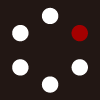
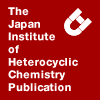
HETEROCYCLES
An International Journal for Reviews and Communications in Heterocyclic ChemistryWeb Edition ISSN: 1881-0942
Published online by The Japan Institute of Heterocyclic Chemistry
e-Journal
Full Text HTML
Received, 17th June, 2011, Accepted, 14th July, 2011, Published online, 21st July, 2011.
DOI: 10.3987/COM-11-12285
■ Synthesis of Fluorescent Pyrrolo[3,4-b]quinolizine Derivatives and Evaluation as a Protein-Labeling Probe
Masayori Hagimori, Naoko Mizuyama, Kenichirou Yokota, Osamu Morinaga, Yasuchika Yamaguchi, Hideo Saji, and Yoshinori Tominaga*
School of Pharmaceutical Sciences, Nagasaki University, 1-14, Bunkyo-machi, Nagasaki 852-8521, Japan
Abstract
New fluorescent compounds, pyrrolo[3,4-b]quinolizines, were synthesized in good yields via a one-pot method based on the condensation of functionalized maleimides (1a–c) with 2-pyridylacetonitrile (2a), and alkyl 2-pyridylacetates (2b, c). These pyrrolo[3,4-b]quinolizine derivatives emitted over a wide wavelength range in solution (Em max: 484–604 nm) and in the solid state (Em max: 534–640 nm). In addition, we evaluated the utility of these heterocycles as fluorescent labeling probes for BSA.Fluorescent dyes are widely used as emitting materials in various fields such as clinical diagnostics, and organic electroluminescent (OEL) devices.1 Although their practical applications have attracted attention, there remains some scope to improve the chemical, physical, and optical properties of their core skeletons. Thus, the discovery of new fluorescent core skeletons has become an important area of research in this field. One of our targets is to discover a new heterocyclic core structure that can emit light over a wide wavelength range both in solutions and in the solid state, by changing its substituent groups, since such examples are still limited.2 Quinolizines have attracted attention due to their potential applications as photographic materials and dyes, and were typically synthesized by the condensation of alkyl 2-pyridylacetates or 2-pyridylacetonitrile with (ethoxymethylene)malononitrile or 3,3-bis(methylsulfanyl)acrylonitriles.3 Recently, we described the synthesis of fluorescent quinolizine compounds such as pyrano[4,3-b]quinolizine3c and quinolizino[3,2-a]quinolizines.3e However, these derivatives do not show clear substitution effects in their emission wavelengths. In this paper, we report the synthesis of novel fused quinolizine derivatives, pyrrolo[3,4-b]quinolizines, by a one-pot synthesis method using functionalized maleimides (3-methylsufanyl-2,5-dioxo-1H-pyrroles), instead of using (ethoxymethylene)malononitrile or 3,3-bis(methylsulfanyl)acrylonitriles. Pyrrolo[3,4-b]quinolizines showed interesting fluorescent properties including substitution effects in solution and in the solid state. We also present preliminary results to demonstrate the potential application of this core skeleton in biochemical analysis.
Maleimides (1a–c) were prepared by the previously reported method.4,5 We carried out the condensation of 1a with 2-pyridylacetonitrile (2a) in the presence of potassium carbonate in DMSO. The reaction was conducted at room temperature for 2 h, stirred at 50–60 °C for 30 min, and finally, was returned to room temperature for an additional 2 h. The reaction solution was then neutralized with 10% hydrochloric acid, and the orange red product, 4-imino-2-methyl-1,3-dioxo-1,2,3,4-tetrahydropyrrolo[3,4-b]quinolizine-10-carbonitrile (3a),6 was obtained in 89% yield (Scheme 1). Scheme 2 illustrates the presumed reaction mechanism that forms 3a. The addition of 2a to maleimide (1a), followed by elimination of the methylsufanyl group, and subsequent cyclization gave compound 3a. In a similar manner, the ester derivatives 3b and 3c were easily prepared in 65% and 58% yields, respectively, from 1a with alkyl 2-pyridylacetates (2b, c). 1,3,4-Trioxo-pyrrolo[3,4-b]quinolizine derivatives (4a–c) were similarly prepared from 1b with 2a–c in 29–54% yields (Scheme 3, Method A).7 In these cases, neutralization by 10% hydrochloric acid was not needed. We directly obtained the desired products from the alkaline solution. Alternatively, the reaction of 1b with 2a–c, simply by heating the reagents at 200°C in the absence of solvent or catalyst gave 4a–c in 81–83% yield (Method B). Compound 5 was synthesized from 1c and 2b in the same way in which 4a–c (Method A) were synthesized. The decarboxylated derivative (6) was obtained via 4b treated with polyphosphoric acid (PPA) at 100 °C for 20 min in 84% yield.
We analyzed the fluorescence emission spectra of 3a–c, 4a–c, 5, and 6 in solution and in the solid state, at room temperature. The fluorescence emission maxima (Em max) and fluorescence quantum yields (Φ) in solution are listed in Table 1. In ethanol, all compounds exhibited blue fluorescence (Em max: 492–509 nm), and therefore we did not observe clear substitution effects. On the other hand, in dichloromethane, 3a–c showed a large bathochromic shift, and red fluorescence (Em max: 576–604 nm). This solvent effect may be related to the influence of the imino group at the 4-position of the pyrrolo[3,4-b]quinolizine skeleton on its fluorescent properties. With regard to the Φ values, 3a–c exhibited weaker fluorescence than 4a–c, 5, and 6 in both solvents. In the solid state, the Em max and Φ values of 3a–c, 4a–c, 5, and 6 ranged from 534 to 640 nm, and from 0.01 to 0.06, respectively. Among these compounds, 3a–c showed a pronounced bathochromic shift and light emission at 636–640 nm, which was much longer than that of DCM ([4-(dicyanomethylene)-2-methyl-6-(p-dimethylaminostyryl)-4H-pyran]), a red laser dye (Em max: 577 nm).1d The above fluorescent properties along with their efficient method of synthesis make pyrrolo[3,4-b]quinolizines a new and attractive core skeleton.
We next sought to evaluate the utility of the pyrrolo[3,4-b]quinolizine skeleton and prepared a bovine serum albumin (BSA) conjugate as a preliminary example. The synthesis of 9 is represented by scheme 4. Hydrolysis of 4b yielded 7, which was coupled with methyl 6-aminohexanoate·HCl to give 8. Subsequent hydrolysis of 8 afforded 9. The Em max and Φ values of 9 were 500 nm and 0.20, respectively. The conjugation 9 with BSA was conducted by the EDC (1-Ethyl-3-(3-dimethylaminopropyl)carbodiimide) method. MALDI-TOF mass spectrometry revealed that approximately 15 molecules of 9 conjugated with a single molecule of BSA. 9-BSA conjugate emitted at 512 nm in water. Figure 1 shows the standard plot of the 9-BSA conjugate fluorescence intensity variation with 9-BSA conjugate concentration. The linear range extended from 0.025 to 10 μg/mL and the detection limit of the conjugate 9-BSA was 0.01 μg/mL if the amount was applied directly (data not shown).
In conclusion, we have synthesized fluorescent pyrrolo[3,4-b]quinolizine derivatives (3a–c, 4a–c, 5, and 6) in good yields by a one-pot method based on the condensation of functionalized maleimides (1a–c) with 2-pyridylacetonitrile (2a), and alkyl 2-pyridylacetates (2b, c). Modification of functional groups on the pyrrolo[3,4-b]quinolizine skeleton can change the corresponding emission maxima. These derivatives emitted over a wide wavelength range in solution (Em max: 484–604 nm) and in the solid state (Em max: 534–640 nm). Further, we demonstrated the utility of the pyrrolo[3,4-b]quinolizine skeleton as a protein-labeling probe by the conjugation of 9 with BSA. Currently, we are evaluating the relationship between the structure and fluorescence properties of this new class of compounds and their biochemical applications.
References
1. a) D. W. Domaille, E. L. Que, and C. J. Chang, Nat. Chem. Biol., 2008, 4, 168; CrossRef b) M. S. Gonçalves, Chem. Rev., 2009, 109, 190; CrossRef c) C. W. Tang and S. A. Van Slyke, Appl. Phys. Lett., 1987, 51, 913; CrossRef d) C. W. Tang, S. A. Van Slyke, and C. H. Chen, J. Appl. Phy., 1989, 65, 3610; CrossRef e) M. A. Baldo, M. E. Thompson, and S. R. Forrest, Nature, 2000, 403, 750. CrossRef
2. L. D. Lavis and R. T. Raines, ACS Chem. Biol., 2008, 3, 142. CrossRef
3. a) H. Awaya, C. Maseda, Y. Tominaga, R. Natsuki, Y. Matsuda, and G. Kobayashi, Chem. Pharm. Bull., 1974, 22, 1424; b) H. Awaya, C. Maseda, Y. Tominaga, Y. Matsuda, and G. Kobayashi, Yakugaku Zasshi, 1975, 95, 13; c) M. Hagimori, N. Mizuyama, and Y. Tominaga, ARKIVOC, 2008, 13, 16; d) M. Hagimori, S. Matsui, N. Mizuyama, K. Yokota, J. Nagaoka, and Y. Tominaga, Eur. J. Org. Chem., 2009, 33, 5847; CrossRef e) K. Yokota, M. Hagimori, Y. Shigemitsu, N. Mizuyama, B. C. Wang, and Y. Tominaga, Heterocycles, 2009, 78, 1271; CrossRef f) Y. Tominaga, “Product Class 7, Cyclazines”, in “Science of Synthesis”, Vol. 17, ed. by S. M. Weinreb, Georg Thieme Verlag, Stuttgart, New York, pp. 1075, 2004.
4. a) Y. Tominaga and Y. Matsuda, J. Heterocycl. Chem., 1985, 22, 937; CrossRef b) Y. Tominaga and Y. Matsuda, J. Synth. Org. Chem. Jpn, 1985, 43, 669; CrossRef c) R. K. Dieter, Tetrahedron, 1986, 42, 3029; CrossRef d) Y. Tominaga, J. Synth. Org. Chem. Jpn, 1989, 47, 413; CrossRef e) Y. Tominaga, ‘Synthesis of Heterocyclic Compounds Using Ketene Dithioacetals’ in ‘Trends in Heterocyclic Chemistry’, ed. by J.Menon, Council of Scientific Research Integration, Research Trends, 1991, 2, 43.
5. a) Y. Tominaga, N. Yoshioka, and S. Kataoka, Heterocycles, 1996, 43, 1597; CrossRef b) Y. Tominaga, N. Yoshioka, H. Minematsu, and S. Kataoka, Heterocycles, 1996, 44, 85. CrossRef
6. T. Nakatani, Y. Tominaga, and K. Hiraoka, Nippon Gomu Kyokaishi, 2008, 81, 197. CrossRef
7. 4b: yellow crystals. mp 288–290 °C. IR (KBr, cm-1): 1770 (CO), 1727 (CO), 1603, 1432, 1225, 987. UV (EtOH) λ nm (log ε): 453 (3.94). 1H NMR (400 MHz, CDCl3) δ: 3.19 (s, 3H, NMe), 4.06 (s, 3H, OMe), 7.54 (dd, J = 5.9, 6.8 Hz, 1H, 7-H), 8.26 (d, J = 8.8 Hz, 1H, 9-H), 7.98 (dd, J = 7.3, 8.8 Hz, 1H, 8-H), 9.64 (d, J = 7.3 Hz, 1H, 6-H). 13C NMR (100 MHz, CDCl3) δ: 24.29, 53.40, 104.06, 119.36, 125.35, 131.60, 137.51, 138.70, 145.90, 150.70, 164.81, 165.39, 166.19. MS (EI) m/z: 287 (M++1, 19), 286 (M+, 100), 256 (10), 255 (70), 228 (11), 227 (32), 183 (11), 171 (12), 170 (11), 143 (27). Anal. Calcd for C14H10N2O5=286.0590: C, 58.74; H, 3.52; N, 9.79. Found: C, 58.60; H, 3.42; N, 10.04.