
Concerning the course of these reactions, two main questions must be explained. The first one is the reactivity of these nitrones toward dipolarophiles mono or gem-diactivated and the second one the stereoselectivity observed in these processes. We have performed theoretical calculations at the DFT (mPW1PW91)15 level, with the CPCM model,16 by using the Gaussian09 program17 of the relative stability of the different conformations of nitrone 2 at their two possible configurations, Z and E, around the C=N bond. Z-diastereoisomers resulted to be at least 6 kcal/mol more stable than the corresponding E-isomers. The two most stable rotamers found in solvent-phase (toluene) for Z-2, C1 and C2, are indicated in Scheme 8. C1 is mainly stabilized by the hydrogen bond between the sulfinyl oxygen and the hydrogen at C-1 of the nitrone. C2 is less stable than C1 by only 0.6 kcal/mol and arrange the sulfinyl oxygen far from the H at C-1. The orientation of the tolyl group is opposite in both conformations and precludes the approach of the dipolarophile to a different face. It would explain that resulting isoxazolidines exhibit the opposite configuration at the carbon.18 A similar behavior was observed for N-phenylnitrone 4. Other interesting point is that the most stable conformations predicted by calculations are clearly different to that observed in solid state, because C1 and C2 have the aromatic ring conjugated with C=N (coplanar). Finally, we have performed the NBO analyses18 of the calculated structures that provides information about the charge density localized at the different atoms of the nitrone. From these calculations a surprising low negative natural charge was assigned to the carbon atom taking part in the double bond at the nitrone (Scheme 8), which could be the related to the reactivity of these dipoles.20 However, we must also consider that the easy cycloreversion of many of these reactions with strongly stabilized dipolarophiles could be the reason of the apparent low reactivity of these nitrones.
In order to explain the stereochemical course of these reactions, we assume that the approach of the diethyl ethylidenemalonate can only take place to the less hindered face of each of the two most stable conformations of the nitrone (C1 and C2). The approach of the dipole to the Si face of dipolarophile is favored in order to avoid the interaction of the methyl group at the alkene with the aromatic ring of the nitrone. According to these rules, the two possible TSs will be I from C1 and II from C2 (Scheme 8). The formation of 8a as the major product suggests the lower stability of the TS I which could be explained by the electronic repulsion of the sulfinyl and carbonyl oxygens indicated in Scheme 8. This repulsion would be lower for TS II due to the longer distance between both oxygen atoms.
One interesting transformation we performed on the adducts 8a and 9a, resulting in reaction of nitrones with diethyl ethylidene malonate, was observed in the reaction with t-BuLi. It initially affords t-butyl p-tolyl sulfoxide and the corresponding aromatic carbanion, which evolved attacking to the ester group (instead of suffering protonation20) and affording the 3,3a,4,8b-tetrahydro-1H-indeno[1,2-c]isoxazoles 11 and 12 with isolated yields of 58% and 52% respectively (Scheme 9). The transformation involves desimetrization of C-4 of isoxazolidine with generation of a quaternary carbon in a completely stereocontrolled manner. The stereochemistry of the obtained compounds is that resulting in the attack to the ester group in cis arrangement with respect to the phenyl ring where the carbanion is generated (Scheme 9).
In conclusion we described the preparation of enantiomerically pure α-2-(p-tolylsulfinyl)phenylnitrones and their use as dipoles in asymmetric synthesis. The reactivity of these compounds is dependent on the dipolarophile, the diethyl ethylidenemalonate is reactive and give the best diastereofacial selectivity of 95:5. The major diastereomeric cycloadducts can be transformed into enantiopure tetrahydroindeno[1,2-c]isoxazole derivatives on treatment with t-butyllithium. Theoretical calculation at DTF level revealed the higher stability of the Z configuration of the nitrones and the existence of two conformations of similar stability the evolution of which allows explaining the observed stereoselectivity.
EXPERIMENTAL
All melting points were determined in open capillary tubes and are uncorrected. IR spectra were determined as it is indicated in each compound with a Bruker FTIR-Vector 22 spectrophotometer. The NMR spectra were determined using TMS as an internal reference on a Bruker AC-300 FT NMR spectrometer operating at 300 and 75 MHz for 1H and 13C NMR, respectively. High resolution mass spectrometry (HRMS), FAB: Waters, VG AutoSpec.The optical rotations were measured at room temperature in the solvent and concentration (gr/100 mL) indicated in each case. Silica gel 60 (230-400 mesh ASTM) and DC-Alufolien 60 F254 were used for flash column chromatography and analytical TLC, respectively. Acetonitrile was dried over activated molecular sieves 4 Å. Microanalyses were carried out on a LECO CHNS-932 in Laboratory of elemental analyses of SIDI of Universidad Autónoma de Madrid, and were in good agreement with the calculated values.
Starting Materials. (S)-1-(Diethoxymethyl)-2-(p-tolylsulfinyl)benzene12 was prepared by previously reported procedure. All other chemicals used in this study were commercially available. Geometries were fully optimized in the solvent-phase (toluene with CPCM model) at the DFT (mPW1PW91) level using the standard 6-31G(d) basis set for all the atoms. Frequencies and zero-point energy (ZPE) were also computed at the same level of theory. Final energies and NBO analysis were obtained using the more extended 6-311G(d,p) basis set for all atoms. Relative free energies (in kcal/mol) were evaluated at the mPW1PW91/6-311G(d,p) level with ZPE and entropy corrections evaluated at 298 K using the frequencies previously calculated at mPW1PW91/6-31G(d) level.
Synthesis of α-(p-tolylsulfinyl)phenylnitrones. (S)-2-(p-Tolylsulfinyl)benzaldehyde (1). To a solution of (S)-1-(diethoxymethyl)-2-(p-tolylsulfinyl)benzene (1.27 g, 4.0 mmol) in a mixture of acetone (100 mL) and water (7 mL) was added 0.70 g (2.78 mmol) of pyridinium p-toluenesulfonate (PPTS). Then, the mixture was stirred at 50 ⁰C for 3 h and the solvent was removed under reduced pressure. The residue was dissolved in a mixture of CH2Cl2 (100 mL) and water (25 mL). The organic layer was separated and the aqueous was extracted with CH2Cl2 (2 x 25 mL). The combined of organic layer was dried (Na2SO4) and the solvent removed, the compound 1 was obtained in 98% and used in the next step without any purification; a yellow solid; mp 106-108 ºC; [α]20D -238.8 (c 1.0, CHCl3); IR (KBr) 1678, 1210, 1022 cm-1; 1H NMR (300 MHz, CDCl3) δ 2.32 (s, 3H), 7.57 and 7.16 (AA’BB’ system, 4H), 7.69 (dt, J =1.1, 7.4 Hz, 1H), 7.96-7.83 (m, 2H), 8.51 (d, J =7.2 Hz, 1H), 9.97 (s, 1H); 13C NMR (CDCl3) δ 21.3, 124.6, 126.6 (2C), 129.7 (2C), 130.6, 132.7, 134.2, 134.9, 141.6, 142.6, 148.1, 190.6; HRMS-(FAB +) m/z [M+H+] calcd for C14H13O2S: 245.0636, found: 245.0629.
(S,Z)-N-Methyl-α-[2-(p-tolylsulfinyl)phenyl]nitrone (2). A mixture of aldehyde 1 (2.0 g, 8.2 mmol), N-methylhydroxylamine hydrochloride (0.68 g, 8.2 mmol), NaHCO3 (1.4 g, 16.4 mmol), and molecular sieves 4 Å (8.0 g) in 80 mL of absolute EtOH was stirred for 4 days at rt. Then, the reaction mixture was filtered through Celite and washed with EtOH. The solvent was removed under reduced pressure and the residue was purified by column chromatography on silica gel (95:5 AcOEt-MeOH) to give 2 (86%); a white solid; mp 115-117 ºC; [α]20D -210.9 (c 1.0, CHCl3); IR (KBr) 1585, 1436, 1411, 1174, 762, 623 cm-1; 1H NMR (300 MHz, CDCl3) δ 2.36 (s, 3H), 3.82 (s, 3H), 7.24 and 7.39 (AA’BB’ system, 4H), 7.55 (m, 2H), 7.90 (m, 1H), 8.11 (s, 1H), 9.16 (m, 1H); 13C NMR (CDCl3) δ 21.3, 55.1, 124.9 (2C), 126.5, 128.9, 129.1, 130.1 (2C), 130.5, 131.7, 141.2, 141.6, 141.9; HRMS-ESI m/z [M+H+] calcd for C15H16NO2S: 274.0902, found: 274.0903. HPLC Daicel Chiralpack AD (75:25 hexane-i-PrOH, 1mL/min), 96% ee, tR = 16.8 min. (±)-2 tR = 16.4 min and 18.7 min.
(±)-(Z)-N-Benzyl-α-[2-(p-tolylsulfinyl)phenyl]nitrone (3). A mixture of racemic aldehyde 1 (244 mg, 1.0 mmol), N-benzylhydroxylamine hydrochloride (160 mg, 1.0 mmol), NaHCO3 (168 mg, 2.0 mmol), MgSO4 (481 mg) and of absolute EtOH (10 mL) was stirred for 24 h at rt. Then, the reaction mixture was filtered through Celite and washed with CH2Cl2. The filtrate was concentrated under reduced to afford a white solid in 95% yield. 1H NMR (300 MHz, CDCl3) δ 2.35 (s, 3H), 5.00 (s, 2H), 7.17 (AA’BB’ system, 4H), 7.43-7.34 (m, 5H), 7.54 (m, 2H), 7.94 (m, 1H), 8.07 (s, 1H), 9.23 (m, 1H).
(S,Z)-N-Phenyl-α-[2-(p-tolylsulfinyl)phenyl]nitrone (4). A mixture of 2-(p-tolylsulfinyl)benzaldehyde (1) (500 mg, 2.05 mmol), N-phenylhydroxylamine (374 mg, 3.4 mmol), NaHCO3 (571 mg, 6.8 mmol), 4 g molecular sieves 4 Å and dry CH2Cl2 (20 mL) was heated at 50 ºC with vigorous stirring under argon atmosphere for 24 h. After cooling to rt, the reaction mixture was filtered through Celite and the solid was washed with abundant CH2Cl2 and the solvent of filtrate was removed under reduced pressure. The residue was purified by column chromatography on silica gel (3:1 hexane-AcOEt) to give sulfinylnitrone 4 (65%); a yellow oil; [α]20D -133.7 (c 1.0, CHCl3); 1H NMR (300 MHz, CDCl3) δ 2.34 (s, 3H), 7.21 and 7.38 (AA’BB’ system, 4H), 7.47 (m, 3H), 7.57-7.66 (m, 4H), 7.98 (m, 1H), 8.68 (s, 1H), 9.43 (m, 1H); 13C NMR (CDCl3) δ 21.3, 121.6, 125.1, 126.9, 128.4, 129.1, 129.2 (2C), 130.1, 130.3, 130.7, 131.8, 141.4, 141.6, 142.5, 149.1; HRMS-(FAB +) m/z [M+H+] C20H18NO2S: 336.1058, found: 336.1058.
(±)-(Z)-α-[2-(p-Tolylsulfinyl)phenyl]-N-tert-butylnitrone (5). A mixture of racemic 2-(p-tolylsulfinyl)benzaldehyde (1) (100 mg, 0.41 mmol), N-tert-butylhydroxylamine (88 mg, 0.70 mmol), NaHCO3 (117 mg, 1.4 mmol), 500 mg of molecular sieves 4Å and 5 mL of dry CH2Cl2 was heated at 50 ºC with vigorous stirring under argon atmosphere for 3 days. After cooling to rt, the reaction mixture was filtered through Celite and the solid was washed with abundant CH2Cl2 and the solvent of filtrate was removed under reduced pressure. The residue was purified by column chromatography on silica gel (1:1:1 hexane- CH2Cl2-Et2O) to give sulfinylnitrone 5 (10%); and 50 mg of starting aldehyde 1.1H NMR (300 MHz, CDCl3) δ 1.49 (s, 9H), 2.34 (s, 3H), 7.22 and 7.37 (AA’BB’ system, 4H), 7.54 (m, 2H), 7.93 (m, 1H), 8.26 (s, 1H), 9.26 (m, 1H); 13C NMR (CDCl3) δ 21.3, 28.1, 72.0, 124.1, 125.2, 126.8, 129.1, 129.7, 129.88, 129.95, 131.7, 141.5, 141.6, 141.9.
General procedure for cycloadition reactions. To a stirred mixture of nitrone 2, 3, 4 or 5 (0.17 mmol) and dipolarophile (excess), and in any case 0.5 mL of dry toluene, was heated to 50 ºC. The reaction was monitored by TLC; after the time indicated in each case, the solvent and/or excess of dipolarophile was removed under reduced pressure and the residue analyzed by 1H NMR and purified by column chromatography on silica gel.
4-Acetyl-2,5-dimethyl-3-[2-(p-tolylsulfinyl)phenyl]isoxazolidines (6a,b): They were obtained as mixture of stereoisomers (a/b= ca. 1:1) by reaction of nitrone 2 (50 mg, 0.18 mmol) and but-3-en-2-one (0.25 mL), at 50 ºC for 4 d without solvent. They were isolated diastereoisomerically pure by column chromatography on silica gel (3:2 hexane-AcOEt).
6a (or b) Rf = 0.33 3:2 (hexane-AcOEt ), in 41% yield; colorless oil; [α]20D + 83.5 (c 1.0, CHCl3); 1H NMR (300 MHz, CDCl3) δ 1.26 (s, 3H), 1.27 (d, J =6.4 Hz, 3H), 2.10 (s, 3H), 2.38 (s, 3H), 3.21 (dd, J =10.0, 7.3 Hz, 1H), 4.34 (d, J =10.0 Hz, 1H), 4.68 (m, 1H), 7.29 (d, J =8.1 Hz, 2H), 7.54-7.44 (m, 5H), 7.97 (m, 1H); 13C NMR (CDCl3) δ 18.6, 21.3, 30.9, 42.0,. 66.9, 69.6, 75.1, 125.7, 126.9, 128.8, 130.0, 130.8, 131.9, 135.5, 141.5, 141.7, 143.3, 206.4; HRMS-(ESI +) m/z [M+H+] calcd for C20H24NO3S: 358.1477, found: 358.1479.
6b (or a) Rf = 0.17 (3:2 hexane-AcOEt); 39% yield; colorless oil; [α]20D -230.7 (c 1.5, CHCl3); 1H NMR (300 MHz, CDCl3) δ 1.31 (d, J 6.1, 3H), 1.58 (s, 3H), 2.21 (s, 3H), 2.39 (s, 3H), 3.45 (dd, J =10.0, 7.3 Hz, 1H), 4.34 (d, J =10.0 Hz, 1H), 4.71 (m, J =6.4 Hz, 1H), 7.29 (d, J =8.1 Hz, 2H), 7. 45-7.54 (m, 5H), 7.87 (m, 1H); 13C NMR (CDCl3) δ 18.7, 21.4, 31.4, 42.3, 67.0, 69.9, 75.1, 125.3, 126.5, 129.3, 129.5, 130.2, 131.8, 134.5, 140.8, 142.6, 143.3, 205.8; HRMS-(ESI +) m/z [M+H+] calcd for C20H24NO3S: 358.1477, found: 358.1473.
2-Methyl-4-nitro-5-phenyl-3-[2-(p-tolylsulfinyl)phenyl]isoxazolidines (7). They were obtained as mixture of stereoisomers (a/b= ca. 1:1) by reaction of nitrone 2 (50 mg, 0.18 mmol) and β-nitrostirene (0.73 mmol) in toluene (0.5 mL) at 50 ºC for 4 d. They were isolated and purified by column chromatography on silica gel (2:1 hexane-AcOEt) in 85% combined yield, but their separation was not possible in our hands; 1H NMR (300 MHz, CDCl3) δ 2.27 (s, 3H), 2.33 (s, 3H), 2.42 (s, 3H), 2.55 (s, 3H), 4.40 (dd, J =7.7, 4.8 Hz, 1H), 4.61 (d, J =7.7 Hz, 1H), 4.77 (d, J =7.6 Hz, 1H), 5.05 (dd, J =7.7, 5.1 Hz, 1H), 5.77 (d, J =4.8 Hz, 1H), 5.80 (d, J =5.1 Hz, 1H), 7.12 (d, J =8.0 Hz, 2H), 7.21 (d, J =8.0 Hz, 2H), 7.29-7.63 (m, 20H), 7.83 (m, 1H), 7.88 (m, 1H); 13C NMR (CDCl3) δ 21.2, 21.3, 42.5, 42.6, 70.0, 71.5, 81.8, 82.2, 97.5, 98.1, 125.6, 125.9, 126.4, 126.7, 127.5, 128.1, 128.87, 128.93, 129.07, 129.09, 129.2, 129.7, 129.9, 130.06, 130.12, 131.57, 131.62, 132.2, 132.3, 136.0, 136.3, 140.4, 140.9, 141.7, 141.9, 143.1, 143.5; HRMS-ESI m/z [M+H+] calcd for C23H23N2O4S: 423.1379, found: 423.1375.
Diethyl 2,5-dimethyl-3-[2-((S)-p-tolylsulfinyl)phenyl]isoxazolidine-4,4-dicarboxylates (8): a mixture of stereoisomers (a/b= 83:17) were obtained from nitrone 2 and 2.5 the equimolecular amount of diethyl ethylidenemalonate after 7 days of reaction at 50 ºC. They were separated by column (3:2 hexane, AcOEt).
(SS,3S,5R)-8a: 75% yield of a white solid, mp 148-150 ºC; [α]20D -148.0 (c 1.0, CHCl3); 1H NMR (300 MHz, CDCl3) δ 0.80 (t, J =7.2 Hz, 3H), 1.27 (d, J =6.5 Hz, 3H), 1.32 (t, J =7.2 Hz, 3H), 1.97(s, 3H), 2.35 (s, 3H), 3.29 (dq, J =10.7, 7.2 Hz, 1H), 3.76 (dq, J =10.7, 7.2 Hz, 1H), 4.27 (m, 1H), 4.40 (m, 1H), 4.80 (s, 1H), 4.88 (q, J =6.5 Hz, 1H), 7.43 (td, J =7.8, 1.3 Hz, 1H), 7.56 (td, J =7.8, 1.3 Hz, 1H), 7.61 (dd, J =7.8, 1.3 Hz, 1H), 7.24 and 7.68 (AA’BB’ system, 4H), 8.22 (dd, J =7.8, 1.3 Hz, 1H); 13C NMR (CDCl3) δ 13.3, 14.1, 14.2, 21.4, 41.9, 61.3, 62.1, 71.9, 72.2, 78.4, 123.3,. 127.7, 128.6, 129.1, 130.0, 130.7, 134.4, 141.8, 142.6, 145.0, 167.3, 168.5. Anal. Calcd for C24H29NO6S; C, 62.73; H, 6.36; N, 3.05; S, 6.98. Found: C, 62.87; H, 6.31; N, 3.13; S, 6.89. HPLC Daicel Chiralpack AD (95: 5 hexane-i-PrOH, 1mL/min), 96% ee, tR = 36.4 min, (±)-8 tR = 33.0 min and 36.7 min.
(SS,3R,5S)-8b colorless oil, 10% yield; 1H NMR (300 MHz, CDCl3) δ 0.40 (t, J =6.9 Hz, 3H), 1.21 (t, J= 7.1 Hz, 3H), 1.28 (d, J =6.1 Hz, 3H), 2.32 (s, 3H), 2.67 (s, 3H), 3.23 (m, 1H), 3.54 (m, 1H), 4.17 (m, 2H), 4.98 (q, J =6.1 Hz, 1H), 5.34 (br.s, 1H), 7.18 (m, 2H), 7.33-7. 57 (m, 5H), 7.85 (m, 1H);13C NMR (CDCl3) δ 13.1, 14.1, 14.5, 21.3, 42.8, 60.9, 61.9, 72.3, 72.5, 78.9, 125.6, 125.9, 128.9, 129.5, 129.7, 131.1, 136.5, 141.2, 141.7, 146.0, 167.5, 168.3; HRMS-(ESI +) m/z [M+H+] calcd for C24H30NO6S: 460.1794, found: 460.1786.
(SS,3S,5R) Diethyl 5-methyl-2-pheny-3-[2-p-tolylsulfinyl)phenyl]isoxazolidine-4,4-dicarboxylate (9a): it was obtained from nitrone 4 and 2.5 equimolecular amount of diethyl ethilydenemalonate after 24 h, as a colorless oil, in 73% yield after column chromatography on silica gel (2:1 hexane-AcOEt); [α]20D +5.2 (c 0.5, CHCl3); 1H NMR (300 MHz, CDCl3) δ 0.95 (t, J =7.1 Hz, 3H), 1.34 (t, J =7.2 Hz, 3H), 1.41 (d, J =6.4 Hz, 3H), 2.32 (s, 3H), 3.37 (dq, J =10.7, 7.1 Hz, 1H), 3.84 (dq, J =10.7, 7.2 Hz, 1H), 4.25-4.48 (m, 2H), 5.09 (q, J =6.4 Hz, 1H), 5.58 (s, 1H), 6.41 (m, 2H), 6.76-6.83 (m, 3H), 7.05 (d, J =8.0 Hz, 2H), 7.41-7.48 (m, 3H), 7.56 (m, 1H), 7.85 (d, J =7.2 Hz, 1H), 8.20 (dd, J =7.8, 1.0 Hz, 1H); 13C NMR (CDCl3) δ 13.4, 14.2 (2C), 21.5, 61.8, 62.3, 70.2, 72.2, 78.1, 117.8, 123.1, 123.2, 127.0, 128.3, 128.8, 129.5, 130.9, 134.9, 140.5, 142.2, 145.1, 148.1, 166.9, 168.2; HRMS-(ESI +) m/z [M+H+] calcd for C29H32NO6S: 522.1950, found: 522.1941.
5,5-Bis(phenylsulfonyl)-2-methyl-3-[2-(p-tolylsulfinyl)phenyl]isoxazolidines (10). A mixture of stereoisomers (a/b= 1.5:1) was obtained in 95% yield, after purification by column chromatography on silica gel (1:1 hexane, AcOEt), from nitrone 2 and 1.5 the equimolecular amount of diethyl ethylidenemalonate after 5 days at 50 ºC. 1H NMR (300 MHz, CDCl3) δ: 2.21 (s, 3H, a isomer), 2.39 (s, 3H, a isomer), 2.40 (s, 3H, b isomer), 2.57 (s, 3H, b isomer), 3.04-2.70 (m, 2H, a isomer + 1H, b isomer), 3.40 (dd, J =14.8, 6.5 Hz, 1H, b isomer), 4.39 (2 dd, 2H, a and b isomers), 6.84 (d, J =7.6 Hz, 1H, b isomer), 7.01 (d, J =7.1 Hz, 1H, a isomer).
Tándem desulfinylation-cyclization reaction. To a stirred 0.03 M solution of cycloaducts 8a or 9a in dry THF, at -78 ⁰C and under argon atmosphere, was added 1.7 M t-BuLi in pentane (two equimolecular amount). After stirring for 15 min at -78 ºC, the reaction mixture was quenched with saturated aqueous NH4Cl and extracted with AcOEt. The organic layers were dried over MgSO4, and concentrated under reduced pressure. The residue was purified by column chromatography on silica gel (3:1 hexane-AcOEt).
(3R,3aR,8bR) Ethyl 1,3-dimethyl-4-oxo-3,3a,4,8b-tetrahydro-1H-indene[1,2-c]isoxazolo-3a-carboxylate [(+)-11]; was obtained from cycloadduct 8a as colorless oil in 58% yield; [α]20D +44.5 (c 0.4, CHCl3); 1H NMR (500 MHz 50 ºC, CDCl3) δ 1.30 (t, J =7.2 Hz, 3H), 1.47 (d, J =6.5 Hz, 3H), 2.90 (s, 3H), 4.24 (m, 2H), 4.39 (q, J =6.5 Hz, 1H), 4.88 (brs, 1H), 7.48-7.52 (m, 2H), 7.66 (td, J =7.5, 1.1 Hz, 1H), 7.78 (dd, J =7.7, 0.6 Hz, 1H); 13C NMR (500 MHz, 50 ºC, CDCl3) δ 14.1, 62.1, 78.3, 125.1, 129.9, 135.6, 166.9, 198.8; HRMS-(ESI +) m/z [M+H+] calcd for C15H18NO4 (M+H), 276.1230, found: 276.1229 (100%).
(3R,3aR,8bR) Ethyl 3-methyl-4-oxo-1-phenyl-3,3a,4,8b-tetrahydro-1H-indene[1,2-c]isoxazolo-3a-carboxylate [(-)-12]; was obtained from cycloadduct 9a as colorless oil in 52% yield; [α]20D -296.4 (c 0.7, CHCl3); 1H NMR (300 MHz, CDCl3) δ 1.28 (t, J =7.1 Hz, 3H), 1.44 (d, J =6.6 Hz, 3H), 4.24 (m, 2H), 4.78 (q, J =6.6 Hz, 1H), 5.92 (s, 1H), 7.03 (m, 1H), 7.19 (m, 2H), 7.32 (m, 2H), 7.39 (d, J =7.7 Hz, 1H), 7.48 (m, 1H), 7.59 (td, J =7.7, 1.2 Hz, 1H), 7.79 (d, J =7.7 Hz, 1H); 13C NMR (CDCl3) δ 14.1, 17.4, 62.3, 70.4, 75.7, 81.0, 116.2, 122.8, 124.4, 127.0, 128.9, 129.7, 135.2, 135.9, 149.4, 150.2, 165.7, 197.7; HRMS-(ESI +) m/z [M+H+] calcd for C20H20NO4 (M+H) 338.1392, found: 338.1395 (54%); calcd for C20H19NO4Na (M+Na) 360.1212, found: 360.1209 (100%).
ACKNOWLEDGEMENTS
We thank DGICYT (Grant CTQ2009-12168/BQU) and Comunidad Autónoma de Madrid (AVANCAT S2009/PPQ-1634) for financial support. Computational time provided by the Centro de Computación Científica at the UAM (CCC-UAM) is gratefully acknowledged.
References
1. (a) J. J. Tufariello, 'Dipolar Cycloaddition Chemistry, ' Vol 2, ed. by A. Padwa, John Wiley and Sons, New York: 1984; chapter 9, p. 83; (b) P. N. Confalone and E. M. Huie, 'Organic Reactions', Vol. 36, ed. by A. S. Kende, John Wiley and Sons, 1988, charter 1, p. 1; (c) M. Harwood and R. J. Vickers, 'Synthetic Applications of 1,3-Dipolar Cycloaddition Chemistry Toward Heterocycles and Natural Products,' ed. by A. Padwa and W. H. Pearson, John Wiley-Interscience, 2003; (d) H. Pellissier, Tetrahedron, 2007, 63, 3235; CrossRef (e) K. V. Gothelf and K. A. Jørgensen, Chem. Rev., 1998, 98, 863; CrossRef (f) M. Frederickson, Tetrahedron, 1997, 53, 403. CrossRef
2. For some recent examples: (a) V. Nair and T. D. Suja, Tetrahedron, 2007, 63, 12247; CrossRef (b) A. J. M. Burrell and I. Coldham, Current Organic Synthesis, 2010, 7, 312; CrossRef (c) F. A. Davis, N. Theddu, and R. Edupuganti, Org. Lett., 2010, 12, 4118. CrossRef
3. (a) G. Romeo, D. Iannazzoa, A. Pipernoa, R. Romeoa, A. Corsarob, A. Rescifinab, and U. Chiacchio, Mini-Reviews in Organic Chemistry, 2005, 2, 59; CrossRef (b) J. Revuelta, S. Cicchi, A. Goti, and A. Brandi, Synthesis, 2007, 485; CrossRef (c) H. M. I. Osborn, N. Gemmell, and L. M. Harwood, J. Chem. Soc., Perkin Trans 1, 2002, 2419. CrossRef
4. (a) M. Kissane and A. R. Maguire, Chem. Soc. Rev., 2010, 39, 845; CrossRef (b) H. Tsuge, T. Okano, S. Eguchi, and H. Kimoto, J. Chem. Soc., Perkin Trans 1, 1997, 1581; CrossRef (c) N. Katagiri, N. Watanabe, J. Sakaki, T. Kawai, and C. Kanebo, Tetrahedron Lett., 1990, 31, 4633. CrossRef
5. L. M. Stanley and M. P. Sibi, Chem. Rev., 2008, 108, 2887. CrossRef
6. (a) Z.-Z. Huang, Y.-B. Kang, J. Zhou, M.-C. Ye, and Y. Tang, Org. Lett., 2004, 6, 1677; CrossRef (b) D. Chen, Z. Wang, J. Li, Z. Yang, L. Lin, X. Liu, and X. Feng, Chem. Eur. J., 2011, 17, 5226; CrossRef (c) S. Barroso, G. Blay, M. C. Muñoz, and J. R. Pedro, Org. Lett., 2011, 13, 402; CrossRef (d) T. Hashimoto, M. Omote, T. Kano, and K. Maruoka, Org. Lett., 2007, 9, 4805; CrossRef (e) L. Weseliński, E. Słyk, and J. Jurczak, Tetrahedron Lett., 2011, 52, 381. CrossRef
7. J. L. Garcia Ruano, J. Alemán, M. B. Cid, M. A. Fernández-Ibañez, M. C. Maestro, M. R. Martín, and A. M. Martín Castro, in Organosulfur Chemistry in Asymmetric Synthesis; ed. by T. Toru and C. Bolm, Wiley-VCH, Weinhein, 2008, p. 111.
8. (a) S. Murahashi, J. Sun, H. Kurosawa, and Y. Imada, Heterocycles, 2000, 52, 557; CrossRef (b) V. K. Aggarwal, S. J. Roseblade, J. K. Barrell, and R. Alexander, Org. Lett., 2002, 4, 1227; CrossRef (c) V. K. Aggarwal, S. Roseblade, and R. Alexander, Org. Biomol. Chem., 2003, 1, 684. CrossRef
9. (a) A. Nuñez Jr., M. R. Martín, A. Fraile, and J. L. García Ruano, Chem. Eur. J., 2010, 16, 5443; CrossRef (b) J. L. García Ruano, A. Fraile, M. R. Martin, G. Gonzalez, C. Fajardo, and A. Martin-Castro, J. Org. Chem., 2011, 76, 3296 and references cited therein. CrossRef
10. Ref. 7, p. 123.
11. Recent references: (a) J. L. García Ruano, E. Torrente, and A. M. Martin-Castro, J. Org. Chem., 2011, 76, 3597; CrossRef (b) J. L. García Ruano, A. Parra, I. Alonso, S. Fustero, C. del Pozo, Y. Arroyo, and A. Sanz-Tejedor, Chem. Eur. J., 2011, 17, 6142 and references cited therein. CrossRef
12. C.-Y. Lai, W.-L. Mak, E. Y. Y. Chan, Y.-K. Sau, Q.-F. Zhang, S. M. F. Lo, I. D. Williams, and W.-H. Leung, Inor. Chem., 2003, 42, 5863. CrossRef
13. The authors have deposited atomic coordinates for nitrone 2 and isoxazolidine 8a with the Cambridge Crystallographic Data Centre (Deposit Number 831759, and 831760, respectively). Copies of the data can be obtained, free of charge, on application to CCDC, 12 Union Road, Cambridge CB2 1EZ, U.K. [fax: +44(0)-1223-366033 or e-mail: deposit@ccdc.cam.ac.uk].
14. The only report concerning cycloaddition of C,N-diphenylnitrone to dimethyl ethylidenemalonate was reported in 1973 (M. Joucla, J. Hamelin, and R. Carrie, Bull. Soc. Chim. Fr., 1973, 3116).
15. (a) J. P. Perdew, S. H. Chevary, K. A. Vosko, K. A. Jackson, M. R. Pederson, D. J. Singh, and C. Fiolhais, Phys. Rev. B, 1992, 46, 6671; CrossRef (b) J. P. Perdew, S. H. Chevary, K. A. Vosko, K. A. Jackson, M. R. Pederson, D. J. Singh, and C. Fiolhais, Phys. Rev. B, 1993, 48, 4978; CrossRef (c) J. P. Perdew, K. Burke, and Y. Wang, Phys. Rev. B, 1996, 54, 16533; CrossRef (d) C. Adamo and V. Barone, J. Chem. Phys., 1998, 108, 664; CrossRef It is proposed that the mPW1PW91 can account for the likely long-range dispersive interactions as well as hydrogen bonding (see: M. P. Patil and R. B. Sunoj, Chem. Asian J., 2009, 4, 714 and references therein). CrossRef
16. (a) V. Barone and M. Cossi, J. Phys. Chem. A, 1998, 102, 1995; CrossRef (b) M. Cossi, N. Rega, G. Scalmani, and V. Barone, J. Comp. Chem., 2003, 24, 669. CrossRef
17. .
18. A. E. Reed, L. A. Curtiss, and F. Weinhold, Chem. Rev., 1988, 88, 899. Natural charges on the carbon and nitrogen taking part in the double bond at the nitrone in Z-2 isomers were -0.02 and 0.12 a.u. respectively, for both conformations C1 and C2. In the case of Z-4 these values decreased to -0.01 and 0.10 a.u. CrossRef
19. We have also performed the NBO analysis of the nitrone lacking of the sulfinyl group. The charge localized at the C and N atoms (0.01 and 0.11 a.u. respectively) increase with respect to that determined for 2, which means that the presence of the sulfinyl group could be responsible of the decrease in the charge and could also be related to the observed reactivity. Indeed, the decrease in the charge at carbon could be related with the stabilizing interactions (kcal·mol-1), evaluated by means of a second-order perturbational analysis of the Fock matrix, between a lone pair of the sulfinyl oxygen and the C-H antibonding orbital (3.4 for both Z-2 and Z-4) in the case of C1, or between the lone pair of the sulfinyl sulphur and the aromatic ring (3.2 for both Z-2 and Z-4) and the retrodonation from the aromatic ring to the C nitrone-Cipso (3.6 for both Z-2 and Z-4) in the case of C2.
20. (a) Y. Arroyo, A. Meana, M. A. Sanz-Tejedor, I. Alonso, and J. L. Garcia Ruano, Chem. Eur. J., 2010, 16, 9874; CrossRef (b) J. L. Garcia-Ruano, C. Schoepping, C. Alvarado, and J. Aleman, Chem. Eur. J., 2010, 16, 8968; CrossRef (c) J. L. García Ruano, M. A. Fernandez-Ibañez, J. A. Fernandez-Salas, M. C. Maestro, P. Marquez-Lopez, and M. M. Rodriguez-Fernandez, J. Org. Chem., 2009, 74, 1200. CrossRef