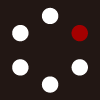
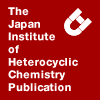
HETEROCYCLES
An International Journal for Reviews and Communications in Heterocyclic ChemistryWeb Edition ISSN: 1881-0942
Published online by The Japan Institute of Heterocyclic Chemistry
e-Journal
Full Text HTML
Received, 24th June, 2011, Accepted, 20th July, 2011, Published online, 21st July, 2011.
■ Practical Total Synthesis of Luotonin A by Intramolecular Double Hetero Diels-Alder Reaction
Ken Natsuki, Tadamichi Shindo, and Masahiro Toyota*
Department of Chemistry, Graduate School of Science, Osaka Prefecture University, 1-1 Gakuencho, Sakai, Osaka 593-8531, Japan
Abstract
An intramolecular double hetero Diels–Alder reaction of N-(2-cyanoquinolin-3-ylmethyl)-2-tosyloxybenzamide (3c) provided luotonin A (2) in 82% yield. Surprisingly, N-(2-bromoquinolin-3-ylmethyl)-2-tosyloxybenzamide (4) directly provided luotonin A (2) in 78% yield in the presence of Pd2(dba)3 (5 mol %), dppf (20 mol %), and K2CO3 (6 eq).We previously reported a concise total synthesis of luotonin A (2), isolated from Peganum nigellastrum by Nomura and colleagues in 1997.1 This synthetic approach employed an intramolecular double hetero Diels–Alder reaction of N-(2-cyanoquinolin-3-ylmethyl)-2-methoxybenzamide (1).2 Although the total synthesis required three steps, a yield of only 46% luotonin A (2) was afforded (Scheme 1).3
To further improve the yield of the key step, we examined the effects of various benzene ring substituents on the intramolecular double hetero Diels–Alder reaction.4 In this paper, we report several notable results of the above cycloaddition.
The substrates 3 were prepared as described previously.2 The products of the cycloaddition reaction are summarized in Table 1. Although the chloro-substituted 3a gave luotonine A (2) in only a 26% yield (entry 1), the bromo-substituted 3b produced 2 in a 73% yield (entry 2). Luotonin A (2) was obtained in a 62% yield in the absence of ZnCl2 (entry 3). This result suggests that a Lewis acid may not necessarily be required for the cycloaddition process. Fortunately, the tosyl-substituted 3c proved to be a suitable substrate for the intramolecular double hetero Diels–Alder reaction, and luotonin A (2) was afforded in 82% yield (entry 4).
Because a 17% yield of luotonin A (2) formed during the transformation of the bromide 4 into the cyanide 3c (entry 1), we searched for conditions that provided an additive effect, as shown in Table 2.3b When the coupling reaction was conducted in the presence of one equivalent of K2CO3, the yield of luotonin A (2) increased slightly (entry 2). Surprisingly, luotonin A (2) was provided in 78% yield when this reaction was performed in the presence of six equivalents of K2CO3 (entry 3). Investigations into the role of K2CO3 and the reaction mechanism underlying these reaction conditions are underway.
In conclusion, the intramolecular double hetero Diels–Alder reaction of N-(2-cyanoquinolin-3-ylmethyl)- 2-tosyloxybenzamide (3c) gave luotonin A (2) in 82% yield. Additionally, luotonin A (2) was obtained directly from N-(2-bromoquinolin-3-ylmethyl)-2-tosyloxybenzamide (4) under the coupling conditions.
EXPERIMENTAL
IR spectra were measured on a SHIMADZU FT-IR 8300 spectrophotometer. 1H NMR spectra were recorded on JEOL JX-500 (500 MHz) spectrometers with CHCl3 (δ 7.26) as an internal standard. 13C NMR spectra were recorded on JEOL JX-500 (125 MHz) spectrometer with CHCl3 (δ 77.16) as an internal standard. All compounds purified by chromatography were sufficiently pure (> 95% by 1H NMR analysis) for use in subsequent reactions.
Luotonin A (2). (Method A) A mixture of tosylate 3c (10.0 mg, 0.02 mmol), ZnCl2 (5.7 mg, 0.04 mmol), TMSCl (0.06 mL, 0.44 mmol) and Et3N (0.06 mL, 0.44 mmol) in DMF (5.0 mL) was heated at 150 °C in a stainless autoclave for 24 h. After removal of the solvent, saturated aqueous NH4Cl solution was added. The resulting mixture was extracted with EtOAc. The organic layer was washed with saturated aqueous NaCl solution, dried over MgSO4, and evaporated. Purification of the crude product by flash chromatography (Hexane:EtOAc=1:1) afforded luotonin A (2) (5.1 mg, 82%) as a pale yellow needle, mp 280-283 °C (lit.5 281-283 °C).
Data for luotonin A (2): 1H NMR (500 MHz, CDCl3) δ: 5.34 (s, 2H), 7.58 (ddd, J = 0.8, 7.2 and 7.2 Hz, 1H), 7.67 (ddd, J = 0.8, 7.2 and 7.2 Hz, 1H), 7.88 (ddd, J = 7.2, 7.2 and 1.2 Hz, 2H), 7.94 (d, J = 8.0 Hz, 1H), 8.11 (d, J = 8.0 Hz, 1H), 8.45 (dd, J = 8.0 and 1.2 Hz, 1H) and 8.41-8.47 (m, 2H); 13C NMR (125 MHz, CDCl3) δ: 160.8, 152.7, 151.3, 149.6, 149.5, 134.7, 131.7, 130.9, 130.8, 129.6, 129.0, 128.7, 128.7, 128.1, 127.6, 126.6, 121.5, and 47.5.
Luotonin A (2). (Method B) A mixture of bromide 4 (11.2 mg, 0.02 mmol), CuCN (7.9 mg, 0.09 mmol), Pd2(dba)3 (1.0 mg, 0.001 mmol), DPPF (2.5 mg, 0.004 mmol) and Et4NCN (5.2 mg, 0.03 mmol) in 1,4-dioxane (10 mL) was heated at 130 °C in a stainless autoclave for 4 h. The resulting mixture was diluted with EtOAc, and the precipitates were filtered off through Celite. The filtrate was washed with saturated aqueous NaHCO3 solution, saturated aqueous NaCl solution, dried over MgSO4, and evaporated. Purification of the crude product by flash chromatography (Hexane:EtOAc=1:1) afforded luotonin A (2) (4.9 mg, 78%) as a pale yellow needle.
References
1. Z.-Z. Ma, Y. Hano, T. Nomura, and Y.-J. Chen, Heterocycles, 1997, 46, 541. CrossRef
2. (a) M. Toyota, C. Komori, and M. Ihara, ARKIVOC, 2003, 15; (b) M. Toyota, C. Komori, and M. Ihara, Heterocycles, 2002, 56, 101. CrossRef
3. Recent total syntheses of luotonin A: (a) M.-C. Tseng, Y.-W. Chu, H.-P. Tsai, C.-M. Lin, J. Hwang, and Y.-H. Chu, Org. Lett., 2011, 13, 920; CrossRef (b) Y. Ju, F. F. Lu, and C. Li, Org. Lett., 2009, 11, 3582; CrossRef (c) Y. Liang, X. Jiang, and Z.-X. Yu, Org. Lett., 2009, 11, 5302; CrossRef (d) V. Sridharan, P. Ribelles, M. T. Ramos, and J. C. Menendez, J. Org. Chem., 2009, 74, 5715; CrossRef (e) K. Nacro, C. Zha, P. R. Guzzo, R. J. Herr, D. Peace, and T. D. Friedrich, Bioorg, Med. Chem., 2007, 15, 4237; CrossRef (f) H.-B. Zhou, G.-S. Liu, and Z.-J. Yao, J. Org. Chem., 2007, 72, 6270; CrossRef (g) H. Twin and R. A. Bately, Org. Lett., 2004, 69, 4563; (h) S. B. Mhaske and N. P. Argade, J. Org. Chem., 2004, 69, 4563; CrossRef (i) S. Dallavalle, L. Merlini, G. L. Beretta, S. Tinelli, and F. Zunino, Bioorg. Med. Chem. Lett., 2004, 14, 5757; CrossRef (j) A. Cagir, S. H. Jones, B. M. Eisenhauer, R. Gao, and S. M. Hecht, Bioorg. Med. Chem. Lett., 2004, 14, 2051; CrossRef (k) A. Cagir, B. M. Eisenhauer, R. Gao, S. J. Thomas, and S. M. Hecht, Bioorg. Med. Chem. Lett., 2004, 14, 6287; (l) S. P. Chavan and R. Sivappa, Tetrahedron Lett., 2003, 44, 1883; CrossRef (m) T. Harayama, A. Hori, G. Servan, Y. Morikami, T. Matsumoto, H. Abe, and Y. Takeuchi, Tetrahedron, 2004, 60, 10645; CrossRef (n) E. S. Lee, J.-G. Park, and Y. Jahng, Tetrahedron Lett., 2003, 44, 1883; CrossRef (o) D. Osborne and P. J. Stevenson, Tetrahedron Lett., 2002, 43, 5469; CrossRef (p) J. S. Yadav and B. V. S. Reddy, Tetrahedron Lett., 2002, 43, 1905; CrossRef (q) S. Dallavalle and L. Merlini, Tetrahedron Lett., 2002, 43, 1835. CrossRef
4. M. Toyota, C. Komori, and M. Ihara, Heterocycles, 2000, 52, 591; CrossRef For the reviews of cycloaddition reaction of 1-azadienes, see: (a) B. Groenendaal, E. Ruijter, and R. V. A. Orru, Chem. Commun., 2008, 5474; CrossRef (b) S. Jayakumar, M. P. S. Ishar, and M. P. Mahajan, Tetrahedron, 2002, 58, 379; CrossRef (c) M. Behforouz and M. Ahmadian, Tetrahedron, 2000, 56, 5259. CrossRef
5. Z.-Z. Ma, Y. Hano, T. Nomura, and Y.-J. Chen, Heterocycles, 1999, 51, 1593. CrossRef