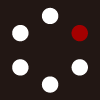
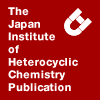
HETEROCYCLES
An International Journal for Reviews and Communications in Heterocyclic ChemistryWeb Edition ISSN: 1881-0942
Published online by The Japan Institute of Heterocyclic Chemistry
e-Journal
Full Text HTML
Received, 7th September, 2011, Accepted, 5th October, 2011, Published online, 7th October, 2011.
DOI: 10.3987/COM-11-S(P)98
■ A New Derivative of Galanthamine: Methylene Insertion into the Aromatic Ring in Place of Cyclopropanation
Péter Keglevich, Péter Kovács, László Hazai, Zsuzsanna Sánta, Zsófia Dubrovay, Viktor Háda, Csaba Szántay, Jr., György Kalaus, and Csaba Szántay*
Department of Organic Chemistry and Technology, Budapest University of Technology and Economics, H-1111 Budapest, Szt. Gellért tér 4, Hungary
Abstract
In the course of the reaction between galanthamine and diazomethane in the presence of a catalyst, such as palladium(II) acetate or copper(I) bromide, methylene insertion into the aromatic ring was observed instead of the expected cyclopropanation of the carbon-carbon double bond.INTRODUCTION
Galanthamine1 (1) is a member of the Amaryllidaceae alkaloids, isolated from the Caucasian snowdrop (Galanthus woronowii).2 Galanthamine is a butyrylcholinesterase and acetylcholinesterase inhibitor2,3 as well as an allosterically potentiating ligand of the neuronal nicotinic receptor,4 currently used for the treatment of Alzheimer’s disease.4,5
Many reports have described different synthetic routes to prepare galanthamine,1,6-13 and with systematic transformations of its cyclic structure a number of derivatives were synthesized; e.g. by changing the position of the nitrogen atom in the azepine d ring,14 building in a sulfone group in ring d in place of the nitrogen atom,15 and substituting the furan b ring with pyrrole.16 Derivatives without a furan b ring,17 compounds containing a nitrogen heteroatom in the aromatic ring a18 and galanthamine with an opened azepine d ring were also investigated.19 The last result in the chemistry of galanthamine was the synthesis of homogalanthamine (2) with a larger azepine ring.20
A study of the literature data presented above led us to the concept of attempting to change the c ring by considering the carbon-carbon double bond as a target. The saturated derivative of galanthamine is known as lycoramine2 and has a weaker biological effect, but the cyclopropano derivative (3) is unknown in the literature.
The cyclopropane subunit has always been considered as an electronically unique21,22 structural element not only in the chemistry of artificial molecular structures23 but in natural compounds as well.24-26 Synthetic methods for the cyclopropanation of carbon-carbon double bond were reviewed recently,27 the Simmons-Smith reaction,28,29 reaction with dimethyloxosulfonium methylide,30 and cyclopropanation with diazomethane31 in the presence of a catalyst are frequently used.
RESULTS AND DISCUSSION
In our first approach to the cyclopropanation reaction of galanthamine (1) we used the Simmons-Smith method by allowing 1 to react with diiodomethane28 in the presence of diethylzinc at 0 oC or using trifluoroacetic acid29 besides the aforementioned reagents at -15 oC. However, from these reactions only decomposition products were isolated. Secondly, a reaction between 1 and diazomethane generated from N-methyl-N-nitrosourea in dichloromethane solution in the presence of palladium(II) acetate31 at 0 oC resulted in a new derivative of galanthamine (Scheme 1). Cyclopropanation has not taken place but from the reaction mixture we could isolate a cycloheptatriene derivative (4) in 8% yield. Besides 4 a side-product with an open d ring (5) could also be detected.
The structure of 4 was determined from 1H, 13C, COSY, TOCSY, HSQC, HMBC and NOESY NMR spectra. The 1H NMR spectrum of 4 showed that, as compared to 1, the aromatic proton signals had disappeared and two new double-bond proton signals emerged. One of these protons (later assigned as H-7) showed only a cis type of vicinal 1H-1H coupling (9.7 Hz), but the other one (H-8) exhibited further vicinal couplings (8.1 and 6.3 Hz) with the geminal CH2 protons at 1.49 and 2.76 ppm (H2-9). The location of that double bond could be unambiguously determined from the 2D gHMBCAD spectrum which exhibited the following diagnostic two- or three-bond 1H-to-13C coupling correlations: 5.06 ppm (H-8) to 32.0 ppm (C-9) and 136.6 ppm (C-6); 6.09 ppm (H-7) to 32.0 ppm (C-9) and 145.5 ppm (C-5a); 1.49 ppm and 2.76 ppm (H2-9) to 59.9 ppm (C-10), 112.3 ppm (C-8), 123.0 ppm (C-7), 126.5 ppm (C-9a) and 134.1 ppm (C-9b). Moreover a strong positive homonuclear NOE was observed from the signal at 3.21 ppm (Hx-10) to that at 2.76 ppm (Hy-9). The other signals in the proton and carbon spectra showed the same pattern and correlations as in the starting material galanthamine indicating that only the a ring was transformed and other parts of the molecule remained unchanged in the reaction.
The formation of compound 4 occurs with insertion of a methylene group into the aromatic ring. The reaction is known in the literature and was mainly investigated in relatively simple aromatics to prepare tropylium salts with different substituents.32-35 (The formation of compound 5 can be explained with an oxidative ring opening of 1; N-formylation reaction with diazomethane is known in the literature.36)
According to the literature32 the Rh/C catalyst gives the best yields in these types of reaction and in some cases copper(I) halogenides, copper(I) chloride33 and copper(I) bromide34,35 were also effective. Using Rh/C catalyst no reaction was observed between 1 and diazomethane. Performing the reaction in the presence of copper(I) bromide, however, the cycloheptatriene derivative 4 was isolated in 13% yield. Replacing diazomethane with trimethylsilyldiazomethane37 proved to be unsuccessful.
Based on literature results38 a possible mechanism is presented in Scheme 2. Carbene formed from diazomethane attaches to the aromatic a ring (1a) producing the cycloheptatriene derivative (4) via a norcaradiene-type intermediate (1b).
Formation of an aromatic methyl derivative (1c) in this reaction is also possible, but no traces of this product were detected.
CONCLUSION
In summary, an attempted cyclopropanation of the carbon-carbon double bond in the c ring of galanthamine (1) with diazomethane resulted in a new derivative (4) with a cycloheptatriene structure in place of the aromatic a ring. Such a reaction with methylene insertion into an aromatic ring has not yet been described in the field of natural compounds.
EXPERIMENTAL
General procedures
Melting points are uncorrected. IR spectra were recorded on Zeiss IR 75 and 80 instruments. NMR measurements were performed on a Varian 800 MHz NMR spectrometer equipped with a 1H{13C/15N} Triple Resonance 13C Enhanced Salt Tolerant Cold Probe operating at 800 MHz for 1H and 201 MHz for 13C, and a Varian 500 MHz NMR spectrometer equipped with a 1H {13C/15N} 5 mm PFG Triple Resonance 13C Enhanced Cold Probe operating at 500 MHz for 1H and 125 MHz for 13C. Chemical shifts are given on the delta scale as parts per million (ppm) with tetramethylsilane (TMS) (1H) or dimethylsulfoxide-d6 (13C) as the internal standard (0.00 ppm and 39.5 ppm, respectively). 15N chemical shifts are reported relative to the internal reference of the spectrometer, set to nitromethane as the reference compound. 1H-1H, direct 1H-13C, and long-range 1H-13C scalar spin-spin connectivities were established from 2D gDQFCOSY, zTOCSY, gHSQCAD, and gHMBCAD experiments, respectively. All pulse sequences were applied by using the standard spectrometer software package. All experiments were performed at 298 K. High-resolution ESI-MS measurements were carried out on a Thermo LTQ FT Ultra mass spectrometer (3.1 kV source voltage, 280 °C capillary temperature, solvent: MeOH:H2O 1:1 + 1 V/V% cc. AcOH). The protonated molecular ion peaks were fragmented by CID at a normalized collision energy of 35-55%. The relative abundance values of the fragment ions in the MS-MS spectrum are given in brackets. TLC was carried out using Kieselgel 60F254 (Merck) glass plates.
(3R,4aS,13aS)-3-Hydroxy-6-methoxy-11-methyl-4,4a,10,11,12,13-hexahydro-3H,9H-11-azabenzofuro-[3a,3,2-ef]heptalene, 4
To a solution of 575 mg (2 mmol) of galanthamine (1) in CH2Cl2 (20 mL) containing 51 mg (0.23 mmol) of palladium(II) acetate, a solution of diazomethane (freshly prepared under the usual manner from 6 g (58.2 mmol) of N-methyl-N-nitrosourea) in CH2Cl2 (180 mL) was dropped in one hour period with stirring in an ice-water bath. Then the reaction mixture was stirred an hour under cooling and was filtered. The solvent was evaporated in vacuum, the crude product was purified by preparative thin-layer chromatography (Kieselgel, CH2Cl2-MeOH 9:1) and 46 mg (8%) of the product (4) was isolated as a pale yellow solid. Mp 143-145 oC. TLC (CH2Cl2-MeOH 10:1) Rf 0.5. [α]Hg54623 -175o (c 1.2, CH2Cl2). IR (KBr) 3529, 2923, 1655, 1626, 1550, 1408, 1293, 1235, 1114, 1045, 978, 927, 811 cm-1. 1H-NMR (500 MHz, DMSO-d6) δ 1.49 (dd, J= 12.5, J= 6.3 Hz, 1H, Hx-9), 1.62 (dd, J= 13.6, J= 6.6 Hz, 1H, Hx-13), 1.81 (td, J= 13.6, J= 7.0 Hz, 1H, Hy-13), 1.95 (ddd, J= 15.6, J= 5.8, J= 2.5 Hz, 1H, Hx-4), 2.23 (m, 1H, Hy-4), 2.27 (s, 3H, H3-N(11)Me), 2.36 (dd, J= 11.6, J= 6.6 Hz, 1H, Hx-12), 2.76 (dd, J= 12.5, J= 8.1 Hz, 1H, Hy-9), 2.76 (m, 1H, Hy-12), 3.21 and 3.47 (AB system, J= 18.4 Hz, 2H, H2-10), 3.54 (s, 3H, H3-OMe), 3.67 (d, J= 7.5 Hz, 1H, OH), 3.99 (m, 1H, H-3), 4.02 (br, 1H, H-4a), 5.06 (ddd, J= 9.7, J= 8.1, J= 6.3 Hz, 1H, H-8), 5.76 (dd, J= 10.3, J= 4.8 Hz, 1H, H-2), 5.96 (d, J= 10.3 Hz, 1H, H-1), 6.09 (d, J= 9.7 Hz, 1H, H-7) ppm. 13C-NMR (125 MHz, DMSO-d6) δ 29.7 (C-4), 32.0 (C-9), 33.5 (C-13), 46.3 (N-CH3), 47.6 (C-13a), 53.3 (C-12), 58.3 (OCH3), 59.9 (C-10), 60.2 (C-3), 82.2 (C-4a), 112.3 (C-8), 123.0 (C-7), 126.5 (C-9a), 128.3 (C-2), 129.3 (C-1), 134.1 (C-9b), 136.6 (C-6), 145.5 (C-5a) ppm. 15N-NMR (81 MHz, DMSO-d6) δ -347 (N-11) ppm. MS: MS-MS of m/z 302 (M+H): m/z 284(59), 253(5), 245(100), 227(4). M+H: m/z 302.17526, calculated value for C18H24O3N: 302.17507 (delta: 0.6 ppm).
In the case of using higher (three times) excess of diazomethane, a side product (5) was detected in the crude product by NMR, which, however, could not be isolated.
5: 1H-NMR (800 MHz, DMSO-d6) δ 1.45 (m, 1H, Hx-4), 1.87 (m, 1H, Hx-13), 2.03 (m, 1H, Hy-13), 2.19 (m, 1H, Hy-4), 2.63 (s, 3H, H3-NMe), 2.98 (m, 1H, Hx-12), 3.22 (m, 1H, Hy-12), 3.88 (s, 3H, H3-OMe), 4.12 (br, 1H, H-3), 4.88 (br, 1H, OH), 4.98 (dd, J=10.3, J= 5.1 Hz, 1H, H-4a), 5.80 (d, J=10.3 Hz, 1H, H-2), 6.29 (dd, J=10.3, J= 2.1 Hz, 1H, H-1), 7.13 (d, J=8.4 Hz, 1H, H-7), 7.53 (d, J=8.4 Hz, 1H, H-8), 7.85 (s, 1H, N(11)CHO), 9.92 (s, 1H, H-10) ppm. 13C-NMR (200 MHz, DMSO-d6) δ 28.8 (N(11)Me); 36.2 (C-4, C-13), 44.7 (C-12), 49.7 (C-13a), 55.9 (OMe), 62.2 (C-3), 83.9 (C-4a), 111.4 (C-7), 126.2 (C-9), 127.2 (C-1), 130.1 (C-8), 131.6 (C-9a), 134.1 (C-2), 147.1 (C-5a), 150.0 (C-6), 162.4 (N(11)CHO), 191.7 (C-10) ppm. 15N-NMR (81 MHz, DMSO-d6) δ -265 (N-11) ppm. MS: MS-MS of m/z 332 (M+H): m/z 314(63), 304(31), 300(23), 296(21), 286(100), 268(14). M+H: m/z 332.14944, calculated value for C18H22O5N: 332.14925 (delta: 0.6 ppm).
Performing the reaction presented above in the presence of 96 mg (0.67 mmol) of copper(I) bromide at room temperature, yield of the product (4) was 80 mg (13%).
References
1. J. Marco-Contelles, M. C. Carreiras, C. Rodriguez, M. Villaroya, and A. G. Garcia, Chem. Rev., 2006, 106, 116. CrossRef
2. P. J. Houghton, Y. Ren, and M.-J. Howes, Nat. Prod. Rep., 2006, 23, 181. CrossRef
3. S. Darwesh, R. Walsh, R. Kumar, A. Caines, S. Roberts, K. Rockwood, and E. Martin, Alzheimer Disease & Assoc. Disorders, 2003, 17, 117. CrossRef
4. A. Maeliczke, M. Samochocki, J. Jostock, A. Fehrenbacher, J. Ludwig, E. X. Albuquerque, and M. Zerlin, Biol. Psychiatry, 2001, 49, 279. CrossRef
5. Y. Ago, K. Koda, K. Takuma, and T. Matsuda, J. Pharmacol. Sci., 2011, 116, 6. CrossRef
6. L. Czollner, W. Frantsist, B. Küenburg, U. Hedenig, J. Fröhlich, and U. Jordis, Tetrahedron Lett., 1988, 39, 2087. CrossRef
7. B. M. Trost, W. Tang, and F. D. Toste, J. Am. Chem. Soc., 2005, 127, 14785. CrossRef
8. M. Node, S. Kodama, Y. Hamashima, T. Katoh, K. Nishide, and T. Kajimoto, Chem. Pharm. Bull., 2006, 54, 1662. CrossRef
9. T. Ishikawa, K. Kudo, K. Kuroyabu, S. Uchida, T. Kudoh, and S. Saito, J. Org. Chem., 2008, 73, 7498. CrossRef
10. P. Magnus, N. Sane, B. P. Fauber, and V. Lynch, J. Am. Chem. Soc., 2009, 131, 16045. CrossRef
11. J. K. Chang, H.-U. Kang, I.-H. Jung, and C.-G. Cho, Org. Lett., 2010, 12, 2016. CrossRef
12. T. Kato, H. Tanimoto, H. Yamada, and N. Chida, Heterocycles, 2010, 82, 563. CrossRef
13. P. Chen, X. Bao, L.-F. Zhang, M. Ding, X.-J. Han, J. Li, G.-B. Zhang, Y.-Q. Tu, and C.-A. Fan, Angew. Chem. Int. Ed., 2011, 50, 8161. CrossRef
14. A. H. Lewin, J. Szewczyk, J. W. Wilson, and F. I. Carroll, Tetrahedron, 2005, 61, 7144. CrossRef
15. M. J. Treu, U. Jordis, and K. Mereiter, Heterocycles, 2001, 55, 1727. CrossRef
16. C. Guillou, C. Bernard, and J.-L. Gras, WO 2002/102803, 2002 (Chem. Abstr., 2002, 138, 39446).
17. S. Vanlaer, W. M. De Borggraeve, and F. Compernolle, Eur. J. Org. Chem., 2007, 4995. CrossRef
18. S. Vanlaer, W. M. De Borggraeve, A. Voet, C. Gielens, M. De Maeyer, and F. Compernolle, Eur. J. Org. Chem., 2008, 2571. CrossRef
19. D. Herlem, M.-T. Martin, C. Thal, and C. Guillou, Biorg. Med. Chem. Lett., 2003, 13, 2389. CrossRef
20. N. Yamamoto, H. Fujii, S. Imaide, S. Hirayama, T. Nemoto, J. Inokoshi, H. Tomoda, and H. Nagase, J. Org. Chem., 2011, 76, 2257. CrossRef
21. F. J. Weigert and J. D. Roberts, J. Am. Chem. Soc., 1967, 89, 5962. CrossRef
22. K. B. Wiberg, Acc. Chem. Res., 1996, 29, 229. CrossRef
23. H. Lebel, J.-F. Marcoux, C. Molinaro, and A. B. Charette, Chem. Rev., 2003, 103, 977. CrossRef
24. R. Faust, Angew. Chem. Int. Ed., 2001, 40, 2251. CrossRef
25. L. A. Wessjohann and W. Brandt, Chem. Rev., 2003, 103, 1625. CrossRef
26. K. Yoshida, K. Yamaguchi, A. Mizuno, Y. Unno, A. Asai, T. Sone, H. Yokosawa, A. Matsuda, M. Arisawa, and S. Shuto, Org. Biomol. Chem., 2009, 7, 1868. CrossRef
27. W. A. Donaldson, Tetrahedron, 2001, 57, 8589. CrossRef
28. M. Tori, T. Hamaguchi, M. Aoki, M. Sono, and Y. Asakawa, Canad. J. Chem., 1997, 75, 634. CrossRef
29. J. C. Lorenz, J. Long, Z. Yang, S. Xue, Y. Xie, and Y. Shi, J. Org. Chem., 2004, 69, 327. CrossRef
30. I. Moldvai, E. Gács-Baitz, M. Balázs, M. Incze, and Cs. Szántay, Arch. Pharm. Pharm. Med. Chem., 1996, 329, 541. CrossRef
31. M. Incze, G. Dörnyei, I. Moldvai, E. Temesvári-Major, O. Egyed, and Cs. Szántay, Tetrahedron, 2008, 64, 2924. CrossRef
32. U. M. Dzhemilev, V. A. Dokichev, S. Z. Sultanov, R. A. Sadykov, G. A. Tolstikov, and O. M. Nefedov, Bull. Acad. Sci. USSR, Div. Chem. Sci. (Eng. Tran.), 1991, 40, 945.
33. R. P. Thummel and P. Chayangkoon, J. Org. Chem., 1983, 48, 596. CrossRef
34. E. Müller, H. Kessler, H. Fricke, and W. Kiedaisch, Liebigs Ann. Chem., 1964, 675, 63. CrossRef
35. K. Komatsu, K. Takeuchi, M. Arima, Y. Waki, S. Shirai, and K. Okamoto, Bull. Chem. Soc. Jpn., 1982, 55, 3257. CrossRef
36. A. R. Katritzky and S. Musierowicz, J. Chem. Soc. (C), 1966, 78. CrossRef
37. N. Hashimoto, T. Aoyama, and T. Shioiri, Chem. Pharm. Bull., 1981, 29, 1475; T. Aoyama, S. Terasawa, K. Sudo, and T. Shioiri, Chem. Pharm. Bull., 1984, 32, 3759.
38. N. Hartz, G. K. Surya Prakash, and G. A. Olah, J. Am. Chem. Soc., 1993, 115, 901. CrossRef