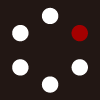
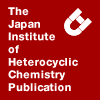
HETEROCYCLES
An International Journal for Reviews and Communications in Heterocyclic ChemistryWeb Edition ISSN: 1881-0942
Published online by The Japan Institute of Heterocyclic Chemistry
e-Journal
Full Text HTML
Received, 29th June, 2011, Accepted, 25th July, 2011, Published online, 1st August, 2011.
DOI: 10.3987/COM-11-S(P)62
■ Palladium-Catalyzed Selective and Sequential Functionalization of 2,4,6-Trihalopyridine Rings: Synthesis of Ethynylpyridine Polymers Directly Joined with Aza-Crown Ethers
Shunsuke Takashima, Tsuyoshi Yamamoto, Hajime Abe,* and Masahiko Inouye*
Graduate School of Pharmaceutical Sciences, University of Toyama, Sugitani 2630, Toyama, 930-0194, Japan
Abstract
meta-Ethynylpyridine polymer directly joined with 1-aza-18-crown-6 ether was synthesized by applying 2,4,6-trihalopyridines as a starting material. By using a Pd(OAc)2-BINAP combination with an excess of Cs2CO3, 2,6-dichloro-4-iodopyridine could be condensed with 1-aza-18-crown-6 ether to afford 4-azacrown-substituted 2,6-dichloropyridine selectively. The two chlorine atoms of this product were replaced with ethynyl groups by copper-free Sonogashira reaction with tert-butyldimethylsilylacetylene followed by protiodesilylation. Subsequent steps involving Sonogashira reactions gave diiodo- and diethynyl- substituted tripyridine units, and their co-polymerization yielded the targeted polymer.Crown ethers represent a class of the most commonly used host molecules, which can associate with a variety of cationic species through electrostatic interactions.1 This characteristic has attracted chemists to the development of molecular devices possessing crown ethers as a functional moiety.2,3 For example, Yashima et al. and Kakuchi et al. reported the helicity induction on polymers regulated by host-guest interaction between crown ethers and amino acids.3 Thus, an efficient synthetic strategy for introducing crown rings on organic frameworks is valuable in the progress of supramolecular chemistry.
During the course of our research, we have developed “meta-ethynylpyridine polymers”, which consist of 4-functionalized pyridine rings linked at their 2,6-positions with acetylene bonds.4 These polymers recognize various kinds of saccharide guests by multiple hydrogen bonds to form biassed helical higher-order structures. Functional groups on the pyridine units add extra properties such as amphiphilicity4c and strong basicity4b to the polymers. Recently, we have attained to introduce coordinating side chains on the polymer.4e,f Azacrown moieties could have been introduced indirectly on the polymer through the intervention by methylene groups that link between the azacrown and the pyridine rings. The obtained indirectly azacrown-induced polymers exhibited positive heteroallosteric saccharide recognition, in which oligoammonium axle molecules worked as an effector by pseudorotaxane formation with the azacrown rings. We thought that such heteroallosteric effects would be more effective by shortening the distance between azacrown moieties and pyridine rings. Thus, we designed 1-aza-18-crown-6-functionalized meta-ethynylpyridine co-polymer 2, in which the azacrown moieties are directly attached to the pyridine units in the main chain. The resulting higher-order structure would be more rigid and more regulable than that of 14e by the addition of cationic additives. To prepare the polymer, tri-substituted pyridine derivative 3 was supposed as a key building block that has one azacrown ring at the 4-position and two halogen atoms at the 2- and 6-positions (Figure 1). Thus, 1-aza-18-crown-6 ether must be regioselectively introduced on the 4-position of a pyridine ring. On the other hand, the two halogen atoms in 3 can be reaction points for the subsequent Sonogashira reactions. Herein, we describe the selective and sequential functionalization of 2,4,6-trihalopyridine 4c and the synthesis of the ethynylpyridine polymer 2.
Simple dialkylamino groups could have been introduced by nucleophilic aromatic substitution of the nitro group of 2,6-dibromo-4-nitropyridine (4a) with secondary amines and NaH.4b However, we failed in nucleophilic aromatic substitution on 4a and on 2,4,6-tribromopyridine (4b) with azacrown 5 under the presence of a strong base (Table 1, entries 1−4). The secondary amino group in 5 has only weak nucleophilicity because of the transannular steric hindrance and the coordination with the metal cations of the bases. Next, we attempted palladium-catalyzed amination methodologies. When non-nucleophilic strong base (LiHMDS) and highly active Pd complex PEPPSITM-IPr were applied,5 the azacrown moiety could be introduced on the pyridine ring of 4b (Table 1, entry 5). Unfortunately, not only the desired 4-substituted product but also undesired 2-substituted one was obtained as a difficultly separable mixture. Changing the reactivity of each halogen atom, we subjected 2,6-dichloro-4-iodopyridine (4c)6 to a similar amination. Although the combination of LiHMDS and PEPPSITM-IPr could not improve the selectivity
(Table 1, entry 6), the use of a Pd(OAc)2-BINAP combination with a large excess of Cs2CO37 did work, yielding 4-substituted product 3b as a sole product (entry 7).8 The use of alkali butoxides gave no coupling product (entries 8,9).
Subsequently, the remained two chlorine atoms were subjected to Sonogashira reaction. When Buchwald’s copper-free procedure9 was applied to 3b, bis(tert-butyldimethylsilylethynyl) product 8 could be obtained in 92% yield without disturbance by the bulky azacrown rings (Scheme 1).10 The co-polymer 2 was prepared as shown in Scheme 2. After protiodesilylation of 8, the obtained 9 was extended to co-trimeric diiodide 10 with the treatment of an excess of 2,6-diiodo-4-octyloxypyridine.11 Further diethynylation of 10 gave 12, which was used as the counterpart of 10 at the final step. The co-polymerization between the diiodide 10 and the diacetylene 12 was carried out by Sonogashira reaction as shown in Scheme 2 to give the co-polymer 2 (Mn = 120,000 g/mol vs polystyrene standards), which was identified on the basis of 1H NMR.12
In summary, we succeeded in preparing a new type of ethynylpyridine polymer that has 1-aza-18-crown-6 rings directly attached to the pyridine rings at the 4-positions. In that connection, we developed a selective and sequential functionalization of 2,4,6-trihalopyridine. The use of Pd(OAc)2 with BINAP and Cs2CO3 proved to selectively afford 4-azacrown-substituted 2,6-dichloropyridine in moderate yield. The remained two chlorine atoms could successively be alkynylated by Sonogashira reaction according to Buchwald’s copper-free procedure. The targeted polymer was obtained by the stepwise co-polymerization of the azacrown-attached pyridine units and the octyloxy-attached pyridine units by usual Sonogashira reactions. The co-polymer has potential as a host molecule, the higher-order structures of which could more effectively be regulated by the addition of guest cationic species. Such study about the saccharide recognition ability and heteroallosterism caused by the guest is underway.
References
1. (a) C. Pedersen, J. Am. Chem. Soc., 1967, 89, 2495; CrossRef (b) E. B. Kyba, K. Koga, L. R. Sousa, M. G. Siegel, and D. J. Cram, J. Am. Chem. Soc., 1973, 95, 2692. CrossRef
2. (a) S. Shinkai and O. Manabe, Top. Curr. Chem., 1984, 121, 67; (b) G. W. Gokel, W. M. Leevy, and M. E. Weber, Chem. Rev., 2004, 104, 2723; CrossRef (c) V. Balzani, M. Venturi, and A. Credi, Molecular Devices and Machines, Wiley-VCH, Weinheim, 2003; CrossRef (d) E. R. Kay, D. A. Leigh, and F. Zerbetto, Angew. Chem. Int. Ed., 2007, 46, 72; CrossRef (e) P. R. Ashton, E. J. T. Chrystal, P. T. Glink, S. Menzer, C. Schiavo, N. Spencer, J. F. Stoddart, P. A. Tasker, A. J. P. White, and D. J. Williams, Chem. Eur. J., 1996, 2, 709; CrossRef (f) S. J. Rowan and J. F. Stoddart, Polym. Adv. Technol., 2002, 13, 777; CrossRef (g) W. R. Dichtel, O. S. Miljanić, W. Zhang, J. M. Spruell, K. Patel, I. Aprahamian, J. R. Heath, and J. F. Stoddart, Acc. Res. Chem., 2008, 41, 1750; CrossRef (h) F. Huang and H. W. Gibson, Prog. Polym. Sci., 2005, 30, 982. CrossRef
3. (a) K. Morino, M. Oobo, and E. Yashima, Macromolecules, 2005, 38, 3461; CrossRef (b) T. Miyagawa, M. Yamamoto, R. Muraki, H. Onouchi, and E. Yashima, J. Am. Chem. Soc., 2007, 129, 3676; CrossRef (c) R. Sakai, T. Satoh, R. Kakuchi, H. Kaga, and T. Kakuchi, Macromolecules, 2003, 36, 3709; CrossRef (d) R. Kakuchi, R, Sakai, I. Otsuka, T. Satoh, H. Kaga, and T. Kakuchi, Macromolecules, 2005, 38, 9441. CrossRef
4. (a) M. Inouye, M. Waki, and H. Abe, J. Am. Chem. Soc., 2004, 126, 2022; CrossRef (b) H. Abe, N. Masuda, M. Waki, and M. Inouye, J. Am. Chem. Soc., 2005, 127, 16189; CrossRef (c) M. Waki, H. Abe, and M. Inouye, Chem. Eur. J., 2006, 12, 7839; CrossRef (d) M. Waki, H. Abe, and M. Inouye, Angew. Chem. Int. Ed., 2007, 46, 3059; CrossRef (e) H. Abe, S. Takashima, T. Yamamoto, and M. Inouye, Chem. Commun., 2009, 2121; CrossRef (f) S. Takashima, H. Abe, and M. Inouye, Chem. Commun., 2011, 47, 7455. CrossRef
5. M. G. Organ, M. Abdel-Hadi, S. Avola, I. Dubovyk, N. Hadei, E. A. B. Kantchev, C. J. O’Brien, M. Sayah, and C. Valente, Chem. Eur. J., 2008, 14, 2443. CrossRef
6. M. Schlosser, C. Bobbio, and T. Rausis, J. Org. Chem., 2005, 70, 2494. CrossRef
7. C. Meyers, B. U. W. Maes, K. T. J. Loones, G. Bal, G. L. F. Lemière, and R. A. Dommisse, J. Org. Chem., 2004, 69, 6010. CrossRef
8. Preparation of 3b. To a toluene (15 mL) solution of Pd(OAc)2 (14 mg, 60 µmol) and (±)-BINAP (37 mg, 60 µmol) were added 2,6-dichloro-4-iodopyridine (4c) (0.42 g, 1.6 mmol), 1-aza-18-crown-6 (0.48 g, 1.8 mmol), and Cs2CO3 (2.4 g, 7.5 mmol). The reaction mixture was stirred for 5 min at room temperature and 12 h under reflux. After cooling to room temperature, the resulting mixture was filtered. The filtrate was concentrated by a rotary evaporator and purified by silica gel column chromatography (eluent: CH2Cl2/MeOH = 97:3) to yield 3b (0.31 g, 49%) as a yellowish brown oil. IR (neat) νmax 2869, 1584, 1517 cm−1; 1H NMR (300 MHz, CDCl3) δ 6.52 (s, 2 H), 3.74−3.60 (m, 24 H); 13C NMR (75 MHz, CDCl3) δ 156.0, 150.5, 104.9, 70.9, 70.7, 70.6, 68.1, 51.1, 27.5; HRMS (ESI) Calcd for C17H27Cl2N2O5 (M + H+): 409.1297; Found: 409.1301.
9. D. Gelman and S. L. Buchwald, Angew. Chem. Int. Ed., 2003, 42, 5993.
. CrossRef
10. Preparation of 8. A mixture of 3b (3.0 g, 7.3 mmol), PdCl2(MeCN)2 (0.3 g, 1.2 mmol), ligand 7 (1.7 g, 3.5 mmol), and Cs2CO3 (12 g, 38 mmol) in MeCN (120 mL) was stirred for 30 min at room temperature, then tert-butyldimethylsilylacetylene (2.7 g, 19 mmol) was added to the mixture dropwise. The reaction mixture was stirred for 80 min under reflux. After cooling to room temperature, the resulting mixture was diluted with ether (200 mL) and filtered through a pad of Florisil. The filtrate was evaporated by a rotary evaporator and purified by silica gel column chromatography (eluent: CH2Cl2/MeOH = 97:3) to yield 8 (4.1 g, 92%) as a brown oil. IR (neat) νmax 2951, 2927, 2856, 2155, 1581, 1523 cm−1; 1H NMR (300 MHz, CDCl3) δ 6.68 (s, 2 H), 3.78−3.58 (m, 24 H), 0.98 (s, 18 H), 0.18 (s, 12 H); 13C NMR (75 MHz, CDCl3) δ 152.6, 143.0, 110.0, 105.1, 91.0, 70.8, 70.63, 70.58, 68.2, 50.4, 26.2, 16.7, −4.6; HRMS (ESI) Calcd for C33H57N2O5Si2 (M + H+): 617.3806; Found: 617.3834.
11. H. Abe, H. Machiguchi, S. Matsumoto, and M. Inouye, J. Org. Chem., 2008, 73, 4650.
. CrossRef
12. 1H NMR (300 MHz, CDCl3) δ 7.13 (br s, 4n H), 6.90 (br s, 2n H), 4.02 (br s, 4n H), 3.67 (br s, 24n H), 1.78 (br s, 4n H), 1.52−1.18 (m, 20n H), 0.90 (br s, 6n H).