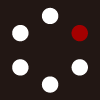
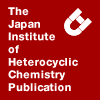
HETEROCYCLES
An International Journal for Reviews and Communications in Heterocyclic ChemistryWeb Edition ISSN: 1881-0942
Published online by The Japan Institute of Heterocyclic Chemistry
e-Journal
Full Text HTML
Received, 10th October, 2011, Accepted, 26th December, 2011, Published online, 10th January, 2012.
DOI: 10.3987/COM-11-12372
■ Scope and Limitations of a Modified Hantzsch Reaction for the Synthesis of Oxazole-Dehydroamino Acid Derivatives from Dehydroamino Acid Amides
Akihiro Nagaya, Yoji Yamagishi, Yasuchika Yonezawa, Shoji Akai,* Chung-gi Shin, and Ken-ichi Sato*
Department of Material & Life Chemistry, Faculty of Engineering, Kanagawa University, 3-27-1 Rokkakubashi, Kanagawa-ku, Yokohama 221-8686, Japan
Abstract
A variety of oxazole derivatives that possess an α,β-unsaturated substituent at the 2-position were conveniently synthesized in good yields via a Hantzsch-type reaction between dehydroamino acid amides and β-bromopyruvate derivatives. Furthermore, oxazoles with substituents at the 2- and 5-positions were also obtained in good yields using the corresponding β-substituted β-bromopyruvate derivatives. A revised reaction mechanism to explain the enhanced reactivity of dehydroamino acid amides for the Hantzsch-oxazole-type reaction is presented.INTRODUCTION
The synthesis of oxazole–containing bioactive natural products1 has been an important subject over the last two decades. Among the various synthetic routes for the oxazole ring system that have been reported,2-4 a popular strategy involves the heterocyclization of a serine or threonine side chain onto a dehydroamino acid amide carbonyl group to give an oxazoline that is subsequently oxidized to the oxazole (Scheme 1). Alternatively, thiazole building blocks of natural products can be conveniently synthesized via a modified Hantzsch reaction of thioamides that are derived from α- amino acids with β-halopyruvate (Scheme 2).5
However, the application of such conditions on the corresponding acid amides derived from saturated α-amino acids only gave the desired oxazole derivatives in moderate yields.4a,b The differences in the reactivities are presumably due to the nucleophilic properties of oxygen and sulfur. Therefore, we decided to develop a general synthetic methodology for preparing oxazole building blocks via Hantzsch-type reactions of acid amide derivatives that possess conjugated carbonyl oxygens with enhanced nucleophilicity. In 1996, Panek and co-workers reported on the efficient reaction of cinnamic acid amide with ethyl β-bromopyruvate to give the corresponding oxazole derivative,6a which was further converted to the tris-oxazole derivative in high yield.6b A similar type of oxazole synthesis based on the Hantzsch thiazole synthesis was reported by Bagley7 and Hoffman.8 Unfortunately, the enhanced reactivities and limitations of this special case of the Hantzsch protocol were not discussed. Herein, we describe the scope and limitations of the Hantzsch-oxazole protocol for synthesizing 2,4- and 2,4,5-substituted oxazole derivatives (having a dehydroamino substituent at the 2-position) from dehydroamino acid amide derivatives obtained from N-protected dehydroamino acids9 and methyl or ethyl β-bromopyruvate derivatives (with or without a β-substituent).
RESULTS AND DISCUSSION
We initially examined the reaction conditions for the Hantzsch-type reaction between a dehydroamino acid amide (2a)9 and ethyl β-bromopyruvate (3a)10 towards the synthesis of oxazole 1a. Favorable results were obtained with moderate temperatures and the use of a mild inorganic base. As shown in Table 1, optimal reaction conditions involved THF under reflux with NaHCO3 as a base (entry 5). Reactions with NaHCO3 and K2CO3 at room temperature did not proceed (entry 3 and 4). Furthermore, under homogeneous conditions (entries 1 and 2), 3a rapidly decomposed, even at rt, such that the reaction did not occur. Reactions at higher or lower temperatures, or in the presence of an organic base (homogeneous conditions) were not fruitful. In contrast, 2a was relatively stable at higher temperatures. Our results strongly indicated that both the reaction rate and the decomposition rate of 3a required careful consideration.
Consequently, the optimal reaction conditions shown in entry 5, Table 1 were employed to explore the scope and limitations of the reaction between 3a and various β-substituted dehydroamino acid amides (derivatives of 2a) (Table 2). The reactions were allowed to proceed until the disappearance of substrates 2 (as determined by TLC) to maximize the yields of 1. In some cases, due to the instability of 3a (see above), extra portions of 3a (2 equiv) were added after 5 and 10 h. Longer reaction times (over 5 h) were required in many cases, which can be attributed to the steric hindrance between the bulky substituents of the substrates 2 and β-bromopyruvate derivative 3, with the exception of entry 6. Interestingly, the reactivities of the methoxy (entry 6, enhanced reactivity) and nitro (entry 7, reduced reactivity) derivatives, compared to that of entry 5 in Table 1. These results may suggested that the reaction rate decrease with the increasing electron-withdrawing effect of substituents R1 (or/and R2) such as NO2, CO2Me on the dehydroamino acid amide. (Relative reaction rate for substituents: Me > Ph; Me > CH2CO2Me; p-MeO-Ph > Ph > p-NO2-Ph). Futhermore, this Hantzsch-type reaction of N-Cbz-L-alanine amide11 did not proceed (Scheme 3). Therefore, the conjugated double bond seems to play an important role in our Hantzsch-type reaction. Overall, oxazole-dehydroamino acid derivatives 1a-i were conveniently synthesized in good yields via the Hantzsch-type reaction, but with careful attention to the conditions. As shown in Table 2, satisfactory yields and reaction times were observed for entries 1, 2, and 6.
Subsequently, the Hantzsch-type reaction was applied towards the synthesis of a 5-substituted oxazole backbone, which is found in various natural products. As shown in Table 2, we first investigated the synthesis of 5-substituted oxazole dehydroamino acid derivatives using methyl β-substituted β-bromopyruvates (3b-d), which were readily prepared from the dehydroamino acids.12 Methyl β-substituted β-bromopyruvates 3b-d were reacted with dehydroamino acid amide 2a to give the corresponding oxazole derivatives in moderate yields. The influence of steric hindrance in the SN2 reactions was evident from the long reaction times required for 3b (entry 10) and 3c (entry 11). In contrast to the unsubstituted substrate, the methyl β-substituted β-bromopyruvates were relatively stable. In the case of entry 12, the faster reaction rate can be explained by the enhanced SN2 reaction at the benzylic position of 3d. It is generally known that SN2 reactions of halogenated substrates that involve either an allyl or benzyl group at the reaction center are enhanced by the stable π− π conjugation between the sp2 transition state and the π-electrons of the double bond. To improve the speed of the oxazole formation, these reactions were repeated under microwave irradiation at reflux conditions using a microwave apparatus.13,14 As shown in Table 2, improved yields and reaction times were obtained in all cases under such conditions.
Newly proposed reaction mechanisms are shown in Scheme 3 to explain the enhanced reactivities of the Hantzsch-type reactions towards oxazole synthesis. The mechanism with the resonance contributor [A] is inferred from Babadjamian and co-workers’ report15 for the modified Hantzsch reaction of saturated amino thioacid amide derivatives to give the corresponding thiazole derivatives. An alternate mechanism with the consideration of resonance contributor [B], which involves stabilized resonance hybrid oxyanion intermediates, might support the enhanced reactivities.
The reaction mechanism was further investigated using configurational isomers, as shown in Scheme 4. Because of the difficulty of preparing both (E)- and (Z)-isomers of the dehydroamino esters, the (E)- and (Z)-isomers of the α,β-unsaturated acid amides [2-methylbutenamide (2j and 2k)]16 were synthesized first as model compounds in good yields from the corresponding commercially available (E)- and (Z)-acids by treatment with DCC (N,N-dicyclohexylcarbodiimide) and HOSu (N-hydroxysuccinimide) in aqueous NH3 solution. These olefins apparently do not undergo isomerization because the corresponding products were exclusively obtained.16 To prepare such substrates 2a-i, three established methods, such as (1) β-elimination (anti-elimination) of Ser, Thr, and Cys,9 (2) condensation of α-ketoester and protected amine (CbzNH2),9,17 (3) Wittig reaction of N-protected diethylphospholylglycine ester to the corresponding aldehyde, and subsequent amidation,9,17 were employed. It is known that these reactions predominantly give (Z)-dehydroamino esters. The preference for the (Z)-isomer may be attributed to the thermodynamic stability of the configuration by a similar isomerization mechanism via the sp2 carbocation resonance contributor [B] as shown in Scheme 4. If the subsequent Hantzsch-type reaction proceeds with the resonance contributor [A] without participation of resonance contributor [B], the configuration of R1 and R2 (E, Z) will be retained in the products (4e and 4f),18 whereas if the reaction proceeds via resonance contributor [B], the configuration will be influenced (scrambled) by the sp2 intermediate to give the thermodynamically stable products. Our results show that both starting isomers afforded oxazole products with similar ratios between the (E)- and (Z)-isomers. In other words, the reaction proceeded via resonance contributor [B] with isomerization. The configurations of 4e and 4f were determined using NMR experiments (1H and NOESY) and the results were compared to reported spectral data.18 Other possibilities still remain, for example, that isomerization occurs via Michael addition – elimination reactions of oxazolines or oxazoles. However this mechanism cannot explain the enhanced reactivities.
Although the configurations of the oxazole-dehydroamino acid derivative products 1a-i have yet to be established by NMR and X-ray structure analyses, because of the absence of critical NOE correlations and the difficulties in getting single crystals, the stable configurations of the starting dehydroamino esters may be retained by deducing from the reaction mechanistic study using 4e and 4f (Scheme 5). The preference for the (Z)-isomer may also be in good agreement with the thermodynamically stable configuration of the corresponding starting dehydroamino ester by the mechanism via resonance contributor [B].
In conclusion, various oxazole-dehydroamino acid derivatives were efficiently synthesized from β-bromopyruvates and dehydroamino acid amides (easily derived from the corresponding dehydroamino acids) via a Hantzsch-type reaction with microwave irradiation. Using β-substituted β-bromopyruvates, this methodology was applied towards the synthesis of various 5-substituted oxazole derivatives, which can be found in the structures of various natural products.4d,19 Additional investigations indicated that the reaction mechanism of our Hantzsch-type reaction, due to the contribution of the α,β-unsaturated bond on the dehydroamino moiety, might differ from that of the modified Hantzsch reaction in thiazole synthesis. Because the synthesized oxazole-dehydroamino ester intermediate itself can serve as an activated substrate, it is our belief that this Hantzsch-type oxazole synthetic methodology can contribute towards the synthesis of various natural products that possess substituted mono-, bis-, and tris-oxazole moieties.
EXPERIMENTAL
All melting points were measured using a Yanaco Model MP-J3 micro-melting point apparatus, and are uncorrected. IR spectra were recorded using a Jasco FT/IR-4200 spectrometer in KBr. 1H NMR spectra were recorded on spectrometers operating at 500 MHz and 600 MHz in chloroform-d or dimethylsulfoxide-d6 referenced to tetramethylsilane (0.00 ppm). 13C NMR spectra were recorded on spectrometers operating at 125 MHz and 150 MHz in chloroform-d or dimethylsulfoxide-d6. The assignments of various NMR spectra were assisted by homonuclear (1H/1H) correlation spectroscopy (COSY), nuclear Overhuser and exchange spectroscopy (NOESY), nuclear Overhauser effect (NOE), and/or heteronuclear (1H/13C) correlation spectroscopy (HETCOR) experiments. The chemical shifts, coupling constants, and IR frequencies were recorded in δ, Hz, and cm-1 units, respectively. Column chromatography was performed on silica gel (Silica gel 60, 70-230 mesh; Silica gel 60N, spherical, neutral, 70-230 mesh). Thin-layer chromatography (TLC) on Silica gel 60F254 was used to monitor the reactions and to certify the purity of the reaction products by heating after spraying with ninhydrin-ethanol solution, phosphomolybdic acid ethanol-H2SO4 solution, and cerium ammonium molybdate water-H2SO4 solution.
Microwave Irradiation Experiments. All microwave experiments were performed with the Wave Magic®(EYELA MWO-1000S). Experiments were carried out in standard microwave process with Pyrex flask. Reaction time reflects irradiation times at the set reaction temperature (fixed hold times). Reaction temperature was measured using a teflon-coated thermocouple of type K.
General procedure for the reaction of dehydroamino acid amides (2a-i) and ethyl β-bromopyruvate (3a)
Thermal reaction: To a stirred solution of compound 2a-i (0.8 mmol) in THF (5 mL) were added NaHCO3 (336 mg, 5 equiv) and a solution of ethyl β-bromopyruvate (3a) (312 mg, 2 equiv) at 0 °C. After stirring for 5-11 h at reflux, the resulting solution was diluted with CHCl3 (10 mL) and organic layer was washed with brine and water (10 mL), dried over anhydrous MgSO4, concentrated in vacuo to give a yellow syrup as crude oxazoline. Crude oxazoline was dissolved in THF (5 mL), TFAA (trifluoroacetic anhydride) (333 µL, 3 equiv) and pyridine (388 µL, 6 equiv) were added to the above THF solution with stirring at 0 °C for 30 min. After the disappearance of oxazoline on TLC, the reaction mixture was concentrated in vacuo to give a brown syrup. Resulting residue was further dissolved in EtOAc (5 mL), 28% NH4OH (1 mL) was added to the mixture and stirred at 0 °C for 15 min. The reaction mixture was poured into brine, washed with water, brine, dried over anhydrous MgSO4, concentrated in vacuo to give oxazole-dehydroamino acid derivatives 1a-i (69-89%), which were purified on a silica gel column with hexanes-EtOAc.
Microwave assisted reaction: To a stirred solution of 2a-i (0.8 mmol) in THF (5 mL) were added NaHCO3 (336 mg, 5 equiv) and a solution of ethyl β-bromopyruvate (3a) (312 mg, 2 equiv) in THF (1 mL) at 0 °C. The flask was flushed with argon, heated with stirring at reflux under microwave irradiation for 5-72 min. After cooling to rt, the reaction mixture was diluted with CHCl3 (10 mL) and the organic layer was washed with brine and water, dried over anhydrous MgSO4, and concentrated in vacuo to give a yellow syrup. Resulting syrup was dissolved in THF (5 mL), TFAA (333 µL, 3 equiv) and pyridine (388 µL, 6 equiv) were added to the mixture at 0 °C, and stirred for 30 min. The reaction mixture was concentrated in vacuo to give brown syrup. Resulting syrup was further dissolved in EtOAc (5 mL), 28% NH4OH (1 mL) was added to the above mixture at 0 °C, and stirred for 15 min. Reaction mixture was poured into brine, washed with brine and water, dried over anhydrous MgSO4, concentrated in vacuo. Resulting brown syrup was purified on a silica gel column to give 5-substituted oxazole derivatives 1a-i (66-91%).
Ethyl (Z)-2-[1-((1-(Benzyloxy)carbonyl)amino)prop-1-en-1-yl]oxazole-4-carboxylate (Cbz-ΔAbu-Ozl-OEt) (1a): Yield 85%; white solid; mp 95-97 °C (acetone-hexanes); IR (KBr, disk) νmax 3302, 2959, 1732, 1505, 1313 cm-1; 1H NMR (600 MHz, CDCl3) δ 1.37 (3H, t, J = 7.2 Hz, Et’s CH3), 1.67 (3H, d, J = 7.2 Hz, ΔAbu’s CH3), 4.39 (2H, q, J = 7.2 Hz, Et’s CH2), 5.16 (2H, s, Cbz’s CH2), 6.48 (1H, br s, NH), 6.62 (1H, q, J = 7.2 Hz, vinyl’s H), 7.33-7.39 (5H, m, Cbz’s PhH), 8.12 (1H, s, ring-H); 13C NMR (150 MHz, CDCl3) δ 14.3, 61.3, 67.5, 67.5, 122.8, 128.2, 128.3, 128.5, 134.1, 135.9, 143.7, 153.9, 160.5, 161.1. HRMS (ESI-TOF) m/z: [M+Na]+ calcd for C17H18N2NaO5 353.1113, found 353.1095. Anal. Calcd for C17H18N2O5 (330.12): C, 61.81; H, 5.49; N, 8.48. Found: C, 61.74; H, 5.30; N, 8.21.
Ethyl (Z)-2-[1-(((tert-Butoxy)carbonyl)amino)prop-1-en-1-yl]oxazole-4-carboxylate (Boc-ΔAbu-Ozl-OEt) (1b): Yield 83%; white solid; mp 130.5-132 °C (acetone-hexanes); IR (KBr, disk) νmax 3322, 2980, 1724, 1498, 1317 cm-1; 1H NMR (600 MHz, CDCl3) δ 1.38 (3H, t, J = 7.1 00Hz, Et’s CH3), 1.47 (9H, s, Boc’s t-Bu), 1.87 (3H, d, J = 7.2 Hz, ΔAbu’s CH3), 4.39 (2H, q, J = 7.1 Hz, Et’s CH2), 6.26 (1H, br s, NH), 6.55 (1H, q, J = 7.2 Hz, vinyl’s H), 8.14 (1H, s, ring-H); 13C NMR (150 MHz, CDCl3) δ 14.1, 14.2, 28.1, 61.3, 80.6, 123.0, 127.1, 134.0, 143.5, 152.9, 160.8, 161.2. HRMS (ESI-TOF) m/z: [M+Na]+ calcd for C14H20N2NaO5 319.1270, found 319.1256. Anal. Calcd for C14H20N2O5 (296.14): C, 56.75; H, 6.80; N, 9.45. Found: C, 56.71; H, 6.58; N, 9.29.
Ethyl (Z)-2-[1-(((Benzyloxy)carbonyl)amino)-2-methylprop-1-en-1-yl]oxazole-4-carboxylate (Cbz-ΔVal-Ozl-OEt) (1c): Yield 85%; white solid; mp 108-109 °C (acetone-hexanes); IR (KBr, disk) νmax 3160, 2981, 1733, 1506, 1317 cm-1; 1H NMR (600 MHz, DMSO-d6 at 60 °C) δ 1.29 (3H, t, J = 7.1 Hz, Et’s CH3), 1.86, 2.10 (3H × 2, each s, ΔVal’s CH3 × 2), 4.29 (2H, q, J = 7.1 Hz, Et’s CH2), 5.05 (2H, s, Cbz’s CH2), 7.31-7.36 (5H, m, Cbz’s PhH), 8.66 (1H, bs, NH), 8.80 (1H, s, ring-H); 13C NMR (150 MHz, DMSO-d6, 60 °C) δ 13.9, 20.4, 20.7, 60.2, 65.5, 117.5, 127.3, 127.5, 128.0, 132.7, 136.6, 138.8, 143.7, 154.3, 159.9, 160.4. HRMS (ESI-TOF) m/z: [M+Na]+ calcd for C18H20N2NaO5 367.1270, found 367.1251. Anal. Calcd for C18H20N2O5 (344.14): C, 62.78; H, 5.85; N, 8.13. Found: C, 62.44; H, 5.47; N, 7.84.
Ethyl (Z)-2-[1-(((Benzyloxy)carbonyl)amino)-2,3-dimethylbut-1-en-1-yl]oxazole-4-carboxylate (Cbz-ΔLue-Ozl-OEt) (1d): Yield 88%; yellow syrup; IR (KBr, neat) νmax 3305, 2962, 1730, 1500, 1320 cm-1; 1H NMR (600 MHz, CDCl3) δ 1.08 (6H, d, J = 6.6 Hz, ΔLeu’s CH3), 1.38 (3H, t, J = 7.2 Hz, Et’s CH3), 2.78 (1H, dqq, J = 10.2, 6.6, 6.6 Hz, ΔLeu’s H), 4.39 (2H, q, J = 7.2 Hz, Et’s CH3), 5.15 (2H, s, Cbz’s CH2), 6.27 (1H, bs, NH), 6.39 (1H, d, J = 10.2 Hz, vinyl’s H), 7.26-7.36 (5H, m, Cbz’s PhH), 8.11 (1H, s, ring-H); 13C NMR (150 MHz, CDCl3) δ 14.7, 22.3, 27.9, 61.7, 67.9, 120.4, 128.6, 128.6, 128.9, 134.6, 136.3, 141.3, 144.0, 155.0, 161.1, 161.6. HRMS (ESI-TOF) m/z: [M+Na]+ calcd for C19H22N2NaO5 381.1426, found 381.1395.
Ethyl (Z)-2-[1-(((Benzyloxy)carbonyl)amino)-2-phenylethen-1-yl]oxazole-4-carboxylate (Cbz-ΔPhe-Ozl-OEt) (1e): Yield 88%; colorless amorphous; IR (KBr, disk) νmax 3254, 2980, 1733, 1506, 1317 cm-1; 1H NMR (600 MHz, CDCl3) δ 1.39 (3H, t, J = 7.2 Hz, Et’s CH3), 4.40 (2H, q, J = 7.2 Hz, Et’s CH2), 5.11 (2H, s, Cbz’s CH2), 6.68 (1H, s, NH), 7.29 (1H, s, vinyl’s H), 7.29-7.53 (10H, m, Cbz’s PhH, ΔPhe’s PhH), 8.16 (1H, s, ring-H); 13C NMR (150 MHz, CDCl3) δ 14.3, 61.4, 67.6, 120.9, 128.0, 128.2, 128.2, 128.5, 128.7, 129.1, 129.4, 133.8, 134.5, 135.8, 143.9, 153.8, 160.9, 161.1. HRMS (ESI-TOF) m/z: [M+Na]+ calcd for C22H20N2NaO5 415.1270, found 415.1279.
Ethyl (Z)-2-[1-(((Benzyloxy)carbonyl)amino)-2-(4-methoxyphenyl)vinyl]oxazole-4-carboxylate (Cbz-ΔTyr(OMe)-Ozl-OEt) (1f): Yield 89%; colorless amorphous; IR (KBr, disk) νmax 3291, 2981, 1728, 1511, 1318 cm-1; 1H NMR (600 MHz, DMSO-d6 at 80 °C) δ 1.31 (3H, t, J = 7.1 Hz, Et’s CH3), 3.80 (3H, s, Tyr’s OMe), 4.32 (2H, q, J = 7.1 Hz, Et’s CH2), 5.10 (2H, br s, Cbz’s CH2), 6.94-7.66 (10H, m, Cbz’s PhH , ΔTyr’s PhH, Vinyl’s H), 8.73 (1H, s, ring-H), 9.17 (1H, s, NH); 13C NMR (150 MHz, DMSO-d6 at 80°C) δ 13.7, 55.0, 60.2, 65.6, 113.8, 119.6, 126.0, 127.1, 127.4, 127.9, 128.9, 131.0, 133.4, 136.4, 144.5, 154.1, 159.7, 160.3, 161.1. HRMS (ESI-TOF) m/z: [M+Na]+ calcd for C23H22N2NaO6 445.1376, found 445.1353.
Ethyl (Z)-2-[1-(((Benzyloxy)carbonyl)amino)-2-(4-nitrophenyl)vinyl]oxazole-4-carboxylate (Cbz-p-NO2ΔPhe-Ozl-OEt) (1g): Yield 87%; yellow solid; mp 162-164 °C (acetone-hexanes); IR (KBr, disk) νmax 3255, 3150, 1724, 1704, 1521, 1347, 1108, 738 cm-1; 1H NMR (600 MHz, CDCl3) δ 1.39 (3H, t, J = 7.2 Hz, Et’s CH3), 4.41 (2H, q, J = 7.2 Hz, Et’s CH2), 5.06 (2H, s, Cbz’s CH2), 7.20 (1H, br s, NH), 7.24 (1H, s, vinyl’s H), 7.33 (5H, br s, Cbz’s PhH), 7.59 (2H, d, J = 8.6 Hz, PhH), 8.09 (2H, d, J = 8.6 Hz, PhH), 8.22 (1H, s, ring-H); 13C NMR (150 MHz, CDCl3) δ 14.3, 61.6, 67.9, 123.2, 123.5, 123.7, 128.5, 128.5, 128.6, 129.6, 134.8, 135.5, 141.0, 144.5, 147.0, 152.9, 160.0, 160.7. HRMS (ESI-TOF) m/z: [M+Na]+ calcd for C22H19N3NaO7 460.1120, found 460.1150. Anal. Calcd for C22H19N3O7 (437.12): C, 60.41; H, 4.38; N, 9.61; O, 25.60. Found: C, 60.34; H, 4.34; N, 9.51.
Ethyl (Z)-2-[1-(((Benzyloxy)carbonyl)amino)-4-methoxy-4-oxobut-1-en-1-yl]oxazole-4-carboxylate (Cbz-ΔGlu(OMe)-Ozl-OEt) (1h): Yield 69%; colorless syrup; IR (KBr, neat) νmax 3307, 2954, 1733, 1508 cm-1; 1H NMR (600 MHz, CDCl3) δ 1.38 (3H, t, J = 7.1 Hz, Et’s CH 3), 3.37 (2H, d, J = 7.2 Hz, γ-H), 3.73 (3H, s, COOCH3), 4.39 (2H, q, J = 7.2 Hz, Et’s CH2), 5.16 (2H, s, Cbz’s CH2), 6.67 (1H, t, J = 7.2 Hz, vinyl’s H), 6.79 (1H, br s, NH), 7.32-7.42 (5H, m, Cbz’s PhH), 8.15 (1H, s, ring-H); 13C NMR (150 MHz, CDCl3) δ 14.3, 33.7, 52.2, 61.4, 67.8, 122.1, 123.9, 128.3, 128.4, 128.6, 134.2, 135.6, 144.1, 153.6, 159.7, 161.0, 170.1. HRMS (ESI-TOF) m/z: [M+Na]+ calcd for C19H20N2NaO7 411.1168, found 411.1128.
Ethyl (Z)-2-[[1,4-Bis(((benzyloxy)carbonyl)amino)]but-1-en-1-yl]oxazole-4-carboxylate (Cbz-ΔOrn(NCbz)-Ozl-OEt) (1i): Yield 91%; colorless syrup; IR (KBr, neat) νmax 3320, 2981, 1719, 1525, 1249 cm-1; 1H NMR (600 MHz, CDCl3) δ 1.37 (3H, t, J = 7.1 Hz, Et’s CH3), 2.51 (2H, dt, J = 6.4, 7.4 Hz, ΔOrn’s γ-CH2), 3.39 (2H, dt, J = 4.8, 6.4 Hz, ΔOrn’s δ-CH2), 4.38 (2H, q, J = 7.1 Hz, Et’s CH2), 5.09, 5.14 (4H, each s, Cbz’s CH2 × 2), 5.32 (1H, br s, NH), 6.46 (1H, t, J = 7.4 Hz, vinyl’s H), 6.66 (1H, br s, NH), 7.28-7.36 (10H, m, Cbz’s PhH × 2), 8.12 (1H, s, ring-H); 13C NMR (150 MHz, CDCl3) δ 14.3, 28.6, 39.8, 61.4, 66.6, 67.7, 123.5, 128.0, 128.0, 128.3, 128.4, 128.5, 128.6, 129.1, 134.2, 135.7, 136.6, 143.9, 154.2, 156.5, 160.0, 161.0. HRMS (ESI-TOF) m/z: [M+Na]+ calcd for C26H27N3NaO7 516.1747, found 516.1720.
General procedure for the preparation of dehydroamino acid amides (2a-i). To a solution of N-protected dehydroamino acid (ΔAA-OH) (4.0 mmol) in THF (10 mL) was added a solution of DCC (1.1 equiv) in THF at 0 °C. After stirring for 30 min, HOSu (N-hydroxysuccinimide) (1.1 equiv) was added to the resulting mixture. After stirring at room temperature for 5 min, the precipitates (dicyclohexylurea: DCU) was filtrated off through a pad of Celite. The filtrate was concentrated in vacuo to give a residue. The resulting residue was dissolved in EtOAc (10 mL), added 28% NH4OH (1 mL), and stirred continuously for 30 min at room temperature. The reaction mixture was washed with brine water, dried over anhydrous MgSO4, concentrated in vacuo to give a N-protected ΔAA-NH2 (2a-i)(70-92%), which was recrystallized from acetone-hexanes.
(Z)-2-((Benzyloxy)carbonyl)amino-2-butenamide (Cbz-ΔAbu-NH2) (2a): Yield 82%; white solid; mp 109-110 °C (acetone-hexanes); IR (KBr, disk) νmax 3468, 3144, 1692, 1657, 1503 cm-1; 1H NMR (600 MHz, DMSO-d6) δ 1.62 (3H, d, J = 7.0 Hz, ΔAbu’s CH3), 5.05 (2H, s, Cbz’s CH2), 6.23 (1H, q, J = 7.0 Hz, vinyl’s H), 7.01 (1H, s, NH2’s H), 7.31−7.38 (6H, m, Cbz’s PhH, NH2), 8.50 (1H, s, NH); 13C NMR (150 MHz, DMSO-d6) δ 12.9, 65.7, 126.0, 127.7, 127.8, 128.3, 131.4, 136.8, 154.1, 166.4. HRMS (ESI-TOF) m/z: [M+Na]+ calcd for C12H14N2NaO3 257.0902, found 257.0882. Anal. Calcd for C12H14N2O3 (234.10): C, 61.53; H, 6.02; N, 11.96. Found: C, 61.47; H, 6.02; N, 11.70.
(Z)-2-((tert-Butoxy)carbonyl)amino-2-butenamide (Boc-ΔAbu-NH2) (2b): Yield 86%; white solid; mp 116-117 °C (acetone-hexanes); IR (KBr, disk) νmax 3293, 2979, 1677, 1647 cm-1; 1H NMR (600 MHz, DMSO-d6) δ 1.39 (9H, s, Boc’s t-Bu), 1.59 (3H, d, J = 6.9 Hz, ΔAbu’s CH3), 6.11 (1H, br s, NH), 6.95, 7.20 (2H, each s, NH2), 8.00 (1H, br s, vinyl’s H); 13C NMR (150 MHz, DMSO-d6) δ 12.9, 28.1, 78.5, 125.0, 131.7, 153.3, 166.8. HRMS (ESI-TOF) m/z: [M+Na]+ calcd for C9H16N2NaO3 2223.1058, found 2223.1081. Anal. Calcd for C9H16N2O3 (200.24): C, 53.98; H, 8.05; N, 13.99. Found: C, 53.63; H, 8.42; N, 13.70.
2-((Benzyloxy)carbonyl)amino-3-methyl-2-butenamide (Cbz-ΔVal-NH2) (2c): Yield 87%; white solid; mp 146-148 °C (acetone-hexanes); IR (KBr, disk) νmax 3451, 2903, 1667, 1641, 1599 cm-1; 1H NMR (600 MHz, DMSO-d6) δ 1.66, 1.92 (3H × 2, each s, ΔVal’s CH3 × 2), 5.04 (2H, s, Cbz’s CH2), 7.01, 7.11 (1H × 2, each s, NH2), 7.31-7.38 (5H, m, Cbz’s PhH); 8.48 (1H, s, NH); 13C NMR (150 MHz, DMSO-d6) δ 20.4, 20.7, 65.5, 125.6, 127.7, 127.8, 128.3, 133.6, 137.0, 154.3, 167.3. HRMS (ESI-TOF) m/z: [M+Na]+ calcd for C13H16N2NaO3 271.1059, found 271.1030.
(Z)-2-((Benzyloxy)carbonyl)amino-4-methyl-2-pentenamide (Cbz-ΔLue-NH2) (2d): Yield 92%; white solid; mp 117-118 °C (acetone-hexanes); IR (KBr, disk) νmax 3278, 2961, 1684, 1507 cm-1; 1H NMR (600 MHz, DMSO-d6) δ 0.94 (6H, d, J = 6.4 Hz, ΔLeu’s CH3), 2.56 (1H, m, ΔLeu’s H), 5.04 (2H, s, Cbz’s CH2), 6.02 (1H, d, J = 6.9 Hz, vinyl’s H), 7.01 (1H, s, NH2), 7.30-7.38 (6H, m, Cbz’s PhH, NH2), 8.49 (1H, s, NH); 13C NMR (150 MHz, DMSO-d6) δ 21.8, 26.2, 65.6, 127.6, 127.8, 128.2, 128.3, 136.9, 138.5, 154.6, 166.6. HRMS (ESI-TOF) m/z: [M+Na]+ calcd for C14H18N2NaO3 285.1215, found 285.1256.
(Z)-2-((Benzyloxy)carbonyl)amino-3-phenyl-2-propenamide (Cbz-ΔPhe-NH2) (2e): Yield 87%; white solid; mp 166-167 °C (acetone-hexanes); IR (KBr, disk) νmax 3337, 1713, 1607 cm-1; 1H NMR (500MHz, DMSO-d6) δ 5.06 (2H, s, Cbz’s CH2), 7.06 (1H, s, vinyl’s H), 7.23 (2H, s, NH2), 7.30-7.56 (10H, m, Cbz’s PhH, ΔPhe’s PhH), 7.59 (1H, s, NH); 13C NMR (150 MHz, DMSO-d6) δ 65.7, 127.5, 127.7, 127.9, 128.3, 128.4, 128.5, 129.4, 129.5, 134.2, 136.9, 154.4, 167.0. HRMS (ESI-TOF) m/z : [M+Na]+ calcd for C17H16N2NaO3 319.1059, found 319.1044.
(Z)-2-((Benzyloxy)carbonyl)amino-3-(4-methoxyphenyl)-2-propenamide (Cbz-p-ΔTyr(OMe)-NH2) (2f): Yield 77%; white solid; mp 147-149 °C (acetone-hexanes); IR (KBr, disk) νmax 3452, 3336, 3208, 3105, 2898, 1720, 1662, 1578 cm-1; 1H NMR (600 MHz, CDCl3) δ 3.86 (3H, s, Tyr’s OMe), 5.20 (2H, s, Cbz’s CH2), 5.66 (2H, br s, NH2), 6.89 (2H, d, J = 8.7 Hz, Tyr’s PhH), 7.27 (1H, s, vinyl’s H), 7.27-7.37 (5H, m, Cbz’s PhH), 7.44 (2H, d, J = 8.7 Hz, Tyr’s PhH); 13C NMR (150 MHz, CDCl3) δ 24.9, 25.6, 34.0, 55.3, 67.8, 114.3, 125.8, 128.3, 128.4, 128.6, 131.3, 131.3, 135.8, 160.4, 167.6; 13C NMR (150 MHz, DMSO-d6) δ 14.4, 55.2, 65.7, 113.9, 126.6, 127.2, 127.6, 127.7, 128.3, 128.5, 131.2, 137.0, 154.4, 159.5, 167.2. HRMS (ESI-TOF) m/z: [M+Na]+ calcd for C18H18N2NaO4 349.1164, found: 349.1134.
(Z)-2-((Benzyloxy)carbonyl)amino-3-(4-nitrophenyl)-2-propenamide (Cbz-p-NO2ΔPhe-NH2) (2g): Yield 82%; yellow solid; mp 182-184 °C (acetone-hexanes); IR (KBr, disk) νmax 3294, 3466, 3245, 3145, 1688, 1522, 1342, 1255 cm-1; 1H NMR (600 MHz, DMSO-d6) δ 5.06 (2H, s, Cbz’s CH2), 7.01 (1H, s, vinyl’s H) 7.32-7.40 (6H, m, Cbz’s PhH, NH2’s H), 7.75 (2H, d, J = 8.8 Hz, Tyr’s PhH), 7.78 (1H, s, NH2’s H), 8.18 (2H, d, J = 8.8 Hz, Tyr’s PhH); 9.18 (1H, s, NH ); 13C NMR (150 MHz, DMSO-d6) δ 66.0, 123.5, 123.7, 127.7, 127.8, 128.3, 130.1, 133.0, 136.6, 141.4, 146.4, 154.0, 166.8; HRMS (ESI-TOF) m/z: [M+Na]+ calcd for C17H15N3NaO5 364.0909, found 364.0946.
Methyl (Z)-5-Amino-4-[((benzyloxy)carbonyl)amino]-5-oxo-3-pentenoate (Cbz-ΔGlu(OMe)-NH2) (2h): Yield 70%; white solid; mp 110-111 °C; IR (KBr, disk) νmax 3435, 3388, 3191, 2997, 1725, 1701, 1670, 1655, 1609, 1278 cm-1; 1H NMR (600 MHz, DMSO-d6) δ 3.18 (2H, d, J = 7.0 Hz, γ-H), 3.61 (3H, s, COOCH3), 5.05 (2H, s, Cbz’s CH2), 6.21 (1H, t, J = 7.0 Hz, vinyl’s H), 7.14 (1H, s, NH2), 7.32-7.38 (5H, m, Cbz’s PhH), 7.48 (1H, s, NH2), 8.70 (1H, s, CbzNH); 13C NMR (150 MHz, DMSO-d6) δ 32.2, 51.7, 65.9, 121.4, 127.7, 127.8, 128.3, 132.3, 136.7, 153.9, 165.9, 170.7; HRMS (ESI-TOF) m/z: [M+Na]+ calcd for C14H16N2NaO5 315.0956, found 315.1022. Anal. Calcd for C14H16N2O5 (292.29): C, 57.53; H, 5.52; N, 9.58. Found: C, 57.52; H, 5.52; N, 9.75.
(Z)-2,5-Di[((benzyloxy)carbonyl)amino]-2-pentenamide (Cbz-ΔOrn(NCbz)-NH2) (2i): Yield: 71%; white solid; mp 128-130 °C (acetone-hexanes); IR (KBr, disk) νmax 3296, 3036, 1686, 1509, 1249 cm-1; 1H NMR (600 MHz, DMSO-d6) δ 2.21 (2H, dd, J = 6.9, 13.5 Hz, γ-H), 3.08 (2H, dd, J = 6.6, 13.5 Hz, δ-H), 5.01, 5.04 (2H × 2, each s, Cbz’s CH2), 6.09 (1H, t, J = 6.9 Hz, vinyl’s H), 7.06 (1H, s, NH2), 7.29-7.37 (12H, m, Cbz’s PhH × 2, NH2’s H, NH), 8.57 (1H, s, NH); 13C NMR (150 MHz, DMSO-d6) δ 27.6, 39.3, 65.2, 65.7, 127.3, 127.6, 127.7, 127.7, 127.7, 127.8, 128.3, 128.3, 131.5, 136.8, 137.2, 154.2, 156.1, 166.4. HRMS (ESI-TOF) m/z: [M+Na]+ calcd for C21H23N3NaO5 420.1535, found 420.1537.
(E)-2-Methyl-2-butenamide (2j) (Tiglic acid amide): The preparation procedure was the same as 2a-i. Yield 81 %; white solid; mp 126.5-127.5 °C (acetone-hexanes); IR (KBr, disk) νmax 3376, 3181, 2921, 1666, 1612 cm-1; 1H NMR (600 MHz, CDCl3) δ 1.87 (3H, dq, J = 1.5, 7.1 Hz, γ-CH3), 1.91 (3H, dq, J = 1.5, 1.5 Hz, CH3), 5.63 (1H, s, NH2’s H), 5.71 (1H, br qq, J = 1.5, 7.1 Hz, vinyl’s H), 6.30 (1H, s, NH2’s H).
(Z)-2-Methyl-2-butenamide (2k) (Angelic acid amide): The preparation procedure was the same as 2a-i. Yield 88 %; white solid; mp 164.5-166.5 °C (acetone-hexanes); IR (KBr, disk) νmax 3344, 3178, 2935, 1676, 1597 cm-1; 1H NMR (600 MHz, CDCl3) δ 1.77 (3H, dq, J = 1.2, 6.9 Hz, γ-CH3), 1.85 (3H, dq, J = 1.2, 1.2 Hz, CH3), 5.88, 6.22 (1H × 2, each s, NH2’s H × 2), 6.52 (1H, br qq, J = 1.2, 6.9 Hz, vinyl’s H).
Methyl 3-Bromo-2-oxobutanoate (3b). 1H NMR (600 MHz, CDCl3) δ 1.82 (3H, d, J = 6.8 Hz, CHBrCH3), 3.94 (3H, s, OCH3), 5.17 (1H, q, J = 6.8 Hz, CHBr). This compound was used in the next step without further purification after usual workup, because this compound was unstable.
Methyl 3-Bromo-2-oxo-4-methylpentanoate (3c): 1H NMR (600 MHz, CDCl3) δ 1.06, 1.14 (3H × 2, each d, J = 6.6 Hz, (CH3)2CH), 2.36 (1H, dq, J = 7.9, 6.6 Hz, (CH3)2CH), 3.93 (3H, s, OCH3), 4.85 (1H, d, CHBr, J = 7.9 Hz). This compound was used in the next step without further purification after usual workup, because this compound was unstable.
Methyl 3-Bromo-2-oxo-3-phenylpropanoate (3d): 1H NMR (600 MHz, CDCl3) δ 3.94 (3H, s, OCH3), 6.21 (1H, s, CHBr), 7.38-7.47 (5H, m, PhH). This compound was used in the next step without further purification after usual workup, because this compound was unstable.
General procedure for the reaction of dehydroamino acid amides (2a) and β-substituted β-bromopyruvate (3b-d).
Conventional heating: To a stirred solution of 2a (0.8 mmol) in THF (5 mL) were added NaHCO3 (336 mg, 5 equiv) and a solution of methyl β-substituted β-bromopyruvate (3b-d) (2 equiv) in THF (1 mL) at 0 °C. After stirring for 5-72 h at reflux, the resulting solution was diluted with CHCl3 (10 mL) and the organic layer was washed with brine and water, dried over anhydrous MgSO4, and concentrated in vacuo to give a yellow syrup. Resulting syrup was dissolved in THF (5 mL), TFAA (trifluoracetic anhydride) (333 µL, 3 equiv) and pyridine (388 µL, 6 equiv) were added to the mixture at 0 °C, and stirred for 30 min. The reaction mixture was concentrated in vacuo to give brown syrup. Resulting syrup was further dissolved in EtOAc (5 mL), 28% NH4OH (1 mL) was added to the above mixture at 0 °C, and stirred for 15 min. reaction mixture was poured into brine, washed with brine and water, dried over anhydrous MgSO4, concentrated in vacuo. Resulting brown syrup was purified on a silica gel column to give 5-substituted oxazole derivatives (4b-d) (62-78%).
Microwave heating: To a stirred solution of 2a (0.8 mmol) in THF (5 mL) were added NaHCO3 (336 mg, 5 equiv) and a solution of methyl β-substituted β-bromopyruvate (3b-d) (2 equiv) in THF (1 mL) at 0 °C. The flask was flushed with argon, heated with stirring at reflux under microwave irradiation for 5-72 min. After cooling to rt, the reaction mixture was diluted with CHCl3 (10 mL) and the organic layer was washed with brine and water, dried over anhydrous MgSO4, and concentrated in vacuo to give a yellow syrup. Resulting syrup was dissolved in THF (5 mL), TFAA (trifluoracetic anhydride) (333 µL, 3 equiv) and pyridine (388 µL, 6 equiv) were added to the mixture at 0 °C, and stirred for 30 min. The reaction mixture was concentrated in vacuo to give brown syrup. Resulting syrup was further dissolved in EtOAc (5 mL), 28% NH4OH (1 mL) was added to the above mixture at 0 °C, and stirred for 15 min. reaction mixture was poured into brine, washed with brine and water, dried over anhydrous MgSO4, concentrated in vacuo. Resulting brown syrup was purified on a silica gel column to give 5-substituted oxazole derivatives (4b-d)(71-80%).
Methyl (Z)-2-[1-(((Benzyloxy)carbonyl)amino)prop-1-en-1-yl]-5-methyloxazole-4-carboxylate (Cbz-ΔAbu-MeOzl-OMe) (4b): Yield 74%; colorless crystals; mp 136-138 °C (acetone-hexanes); IR (KBr, disk) νmax 3307, 2953, 1717, 1507 cm-1; 1H NMR (600 MHz, CDCl3) δ 1.85 (3H, d, J = 7.2 Hz, ΔAbu’s CH3), 2.60 (3H, s, ring-CH3), 3.90 (3H, s, OCH3), 5.15 (2H, s, Cbz’s CH2), 6.45 (1H, br s, NH), 6.54 (1H, q, J = 7.2 Hz, vinyl’s H), 7.32-7.37 (5H, m, Cbz’s PhH); 13C NMR (150 MHz, CDCl3) δ 12.1, 14.0, 52.0, 67.4, 122.8, 127.4, 128.0, 128.2, 128.2, 128.5, 136.0, 153.9, 156.5, 157.8, 162.6. HRMS (ESI-TOF) m/z: [M+Na]+ calcd for C17H18N2NaO5 353.1113, found 353.1118.
Methyl (Z)-2-[1-(((Benzyloxy)carbonyl)amino)prop-1-en-1-yl]-5-isopropyloxazole-4-carboxylate (Cbz-ΔAbu-i-PrOzl-OMe) (4c): Yield 62%; yellow amorphous; IR (KBr, disk) νmax 3567, 2979, 1717, 1507 cm-1; 1H NMR (600 MHz, CDCl3) δ 1.28 (6H, d, J = 7.0 Hz, i-Pr’s CH3 × 2), 1.87 (3H, d, J = 7.2 Hz, ΔAbu’s CH3), 3.76 (1H, dq, J = 7.0, 7.0 Hz, i-Pr’s CH), 3.90 (3H, s, COOCH3), 5.16 (2H, s, Cbz’s CH2), 6.51 (1H, br s, NH), 6.55 (1H, q, J = 7.2 Hz, vinyl’s H), 7.32-7.39 (5H, m, Cbz’s PhH); 13C NMR (150 MHz, CDCl3) δ 14.0, 20.6, 26.1, 52.0, 67.4, 122.9, 126.1, 127.3, 128.2, 128.2, 128.5, 136.0, 153.9, 157.5, 162.6, 164.6. HRMS (ESI-TOF) m/z: [M+Na]+ calcd for C19H22N2NaO5 381.1426, found: 381.1401.
Methyl (Z)-2-[1-(((Benzyloxy)carbonyl)amino)prop-1-en-1-yl]-5-phenyloxazole-4-carboxylate (Cbz-ΔAbu-PhOzl-OMe) (4d): Yield 80%; coloeless needles; mp 148.5-151 °C (acetone-hexanes); IR (KBr, disk) νmax 3301, 2955, 1724, 1493 cm-1; 1H NMR (600 MHz, CDCl3) δ 1.90 (3H, d, J = 7.2 Hz, ΔAbu’s CH3), 3.93 (3H, s, OCH3), 5.18 (2H, s, Cbz’s CH2), 6.49 (1H, br s, NH), 6.68 (1H, d, J = 7.3 Hz, vinyl’s H), 7.26-7.48 and 8.00-8.02 (10H, m, Cbz’s PhH); 13C NMR (150 MHz, CDCl3) δ 14.1, 52.2, 52.3, 67.5, 122.8, 126.7, 127.4, 128.2, 128.3, 128.4, 128.4, 128.5, 128.5, 130.3, 130.5, 135.9, 153.9, 155.4, 157.8, 162.4. HRMS (ESI-TOF) m/z: [M+Na]+ calcd for C22H20N2NaO5 415.1270, found 415.1251.
Reaction of 2-methylbutenamide (2j, k) and ethyl β-bromopyruvate (3a). To a solution of 2j (2k), (80 mg, 0.8 mmol) in THF (5 mL) were added NaHCO3 (336 mg, 5 equiv) and ethyl β-bromopyruvate (3a) (312 mg, 2 equiv) at 0 °C, and stirred for 2 h at reflux conditions. After cooling to room temperature, the reaction mixture was diluted with CHCl3 (10 mL), washed with water (10 mL) and brine (10 mL), dried over anhydrous MgSO4 and concentrated in vacuo to give a yellow syrup. Remaining residue was dissolved in THF (5mL), TFAA (178 µL, 3 equiv) and pyridine (390 µL, 6 equiv) were added to the mixture at 0 °C, and stirred for 30 min. the reaction mixture was concentrated in vacuo to give a brown syrup. The resulting syrup was further dissolved in EtOAc (5 mL), 28% NH4OH (1 mL) was added to the mixture at 0 °C. After stirring for 15 min, the reaction mixture was washed with brine and dried over anhydrous MgSO4, concentrated in vacuo to give 4e and 4f, which were purified on a silica gel column with hexanes-EtOAc (1:1 v/v).
Ethyl (E)-2-(1-Methyl-1-propen-1-yl)-4-oxazolecarboxylate (4e) (main product): Yield 88%; colorless syrup; IR (KBr, neat) νmax 3469, 3157, 2983, 2930, 2858, 1742, 1719, 1576, 1541, 1317 cm-1; 1H NMR (600 MHz, CDCl3) δ 1.39 (3H, t, J = 7.1 Hz, Et’s CH3), 1.87 (3H, dq, J = 7.2, 1.1 Hz, γ-CH3), 2.10 (3H, dq, J = 1.1, 1.1 Hz, CH3), 4.39 (2H, q, J = 7.1 Hz, Et’s CH2), 6.73 (1H, qq, J = 1.1, 7.2 Hz, vinyl’s H), 8.12 (1H, s, ring-H); 13C NMR (150 MHz, CDCl3) δ 12.7, 14.1, 14.3, 61.1, 123.9, 131.1, 134.1, 143.0, 161.6, 164.4. HRMS (ESI-TOF) m/z: [M+Na]+ calcd for C10H13NNaO3 218.0793, found 218.0748.
NOE correlation: γ-CH3 ←→ CH3, γ-CH3 ←no observation→ vinyl’s H
Ethyl (Z)-2-(1-Methyl-1-propen-1-yl)-4-oxazolecarboxylate (4f) (by-product): Yield 5%; colorless syrup; IR (KBr, neat) νmax 3448, 3157, 2981, 2923, 2852, 1742, 1720, 1575, 1151, 1113 cm-1; 1H NMR (600 MHz, CDCl3) δ 1.39 (3H, t, J = 7.1 Hz, Et’s CH3), 2.11 (3H, dq, J = 7.3, 1.5 Hz, γ-CH3), 2.15 (3H, dq, J = 1.5, 1.5 Hz, CH3), 4.40 (2H, q, J = 7.1 Hz, Et’s CH2), 6.04 (1H, qq, J = 1.5, 7.3 Hz, vinyl’s H), 8.19 (1H, s, ring-H); 13C NMR (150 MHz, CDCl3) δ 14.3, 15.7, 21.2, 61.1, 122.6, 133.2, 133.8, 142.7, 161.6, 163.5. HRMS (ESI-TOF) m/z: [M+Na]+ calcd for C10H13NNaO3 218.0793, found 218.0748.
NOE correlation: γ-CH3 ←no observation→ CH3, γ-CH3 ←→ vinyl’s H
ACKNOWLEDGEMENTS
This work was supported by the Science Frontier Project of Kanagawa University. We thank Dr. S. Hanashima (RIKEN Advanced Science Institute) for helpful discussions.
References
1. (a) D. C. Palmer and S. Venkatraman, Chemistry of Heterocyclic Compounds (Oxazols: Synthesis, Reactions, and Spectroscopy), Part A (ed. by D. C. Palmer), Wiley, Hoboken, NJ, 2003, vol. 60, pp.1-390; CrossRef (b) D. C. Palmer and S. Venkatraman, Chemistry of Heterocyclic Compounds (Oxazols: Synthesis, Reactions, and Spectroscopy), Part B (ed. by D. C. Palmer), Wiley, Hoboken, NJ, 2004, vol. 60, pp.1-720; CrossRef (c) Z. Jin, Nat. Prod. Rep., 2003, 20, 584; CrossRef (d) Z. Jin, Nat. Prod. Rep., 2009, 26, 382; CrossRef (e) P. Wipf, Chem. Rev., 1995, 95, 2115; CrossRef (f) R. A. Hughes and C. J. Moody, Angew. Chem. Int. Ed., 2007, 46, 7930; CrossRef (g) G. Fontana, Curr. Bioact. Compd., 2010, 6, 284; CrossRef (h) C. Shin and Y. Yonezawa, Kagaku to Kogyo (in Japanese), 2004, 57, 933 (CA 141:314023); (i) V. S. C. Yeh, Tetrahedron, 2004, 60, 11995. CrossRef
2. (a) R. Robinson, J. Chem. Soc., Trans., 1909, 95, 2167; CrossRef (b) S. Gabriel, Ber. Dtsch. Chem. Ges., 1910, 43, 134; CrossRef (c) S. Gabriel, Ber. Dtsch. Chem. Ges., 1910, 43, 1283; CrossRef (d) D. K. Knight, G. Pattenden, and D. E. Rippon, Synlett, 1990, 36; CrossRef (e) S. K. Yoo, Tetrahedron Lett., 1992, 33, 2159; CrossRef (f) R. Shapiro, J. Org. Chem., 1993, 58, 5759; CrossRef (g) D. J. Brown and P. B. Ghosh, J. Chem. Soc. B., 1969, 270, 270; CrossRef (h) G. Theilig, Chem. Ber., 1953, 86, 96; CrossRef (i) A. Dornow and H. Hell, Chem. Ber., 1960, 93, 1998; CrossRef (j) W. J. Hammar and M. A. Rustad, J. Heterocycl. Chem., 1981, 18, 885; CrossRef (k) H. Bredereck and R. Gompper, Chem. Ber., 1954, 87, 700; CrossRef (l) J. Hutton, B. Potts, and F. F. Southern, Synth. Commun., 1979, 9, 789; CrossRef (m) H. Bredereck, R. Gompper, and F. Reich, Chem. Ber., 1960, 93, 723; CrossRef (n) R. D. Viirre, G. Evindar, and R. A. Batey, Can. J. Org. Chem., 2008, 73, 3452; CrossRef (o) K. Schuh and F. Glorius, Synthesis, 2007, 2297; CrossRef (p) A. S. K. Hashmi, M. Rudolph, S. Schymura, J. Visus, and W. Frey, Eur. J. Org. Chem., 2006, 4905; CrossRef (q) S. Ueda and H. Nagasawa, Angew. Chem. Int. Ed., 2008, 47, 6411; CrossRef (r) H. Mihara, Y. Xu, E. Shepherd, S. Matsunaga, and M. Shibasaki, J. Am. Chem. Soc., 2009, 131, 8384; CrossRef (s) A. Hassner and B. Fischer, Heterocycles, 1993, 35, 1441; CrossRef (t) E. Riego, D. Hernandez, F. Albericio, and M. Alvarez, Synthesis, 2005, 1907; CrossRef (u) T. G. Graham and A. I. Meyers, Tetrahedron, 1994, 50, 2297; CrossRef (v) B. Shi, A. J. Blake, W. Lewis, I. B. Campbell, B. D. Judkins, and C. J. Moody, J. Org. Chem., 2010, 75, 152; CrossRef (w) P. Wipf and C. P. Miller, J. Org. Chem., 1993, 58, 3604; CrossRef (x) H. Jiang, H. Huang, H. Cao, and C. Qi, Org. Lett., 2010, 12, 5561; CrossRef (y) A. J. Philips, Y. Uto, P. Wipf, M. J. Reno, and D. R. Williams, Org. Lett., 2000, 2, 1165; CrossRef (z) J. Das, J. A. Reid, D. R. Kronenthal, J. Singh, P. D. Pansegrau, and R. H. Mueller, Tetrahedron Lett., 1992, 33, 7835. CrossRef
3. (a) F. O. Blümlein, Ber. Dtsch. Chem. Ges., 1884, 17, 2578; CrossRef (b) M. Lewy, Ber. Dtsch. Chem. Ges., 1887, 20, 2576; CrossRef (c) D. J. Ritson, C. Spiteri, and J. E. Moses, J. Org. Chem., 2011, 76, 3519. CrossRef
4. (a) C. Shin, Y. Nakamura, and K. Okumura, Chem. Lett., 1993, 22, 1405; CrossRef (b) K. Okumura, A. Ito, H. Saito, Y. Nakamura, and C. Shin, Bull. Chem. Soc. Jpn., 1996, 69, 2309; CrossRef (c) T. Yamada, K. Okumura, Y. Yonezawa, and C. Shin, Chem. Lett., 2001, 30, 102; CrossRef (d) H. Saito, T. Yamada, K. Okumura, Y. Yonezawa, and C. Shin, Chem. Lett., 2002, 31, 1098; CrossRef (e) N. Endoh, K. Tsuboi, R. Kim, Y. Yonezawa, and C. Shin, Heterocycles, 2003, 60, 1567; CrossRef (f) T. Kayano, Y. Yonezawa, and C. Shin, Chem. Lett., 2004, 33, 72; CrossRef (g) C. Shin, C. Abe, and Y. Yonezawa, Chem. Lett., 2004, 33, 664. CrossRef
5. (a) M. W. Bredenkamp, C. W. Holzapfel, and W. J. Van Zyl, Synth. Commun., 1990, 20, 2235; CrossRef (b) E. Aguilar and A. I. Meyers, Tetrahedron Lett., 1994, 35, 2473; CrossRef (c) C. J. Moody, J. Am. Chem. Soc., 2005, 127, 15644; CrossRef (d) K. Okumura, H. Saito, C. Shin, K. Umemura, and J. Yoshimura, Bull. Chem. Soc. Jpn., 1998, 71, 1863; CrossRef (e) Y. Yonezawa, H. Saito, S. Suzuki, and C. Shin, Heterocycles, 2002, 57, 903; CrossRef (f) C. Shin, A. Okabe, A. Ito, and Y. Yonezawa, Bull. Chem. Soc. Jpn., 2002, 75, 1583; CrossRef (g) E. A. Merritt and M. C. Bagley, Synthesis, 2007, 22, 3535. CrossRef
6. (a) J. S. Panek and R. T. Beresis, J. Org. Chem., 1996, 61, 6496; CrossRef (b) P. Liu, C. A. Celatka, and J. S. Panek, Tetrahedron Lett., 1997, 38, 5445. CrossRef
7. (a) C. J. Moody and M. C. Bagley, J. Chem. Soc., Perkin Trans. 1, 1998, 601; CrossRef (b) M. C. Bagley, K. E. Bashford, C. L. Hesketh, and C. J. Moody, J. Am. Chem. Soc., 2000, 122, 3301; CrossRef (c) M. C. Bagley, K. Chapaneri, J. W. Dale, X. Xiong, and J. Bower, J. Org. Chem., 2005, 70, 1389; CrossRef (d) M. C. Bagley and C. Glover, Tetrahedron, 2006, 62, 66. CrossRef
8. T. J. Hoffman, J. H. Rigby, S. Arseniyadis, and J. Cossy, J. Org. Chem., 2008, 73, 2400. CrossRef
9. (a) Y. Nakamura, C. Shin, K. Umemura, and J. Yoshimura, Chem. Lett., 1992, 21, 1005; CrossRef (b) Y. Nakamura and C. Shin, Synthesis, 1994, 552; CrossRef (c) C. Shin, Y. Nakamura, Y. Yamada, Y. Yonezawa, K. Umemura, and J. Yoshimura, Bull. Chem. Soc. Jpn., 1995, 68, 3151; CrossRef (d) C. Shin, M. Hayakawa, T. Suzuki, A. Ohtsuka, and J. Yoshimura, Bull. Chem. Soc. Jpn., 1978, 51, 550; CrossRef (e) C. Shin Y. Yonezawa, T. Yamada, and J. Yoshimura, Bull. Chem. Soc. Jpn., 1982, 55, 2147. CrossRef
10. Ethyl β-bromopyruvate was commercially available from Aldrich (CAS 70-23-5).
11. S.-T. Chen, S.-T. Wu, and K.-T Wang, Synthesis, 1989, 37. CrossRef
12. (a) A. Nagasaki, Y. Adachi, Y. Yonezawa, and C. Shin, Heterocycles, 2003, 60, 321; CrossRef (b) Y. Yonezawa, N. Tani, and C. Shin, Bull. Chem. Soc. Jpn., 2005, 78, 1492; CrossRef (c) Y. Yonezawa and C. Shin, Kanagawa Daigaku Kogaku Kenkyusho Shoho (in Japanese), 1993, 16, 40 (CA 121:83940).
13. For a recent example, see: (a) H. M. Kingston and S. J. Haswell, Eds., “Microwave-Enhanced Chemistry. Fundamentals, Sample Preparation and Applications”, American Chemical Society: Washington, DC, 1997; (b) A. Loupy, Ed., “Microwaves in Organic Synthesis”, Wiley-VCH: Weinheim, 2002; (c) B. L. Hayes, “Microwave Synthesis: Chemistry at the Speed of Light”, CEM Publishing: Matthews, NC, 2002; (d) P. Lidström and J. P. Tierney, Eds., ”Microwave-Assisted Organic Synthesis“, Blackwell Publishing: Oxford, 2005; (e) C. O. Kappe and A. Stadler, “Microwaves in Organic and Medicinal Chemistry”, Wiley-VCH: Weinheim, 2005; CrossRef (f) A. Loupy, Ed., “Microwaves in Organic Synthesis, 2nd ed.”, Wiley-VCH: Weinheim, 2006; (g) M. Larhed, K. Olofsson, Eds., “Microwave Methods in Organic Synthesis”, Springer: Berlin, 200614..
14. Microwave irradiation experiments were carried out in standard microwave process with Pyrex flask. Reaction time reflects irradiation times at the set reaction temperature (fixed hold times). Reaction temperature was measured using a Teflon-coated thermocouple of type K.
15. (a) A. Babadjamian, J. Metzger, and M. Chanon, J. Heterocycl. Chem., 1975, 12, 643; CrossRef (b) A. Babadjamian, R. Gallo, J. Metzger, and M. Chanon, J. Heterocycl. Chem., 1976, 13, 1205. CrossRef
16. (a) J. Wojcik, M. Witanowski, L. Stefaniak, and G. A. Webb, Bull. Pol. Acad. Sci., Chem., 1985, 33, 443; (b) O. H. Wheeler, J. Am. Chem. Soc., 1956, 78, 3216; CrossRef (c) W. Kirmse, J. Rode, and K. Rode, Chem. Ber., 1986, 119, 3672; CrossRef (d) C. C. J. Culvenor and T. A. Geissman, J. Org. Chem., 1961, 26, 3045; CrossRef (e) F. Shi, M. R. Smith, III, and R. E. Maleczka, Jr., Org. Lett., 2006, 8, 1411; CrossRef (f) M. Yamada, Yakugaku Zasshi, 1962, 82, 562 (CA 1962:200670).
17. (a) U. Schmidt, H. Griesser, V. Leitenberger, A. Lieberknecht, R. Mangold, R Meyer, and B. Riedl, Synthesis, 1992, 487; CrossRef (b) U. Schmidt, A. Lieberknecht, and J. Wild, Synthesis, 1984, 53; CrossRef (c) P. G. Ciattini, C. E. Morera, and G. Orlar, Synthesis, 1988, 140; CrossRef (d) C. Shin, Y. Yonezawa, and E. Watanabe, Tetrahedron Lett., 1985, 26, 85; CrossRef (e) Y. Yonezawa, C. Shin, Y. Ono, and J. Yoshimura, Bull. Chem. Soc. Jpn., 1980, 53, 2905; CrossRef (f) J. E. Baldwin, J. K. Cha, and L. I. Kruse, Tetrahedron, 1985, 41, 5241; CrossRef (g) C. Shin, Y. Yonezawa, T. Obara, and H. Nishio, Bull. Chem. Soc. Jpn., 1988, 61, 885; CrossRef (h) C. Shin, T. Obara, S. Taniguchi, and Y. Yonezawa, Bull. Chem. Soc. Jpn.,1989, 62, 1127; CrossRef (i) C. Shin, N. Takahashi, and Y. Yonezawa, Chem. Pharm. Bull., 1990, 38, 2020. CrossRef
18. C. Verrier, C. Hoarau, and F. Marsais, Org. Biomol. Chem., 2009, 7, 647. CrossRef
19. For example, (a) J. Linder, J. A. Blake, C. Moody, and J. Christopher, Org. Biomol. Chem., 2008, 6, 3908; CrossRef (b) J. R. Davies, P. D. Kane, and C. J. Moody, J. Org. Chem. 2005, 70, 7305; CrossRef (c) S. Kehraus, G. M. Koenig, A. D. Wright, and G. Woerheide, J. Org. Chem., 2002, 67, 4989; CrossRef (d) H. Abe, K. Kushida, Y. Shiobara, and M. Kodama, Tetrahedron Lett., 1988, 9, 1401. CrossRef
20. The geometric configurations of all oxazole-dehydroamino acid derivatives 1a-i and 4b-d were deduced from the reaction mechanistic studies using 4e and 4f in Scheme 4.