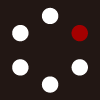
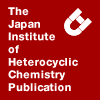
HETEROCYCLES
An International Journal for Reviews and Communications in Heterocyclic ChemistryWeb Edition ISSN: 1881-0942
Published online by The Japan Institute of Heterocyclic Chemistry
e-Journal
Full Text HTML
Received, 31st October, 2011, Accepted, 30th November, 2011, Published online, 2nd December, 2011.
DOI: 10.3987/COM-11-12382
■ First Synthesis of [6-15N]-Cladribine Using Ribonucleoside as a Starting Material
Norikazu Sakakibara, Ai Kakoh, and Tokumi Maruyama*
Faculty of Pharmaceutical Sciences at Kagawa Campus, Tokushima Bunri University, 1314-1 Shido, Sanuki City, Kagawa, 769-2193, Japan
Abstract
We have synthesized two types of [6-15N]-deoxyadenosine analogs: [6-15N]-2′-deoxyadenosine (1) and [6-15N]-2-chloro-2′-deoxyadenosine ([6-15N]-cladribine, 2), which utilized the readily available ribonucleosides inosine and guanosine, respectively, via 3′-benzoyl-5′-trityl- (or tert-butyldimethylsilyl)-substituted intermediates (6, 15).15N-labelled adenosine derivatives can be applicable to a variety of intended purposes. Practically speaking, many of the [15N]-nitrogen-stable isotope-labeled nucleosides are synthetic building blocks of 15N-labelled oligonucleotides, which are more commonly utilized as probes that help clarify the structure of nucleic acids, the mechanism of drug binding, and the interactions between proteins and nucleosides by the use of 1H-NMR spectra, 15N-NMR spectra, or both.1-3 In particular, [6-15N]-2′-deoxyadenosine (1) was synthesized relatively early once this nucleoside analog started to attract attention as a probe material. Jones et al. reported a synthetic process of this labeled compound in which deoxyadenosine was enzymatically deaminated to give deoxyinosine, which was then di-acetylated at the 3′- and 5′-hydroxy groups, and then O6-sulfonylation, followed by [15N]-benzylamination at the 6-position of the adenine skeleton, following oxidation with ruthenium oxide.1 In addition, Kelly and co-workers have synthesized [6-15N]-deoxyadenosine (1) in two steps from commercially available starting material, 6-chloropurine using the enzymatic transglycosylation method.2 Their approach has allowed for the simple and straightforward construction of large quantities of this material. While Maruyama et al. in our laboratory synthesized an effectively potent anti-HIV agent, FddA, using a 6-chloropurine riboside analog as a starting material via the conversion of selective 3′-benzoylation, 2′-fluorination, and 3′-deoxygenation in the sugar moiety.4,5 In this study, we adapted Maruyama’s approach to develop an alternative synthetic route to [6-15N]-2′-deoxyadenosine (1) from the readily available and economical ribonucleoside inosine, using it as the starting substance.
On the other hand, 2-chloro-2′-deoxyadenosine, also known as cladribine, is utilized as a therapeutic agent of hairy cell leukemia and disseminated sclerosis. However, it remains a mystery as to how to exert cladribine’s action mechanism against these diseases.6,7 To solve these problems, tracer experiments with cladribine must be carried out in order to understand the effect administration of cladribine has on the living system in terms of body distribution, modification, metabolism, and excretion by the use of several analytical strategies such as metabolome analysis with a Fourier-transform ion cyclotron resonance-mass spectrometer (FT-ICR-MS)8 and metabolic profiling using nuclear magnetic resonance (NMR).9 In the organic synthesis, Robins et al. reported an effective synthetic approach for forming unlabeled cladribine using 2′-deoxyguanosine as the starting material, which is, however, very expensive among the commercially available nucleic acid reagents.10 Therefore, by the application of the synthetic process for the above-described [6-15N]-2′-deoxyadenosine (1), we succeeded for the first time in synthesizing a nitrogen-stable isotope-labeled novel compound [6-15N]-cladribine (2) from the commercially available and inexpensive ribonucleoside, guanosine.
An alternative synthetic process of [6-15N]-2′-deoxyadenosine (1)
Compound 3 had previously been synthesized in five steps by Maruyama et al.4,5,11 from the starting material inosine (Scheme 1). First, the deoxygenation of compound 3 was conducted at the 2′-position of the hydroxyl group by the Barton-McCombie method using silicon.12 Compound 3 was then esterified with phenyl chlorothionoformate13 to give product 4 in 91% yield. Then, the resulting compound 4 was treated with diphenylsilane4,5 in the presence of 2,2′-azobis(isobutyronitrile) (AIBN) under a nitrogen atmosphere, providing the corresponding deoxidized product 5 in 85% yield. Next, we attempted the general reaction for the amination at the 6-position in the purine skeleton under the methanol solvent condition.14 The 6-chloropurine riboside analog 5 was treated with [15N]-NH4Cl and KHCO3 in methanol solution in the sealed tube at 100 °C, furnishing the [6-15N]-amino-3′-debenzoylated product 7 in 82% yield. Detritylation was then performed with 5% trifluoroacetic acid in chloroform solution,15 which unfortunately resulted in the decomposition into adenine due to cleavage of the glycoside bond. To overcome this challenge, we changed of our point of view and assumed that the free 3′-hydroxyl group in the pentose system of compound 7 accelerated the nucleoside degradation under the acidic condition, hence, 5′-detritylation should be carried out before the 3′-debenzoylation. That is, during the substitution reaction to form the [15N]-amino group at the 6-position in the purine moiety of 5, we examined the feasibility of carrying out the reaction condition without undergoing the 3ꞌ-debenzoylation: compound 5 was treated with 2.0 and 3.0 equivalents of [15N]-NH4Cl and KHCO3 in the DMSO solution in a sealed tube at 80 °C.15 As an interesting result, [6-15N]-aminated product 6 was obtained in 99% yield without undergoing simultaneous 3′-debenzoylation. This consequence suggested that in a DMSO solvent,
[15N]-ammonia, which is generated by [15N]-NH4Cl and KHCO3 in the reactive system, essentially failed to conduct a nucleophilic attack on the carbonyl carbon at the 3′-benzoyl group. It is noteworthy that this is the first reported conversion of amination at the 6-position of a 6-chloropurine riboside analog whose sugar alcohol is protected by a benzoyl group without undergoing deprotection of the benzoyl group at the sugar hydroxyl moiety. There are two advantages to this approach: i) It enables the minimum usage of a stable-labeled compound, [15N]-NH4Cl, which is a relatively expensive reagent, without consuming the debenzoylation, ii) It allows the selective deprotection at the 3′ or 5′ protecting group of compound 6 because of negligible debenzoylation. The resulting compound 6 was treated with 5% trifluoroacetic acid in chloroform to give predictably the detritylated product 8 in 94% yield without any decomposition.16 This result demonstrated that the deoxyadenosine derivative 6, whose pentose alcohol at the 3′-position is protected by a benzoyl group, is less likely to experience cleavage of the glycoside linkage in comparison to the case of the detritylation of 7. Thus, we were able to synthesize [6-15N]-2′-deoxyadenosine (1) by carrying out the 3′-debenzoylation according to the previous method that utilized a methanolic ammonia solution.17 Meanwhile, the spectrum data of 1 were found to be in identical with the corresponding previous data.1
First synthesis of [6-15N]-2-chloro-2′-deoxyadenosine ([6-15N]-cladribine, 2)
[6-15N]-cladribine (2) synthesis began with guanosine as a starting material, which led to the 6-chloro analog 9 in three steps according to a previous report (Scheme 2).18 The resulting 9 was added to dibutyltin oxide (IV), then we carried out the monobenzoylation11 with benzoyl chloride, followed by separation on silica-gel chromatography, providing the desired 3′-O-benzoate 10a and its regioisomer 2′-O-benzoate 10b in 61% and 9% yields respectively. Next, formation of the desired product 10a was carried out by the 5′-selective silylation using one equivalent of tert-butyldimethylsilyl chloride (TBSCl), imidazole, and DMF, to give the corresponding 5′-silylated inseparable mixture of 11a and 11b in 94% combined yield (ratio 11a/11b: 78/22).19 Since 10a was identified as a pure single compound by 1H-NMR analysis, we assumed that the acyl migration of 10a occurred during the 5′-silylated reaction.4,5 Following deoxygenation by the above-described Barton-McCombie method,12 phenoxythiocarbonylation of the hydroxyl group at the 2′- or 3′-position was carried out to obtain the corresponding ester 12a and 12b as the inseparable regioisomeric mixture in 89% combined yield (ratio 12a/12b: 79/21).13 Then, the mixture of 12a and 12b was treated with diphenylsilane in the presence of AIBN under the nitrogen atmosphere,4,5 followed by the separation of silica-gel,
providing 3′-O-benzoate 13a as the isolated product and the corresponding 2′-O-benzoate 13b in 69% and 21% yields, respectively. The resulting 13a was reacted with benzyltriethylammonium chloride, dichloroacetic acid, antimony trichloride, and sodium nitrite in dichloromethane solution to provide the 2,6-dichloropurine riboside analog 14 in 90% yield, reported by Robins et al.10 In the same manner as the [6-15N]-aminated reaction in Scheme 1, compound 14 was converted into the [6-15N]-6-amino-nucleoside 15 without undergoing the debenzoylation at the 3′-positon by the use of [15N]-NH4Cl and KHCO3 in DMSO solution. The 1H-NMR spectrum of 15 revealed an amino proton signal at δ 7.82 (2H, d, J = 90.8 Hz), supporting evidence of the presence of a [15N]-labeled amino group at the 6-positon in the purine skeleton in 15. Finally, deprotective reactions of TBS group at the 5′-position and benzoyl group at the 3′-positon were carried out in high yields, providing the objective compound, [6-15N]-cladribine (2). The molecular formula of 2 was determined to be C10H12CIN415NNaO3 from the HRMS (ESI) at m/z 309.04885 [M+Na]+ (Calcd for 309.04912). In its 1H-NMR spectrum, there were significant differences in the chemical shifts for the 15N-labelled amino group at the 6-position of the purine system, δ 7.75 (2H, d, J = 90.8 Hz), in comparison to the previous report for unlabelled cladribine about 1H, 13C-NMR, and HRMS data,20 suggesting the introduction of [6-15N]-aminated cladribine, namely 2.
EXPERIMENTAL
Instrumentation
1H-, 13C- and 15N-NMR spectra were taken with a UltrashieldTM 400 Plus FT NMR System (BRUKER). Chemical shifts and coupling constants (J) were given in d and Hz, respectively. High-resolution mass spectrometry (HRMS) was performed on a APEX IV mass spectrometer (BRUKER) with electrospray ionization mass spectroscopy (ESI-MS). Melting points (mp) were determined using a YANACO micro-melting point apparatus (MP-500D) and are uncorrected.
9-(3-O-Benzoyl-2-O-phenoxythiocarbonyl-5-O-trityl-β-D-ribofuranosyl)-6-chloropurine (4)
Compound 3 (633.1 mg, 1.00 mmol) was dissolved in dry CH2Cl2 (10.0 mL), under nitrogen atmosphere. To this stirred solution was carefully added ClC(S)(OPh) (220.0 µL, 1.63 mmol) and DMAP (273.0 mg, 2.33 mmol). Stirring was continued at room temperature for 0.5 h and the mixture was extracted with AcOEt. The organic extracts were washed with water, saturated aqueous sodium chloride solution, and dried with sodium sulfate, and then evaporated. The residue was purified by silica gel column chromatography (33% AcOEt in hexane) to give 4 as oil (700.0 mg, 0.91 mmol, 91%). 1H-NMR (400MHz, CDCl3): δ 8.72 (1H, s, H-8), 8.39 (1H, s, H-2), 8.14 (2H, m, 3′O-Bz), 7.66 (1H, m, 3′O-Bz), 6.96−7.55 (22H, m, 3′O-Bz, 2′O-Ph, 5′O-Tr), 6.79 (1H, dd, J = 6.0 and 5.6, H-2′), 6.63 (1H, d, J = 6.4, H-1′), 6.27 (1H, dd, J = 5.6 and 3.2, H-3′), 4.63 (1H, m, H-4′), 3.66 (1H, dd, J = 10.8 and 3.2 Hz, H-5′a), 3.61 (1H, dd, J = 10.4 and 3.2 Hz, H-5′b); HRMS (ESI) Calcd for C43H33ClN4NaO6S [M+Na]+: 791.17015. Found 791.170100.
9-(3-O-Benzoyl-5-O-trityl-β-D-2-deoxyribofuranosyl)-6-chloropurine (5)
Compound 4 (689.0 mg, 0.89 mmol) was dissolved in toluene (4.0 mL), and AIBN (60.0 mg, 0.37 mmol) and diphenylsilane (0.6 mL, 1.95 mmol) was added to the solution, and then stirred for 13 h at 100 °C under nitrogen atmosphere. The mixture was then extracted with AcOEt, and the organic extracts were washed with saturated aqueous sodium chloride solution, and dried with sodium sulfate, and then evaporated. The residue was purified by silica gel column chromatography (50% AcOEt in hexane) to give crystals 5 (392.7 mg, 0.63 mmol, 71%). 1H-NMR (400MHz, CDCl3): δ 8.67 (1H, s, H-8), 8.33 (1H, s, H-2), 8.08 (2H, m, 3′O-Bz), 7.66 (1H, m, 3′O-Bz), 7.48 (2H, m, 3′O-Bz), 7.20-7.44 (15H, m, 5′O-Tr), 6.59 (1H, dd, J = 8.4 and 6.0 Hz, H-1′), 5.81 (1H, m, H-3′), 4.50 (1H, m, H-4′), 3.58 (1H, dd, J = 10.4 and 4.0 Hz, H-5a′), 3.49 (1H, dd, J = 10.4 and 4.0 Hz, H-5b′), 3.66 (1H, ddd, J = 14.0, 8.0 and 6.0 Hz, H-2′a), 2.85 (1H, ddd, J = 14.0, 6.0 and 2.0 Hz, H-2′b); HRMS (ESI) Calcd for C36H29ClN4NaO4 [M+Na]+: 639.17695; Found 639.17513; mp 80.1−84.5 °C.
[6-15N]-3′-Benzoyl-5′-trityldeoxyadenosine (6)
Compound 5 (124.0 mg, 0.2 mmol) was dissolved in DMSO (3.0 mL), and 15NH4Cl (21.7 mg, 0.4 mmol), KHCO3 (60.0 mg, 0.6 mmol) was added to the solution, and then sealed and stirred for 2 d at 100 °C. The mixture was extracted with AcOEt, and washed with saturated aqueous sodium chloride solution, and dried with sodium sulfate, and then evaporated. The residue was purified by silica gel column chromatography (10% MeOH in AcOEt) to give crystals 6 (118.8 mg, 0.20 mmol, 99%). 1H-NMR (400MHz, CDCl3): δ 8.31 (1H, s, H-8), 8.07 (2H, m, 3′O-Bz), 8.01 (1H, s, H-2), 7.62 (1H, m, 3′O-Bz), 7.44 (2H, m, 3′O-Bz), 7.20-7.41 (15H, m, 5′O-Tr), 6.55 (1H, dd, J = 8.4 and 5.6 Hz, H-1′), 5.77 (1H, m, H-3′), 5.77 (1H, d, J = 90.0 Hz, 15NH2), 4.45 (1H, m, H-4′), 3.50-3.65 (2H, m, H-5′ab), 2.78 (1H, m, H-2′a), 2.75 (1H, m, H-2′b); HRMS (ESI) Calcd for C36H31N415NNaO4 [M+Na]+: 621.22386. Found 621.21765; mp 99.9−101.8 °C.
[6-15N]-5′-trityl-2′-deoxyadenosine (7)
Compound 5 (617.1 mg, 1.0 mmol) was dissolved in MeOH (15.0 mL), and 15NH4Cl (1,000 mg, 18.4 mmol), KHCO3 (1.84 g, 18.4 mmol) was added to the solution, and then sealed and stirred for 1 d at 100 °C. The mixture was extracted with AcOEt, and washed with saturated aqueous sodium chloride solution, and dried with sodium sulfate, and then evaporated. The residue was purified by silica gel column chromatography (25% MeOH in CH2Cl2) to give crystals 7 (405.7 mg, 0.82 mmol, 82%). 1H-NMR (400MHz, DMSO-d6): δ 8.23 (1H, s, H-8), 8.07 (1H, s, H-2), 7.20-7.28 (15H, m, 3′O-Tr), 7.26 (1H, d, J = 90.0 Hz, 15NH2), 6.37 (1H, m, H-1′), 5.37 (1H, d, J = 4.4 Hz, 3′=OH), 4.49 (1H, m, H-3′), 3.39 (1H, m, H-4′), 3.15-3.22 (2H, m, H-5′ab), 2.88 (1H, m, H-2′a), 2.32 (1H, m, H-2′b); HRMS (ESI) Calcd for C29H27N415NNaO3 [M+Na]+: 517.19765. Found 517.19832; mp 193.2−195.0 °C.
[6-15N]-3′-Benzoyl-2′-deoxyadenosine (8)
Compound 6 (1180.0mg, 1.97 mmol) was dissolved in dry CHCl3 (60.0 mL) and CF3CO2H (3.0 mL) was added to the solution, and then stirred for 5 min at room temperature. The reaction was quenched with NaHCO3 (6.7g, 80.0 mmol), and the mixture was evaporated in vacuo and the residue was purified by silica gel column chromatography (14% MeOH in CHCl3) to give a crystal 8 (660.0 mg, 1.85 mmol, 94%). 1H-NMR (400MHz, DMSO-d6): δ 8.38 (1H, s, H-8), 8.31 (1H, s, H-2), 8.06 (2H, m, 3′O-Bz), 7.71 (1H, m, 3′O-Bz), 7.58 (2H, m, 3′O-Bz), 7.29 (2H, d, J = 90.0 Hz, 15NH2), 6.48 (1 H, dd, J = 8.8 and 5.6 Hz, H-1′), 5.64 (1H, m, H-3′), 5.57 (1H, dd, J 6.8 and 4.8 Hz, 5′6OH), 4.27 (1H, m, H-4′), 3.65-3.68 (2H, m, H-5′ab), 3.08 (1H, m, H-2′a), 2.63 (1H, m, H-2′b); HRMS (ESI) Calcd for C17H17N415NNaO4 [M+Na]+: 379.11431. Found 379.11191; mp 248.0−249.5 °C.
[6-15N]-2′-Deoxyadenosine (1)
Compound 8 (997.2 mg, 2.80 mmol) was dissolved in 2N NH3/MeOH (50.0 mL), and then sealed and stirred for 18 h at 100 °C. The mixture was evaporated, and the residue was purified by silica gel column chromatography (25% MeOH in CHCl3) to give crystals 1 (505.3 mg, 2.00 mmol, 72%). 1H-NMR (400MHz, DMSO-d6): δ 8.31 (1H, s, H-8), 8.11 (1H, s, H-2), 7.29 (2H, d, J = 90.0 Hz, 15NH2), 6.32 (1H, dd, J = 7.6 and 6.0 Hz, H-1′), 5.29 (1H, d, J = 4.0 Hz, 3′=OH), 5.22 (1H, dd, J = 6.8 and 4.8 Hz, 3′=OH), 4.39 (1H, m, H-3′), 3.86 (1H, m, H-4′), 3.60 (1H, ddd, J = 12.0, 9.2 and 4.8 Hz, H-5′a), 3.50 (1H, ddd, J = 11.6, 6.8, and 4.4 Hz, H-5′b), 2.71 (1H, ddd, J = 13.2, 7.6 and 5.6 Hz, H-2′a), 2.23 (1H, ddd, J = 13.2, 6.0 and 2.8 Hz, H-2′b); 13C-NMR (100MHz, CD3OD): δ 156.1 (d, J = 21.0 Hz, C6), 152.1 (d, J = 2.0 Hz, C2), 148.5, 140.1, 119.4 (d, J = 3.0 Hz, C5), 88.5, 85.7, 71.6, 62.2, 40.1; 15N-NMR (40MHz, CD3OD): δ 83.8 (s, 15NH2); HRMS (ESI) Calcd for C10H13N415NNaO3 [M+Na]+: 275.08810. Found 275.08800; mp 185.1−185.8 °C.
2-Amino-9-(3-O-benzoyl-β-D-ribofuranosyl)-6-chloropurine (10a) and 2-Amino-9-(2-O-benzoyl-β-D-ribofuranosyl)-6-chloropurine (10b)
2-Amino-6-chloro-9-(β-D-ribofuranosyl)purine (9) (286.8 mg, 1.00 mmol) and di-n-butyltin (IV) oxide (249.2 mg, 1.00 mmol) was dissolved in MeOH (10.0 mL) was refluxed for 1 h, then added triethylamine (0.70 mL, 5.00 mmol) and benzoyl chloride (0.58 mL, 5.00 mmol) and was stirred for 0.5 h at room temperature. The mixture was dissolved in AcOEt, and then extracted with AcOEt. The organic extracts were washed with saturated aqueous sodium chloride solution, and dried with sodium sulfate. After removal of the organic solvent, the residue was purified by silica gel column chromatography (AcOEt) to give 3ive ied by si analogue (10a) as white crystals (182.9 mg, 0.48 mmol, 61%). Evaporation of the second fraction gave 2vaporation of analogue (10b) as by-product and white crystals (22.4 mg, 0.05 mmol, 9%). 10a: 1H-NMR (400MHz, DMSO-d6): δ 8.45 (1H, s, H-8), 8.05 (2H, m, 3′O-Bz), 7.72 (1H, m, 3′O-Bz), 7.58 (2H, m, 3′O-Bz), 7.03 (2H, brs, NH2), 5.92-5.97 (2H, m, H-1′, 2′-OH), 5.53 (1H, dd, J = 5.6 and 2.4 Hz, H-3′), 5.30 (1H, t, J = 5.2, 5′-OH), 4.93 (1H, m, H-2′), 4.29 (1H, m, H-4′), 3.66-3.78 (2H, m, H-5′ab); HRMS (ESI) Calcd for C17H16ClN5NaO5 [M+Na]+: 428.07322. Found 428.07436; mp 130.2− 132.0 °C. 10b: 1H-NMR (400MHz, DMSO-d6): δ 8.46 (1H, s, H-8), 7.98 (2H, m, 2′O-Bz), 7.68 (1H, m, 2′O-Bz), 7.56 (2H, m, 2′O-Bz), 7.02 (2H, brs, NH2), 6.24 (1H, d, J = 5.2 Hz, H-1′), 5.69-5.77 (2H, m, H-2′ and 3′-OH), 5.19 (1H, t, J = 5.2 Hz, 5′-OH), 4.59 (1H, m, H-3′), 4.09 (1H, m, H-4′), 3.62-3.81 (2H, m, H-5′ab).
2-Amino-9-(3-O-benzoyl-5-O-tert-butyldimetylsilyl-β-D-ribofuranosyl)-6-chloropurine (11a) and 2-Amino-9-(2-O-benzoyl-5-O-tert-butyldimetylsilyl-β-D-ribofuranosyl)-6-chloropurine (11b)
Compound 10a (387.3 mg, 0.95 mmol) was dissolved in dry DMF (4.0 mL) and imidazole (152.4 mg, 2.24 mmol) and tert-butyldimetylchlorosilane (143.9 mg, 0.95 mmol) were added to the solution, and then stirred for 20 h at room temperature. The mixture was extracted with AcOEt. The organic extracts were washed with water, saturated aqueous sodium chloride solution, and dried with sodium sulfate, and then evaporated. The residue was purified by silica gel column chromatography (33% AcOEt in hexane) to give the inseparable mixture of 11a and 11b as a crystal (463.6 mg, 0.89 mmol, 94% combined yield), ratio 78:22 according to 1H-NMR spectrum. 1H-NMR (400MHz, CDCl3): δ 8.10 (0.2H, s, H-8, 11b), 8.06 (0.8H, s, H-8, 11a), 7.99-8.04 (1.6H, m, 3′O-Bz, 11a), 7.90-7.95 (0.4H, m, 2′O-Bz, 11b), 7.44-7.53 (1H, m, 2′O-Bz of 11b and 3′O-Bz of 11a), 7.31-7.39 (2H, m, 2′O-Bz of 11b and 3′O-Bz of 11a), 6.23 (0.2H, d, J = 5.2 Hz, H-1′, 11b), 5.98 (0.8H, d, J = 6.4 Hz, H-1′, 11a), 5.67 (0.2H, m, H-2′, 11b), 5.53 (0.8H, dd, J = 5.6 and 2.4 Hz, H-3′, 11a), 4.78 (0.8H, m, H-2′, 11a), 4.71 (0.2H, m, H-3′, 11b), 4.39 (0.8H, m, H-4′, 11a), 4.22 (0.2H, m, H-4′, 11b), 3.88-3.96 (0.4H, m, H-5′ab, 11b), 3.86 (1.6H, m, H-5′ab, 11a), 0.85 (1.8H, s, 5′O-TBS, 11b), 0.80 (7.2H, s, 5′O-TBS, 11a), 0.06 (0.6H, s, 5′O-TBS, 11b), 0.05 (0.6H, s, 5′O-TBS, 11b), 0.03 (2.4H, s, 5′O-TBS, 11a), 0.00 (2.4H, s, 5′O-TBS, 11a); HRMS (ESI) Calcd for C23H30ClN5NaO5 [M+Na]+: 542.15969. Found 542.16198.
2-Amino-9-(3-O-benzoyl-2-O-phenoxythiocarbonyl-5-O-tert-butyldimetylsilyl-β-D-ribofuranosyl)-6- chloropurine (12) and 2-Amino-9-(2-O-benzoyl-3-O-phenoxythicarbonyl-5-O-tert-butyldimetylsilyl- β-D-ribofuranosyl)-6-chloropurine (12b)
The mixture of 11a and 11b (463.6 mg, 0.89 mmol) was dissolved in dry CH2Cl2 (7.26 mL), under nitrogen atmosphere. To this stirred solution was carefully added ClC(S)(OPh) (254.1 µL, 1.42 mmol) and DMAP (261.4 mg, 2.14 mmol). Stirring was continued at room temperature for 1 h and the mixture was extracted with AcOEt. The organic extracts were washed with water, saturated aqueous sodium chloride solution, and dried with sodium sulfate, and then evaporated. The residue was purified by silica gel column chromatography (50% AcOEt in hexane) to give the inseparable mixture of 12a and 12b as a crystal (411.6 mg, 0.79 mmol, 89% combined yield), ratio 79:21 according to 1H-NMR spectrum. 1H-NMR (400MHz, CDCl3): δ 8.23 (0.8H, s, H-8, 12a), 8.20 (0.2H, s, H-8, 12b), 8.15 (1.6H, m, 3′O-Bz, 12a), 8.07 (0.4H, m, 2′O-Bz, 12b), 7.66 (0.8H, m, 3′O-Bz, 12a), 7.61 (0.2H, m, 2′O-Bz, 12b), 7.53 (1.6H, m, 3′O-Bz, 12a), 7.46 (0.4H, m, 2′O-Bz, 12b), 6.35−7.41 (5H, m, Ph of 12a and 12b), 6.51 (0.8H, d, J = 7.2 Hz, H-1′, 12a), 6.45 (0.2H, d, J = 6.4 Hz, H-1′, 12b), 6.38 (0.8H, dd, J = 7.2 and 5.6 Hz, H-2′, 12a), 6.18 (0.2H, dd, J = 5.2 and 2.4 Hz, H-3′, 12b), 6.11 (0.2H, dd, J = 6.4 and 5.2 Hz, H-2′, 12b), 6.02 (0.8H, dd, J = 5.6 and 2.0 Hz, H-3′, 12a), 5.12 (1.6H, brs, NH2, 12a), 5.05 (0.4H, brs, NH2, 12b), 4.64 (0.2H, m, H-4′, 12b), 4.53 (0.8H, m, H-4′, 12a), 4.04−4.06 (0.4H, m, H-5′ab, 12b), 4.00−4.04 (1.6H, m, H-5′ab, 12a), 0.97 (1.8H, s, 5′O-TBS, 12b), 0.95 (7.2H, s, 5′O-TBS, 12a), 0.19 (0.6H, s, 5′O-TBS, 12b), 0.17 (2.4H, s, 5′O-TBS, 12a), 0.16 (2.4H, s, 5′O-TBS, 12a), 0.14 (0.6H, s, 5′O-TBS, 12b); HRMS (ESI) Calcd for C30H34ClN5NaO6SSi [M+Na]+: 678.15798. Found 678.15932.
2-Amino-6-chloro-9-(3-O-benzoyl-5-O-tert-butyldimetylsilyl-β-D-ribofuranosyl)purine (13a) and 2-Amino-6-chloro-9-(2-O-benzoyl-5-O-tert-butyldimetylsilyl-β-D-ribofuranosyl)purine (13b)
The mixture of 12a and 12b (393.0 mg, 0.51 mmol) was dissolved in toluene (2.3 mL), and AIBN (34.1 mg, 0.21 mmol) and diphenylsilane (0.6 mL, 1.95 mmol) was added to the solution, and then stirred for 7 h at 100 °C under nitrogen atmosphere. The mixture was then extracted with AcOEt, and the organic extracts were washed with saturated aqueous sodium chloride solution, and dried with sodium sulfate, and then evaporated. The residue was purified by silica gel column chromatography (50% AcOEt in hexane) to give 3vefied by 13a as crystals (209.5 mg, 0.42 mmol, 69%). Evaporation of the second fraction gave 2vaporation 13b as crystals (64.6 mg, 0.13 mmol, 21%). 13a: 1H-NMR (400MHz, CDCl3): δ 8.20 (1H, s, H-8), 8.08 (2H, m, 3′O-Bz), 7.62 (1H, m, 3′O-Bz), 7.49 (2H, m, 3′O-Bz), 6.47 (1H, dd, J = 8.4 and 6.0 Hz, H-1′), 5.66 (1H, m, H-3′), 5.09 (2H, brs, NH2), 4.37 (1H, m, H-4′), 4.01 (1H, dd, J = 11.2 and 2.4 Hz, H-5′a), 3.95 (1H, dd, J = 11.2 and 2.4 Hz, H-5′b), 2.74−2.78 (2H, m, H-2′ab), 0.93 (9H, s, 5′O-TBS), 0.15 (6H, m, 5′O-TBS); HRMS (ESI) Calcd for C23H30ClN5O4Si [M+Na]+: 526.16478. Found 526.16563; mp 67.7−68.4 °C. 13b: 1H-NMR (400MHz, CDCl3): δ 8.23 (1H, s, H-8), 8.08 (2H, m, 2′O-Bz), 7.62 (1H, m, 2′O-Bz), 7.49 (2H, m, 2′O-Bz), 6.20 (1H, d, J = 1.6 Hz, H-1′), 5.83 (1H, m, H-2′), 5.03 (2H, brs, NH2), 4.58 (1H, m, H-4′), 4.07 (1H, dd, J = 11.2 and 2.8 Hz, H-5′a), 3.79 (1H, dd, J = 11.2 and 2.8 Hz, H-5′b), 2.66 (1H, m, H-3′a), 2.26 (1H, dd, J = 5.6 and 3.6 Hz, H-3′b), 0.92 (9H, s, 5′O-TBS), 0.15 (3H, m, 5′O-TBS), 0.15 (3H, m, 5′O-TBS); HRMS (ESI) Calcd for C23H30ClN5O4Si [M+Na]+:526.16478. Found 526.16619; mp 92.0−95.1 °C.
9-(3-O-Benzoyl-5-O-tert-butyldimetylsilyl-β-D-ribofuranosyl)-2,6-dichloropurine (14)
SbCl3 (684 mg 3 mmol) in CH2Cl2 (7.5 mL) was added to a mixture of compound 13a (756.0 mg, 1.5 mmol), benzyltriethylammonium chloride (340.5 mg 1.5 mmol), and NaNO2 (2.1 g, 30 mmol) in CH2Cl2 (60 mL). Cl2CHCO2H (246 µL, 6 mmol) was added, and the flask was flushed with dried N2 and sealed, and the mixture was stirred at room temperature. The reaction was completed after 17 h, and celite (3 g) and CHCl3 were added, and filtered. The filtrate was then extracted with CHCl3, and the organic extracts were washed with saturated aqueous sodium chloride solution, and dried with sodium sulfate, and then evaporated. The residue was purified by silica gel column chromatography (AcOEt) to give crystals 14 (706.3 mg, 1.35 mmol, 90%). 1H-NMR (400MHz, CDCl3): δ 8.62 (1H, s, H-8), 8.09 (2H, m, 3′O-Bz), 7.63 (1H, m, 3′O-Bz), 7.50 (2H, m, 3′O-Bz), 6.65 (1H, dd, J = 8.4 and 6.0 Hz, H-1′), 5.66 (1H, m, H-3′), 4.43 (1H, m, H-4′), 3.98−4.07 (2H, m, H-5′ab), 2.88 (1H, m, H-2′a), 2.74 (1H, m, H-2′b), 0.96 (9H, s, 5′O-TBS), 0.16 (6H, m, 5′O-TBS); HRMS (ESI) Calcd for C23H28Cl2N4NaO4Si [M+Na]+: 545.11491. Found 545.11255; mp 141.2−142.6 °C.
[6-15N]-9-(3-O-Benzoyl-5-O-tert-butyldimetylsilyl-β-D-ribofuranosyl)-2-chloropurine (15)
Compound 14 (52.3 mg, 0.10 mmol) was dissolved in DMSO (5.0 mL), and 15NH4Cl (54.4 mg, 1.0 mmol), KHCO3 (144.0 mg, 1.44 mmol) was added to the solution, and then sealed and stirred for 24 h at 100 °C. The mixture was extracted with AcOEt, and washed with saturated aqueous sodium chloride solution, and dried with sodium sulfate, and then evaporated. The residue was purified by silica gel column chromatography (50% AcOEt in hexane) to give crystals 15 (37.4 mg, 0.07 mmol, 74%). 1H-NMR (400MHz, DMSO-d6): δ 8.31 (1H, s, H-8), 8.02 (2H, m, 3′O-Bz), 7.82 (2H, d, J = 90.8 Hz, 15NH2), 7.66 (1H, m, 3′O-Bz), 7.53 (2H, m, 3′O-Bz), 6.37 (1H, dd, J = 8.0 and 6.0 Hz, H-1′), 5.58 (1H, m, H-3′), 4.25 (1H, m, H-4′), 3.88 (1H, dd, J = 11.2 and 4.8 Hz, H-5′a), 3.81 (1H, dd, J = 11.2 and 4.8 Hz, H-5′b), 3.02 (1H, m, H-2′a), 2.68 (1H, ddd, J = 14.0, 6.0 and 2.0 Hz, H-2′b), 0.80 (9H, s, 5′O-TBS), 0.01 (6H, m, 5′O-TBS); HRMS (ESI) Calcd for C23H30CIN415NNaO4Si [M+Na]+: 527.16181. Found 527.16227; mp 175.2−176.9 °C.
[6-15N]-9-(3-O-Benzoyl-β-D-ribofuranosyl)-2-chloropurine (16)
Compound 15 (50.5 mg, 0.10 mmol) was dissolved in THF (3.0 mL), and 1.0N tetrabutylammonium fluoride-THF solution (1.0 mL) was added to the solution, and then stirred for 5 min at room temperature. The mixture was extracted with AcOEt, and washed with saturated aqueous sodium chloride solution, and dried with sodium sulfate, and then evaporated. The residue was purified by silica gel column chromatography (14% MeOH in CH2Cl2) to give crystals 16 (39.0 mg, 0.10 mmol, 100%). 1H-NMR (400MHz, DMSO-d6): δ 8.07 (2H, m, 3′O-Bz), 7.97 (1H, s, H-8), 7.70-7.90 (2H, d, J = 90.8 Hz, 15NH2), 7.62 (1H, m, 3′O-Bz), 7.58 (2H, m, 3′O-Bz), 6.37 (1H, dd, J = 9.6 and 5.2 Hz, H-1′), 5.50 (1H, m, H-3′), 4.43 (1H, m, H-4′), 4.03−4.04 (2H, m, H-5′ab), 3.17−3.23 (1H, m, H-2′a), 2.63 (1H, m, H-2′b); HRMS (ESI) Calcd for C17H16CIN415NNaO4 [M+Na]+: 413.07534. Found 413.07563; mp 192.1−193.6 °C.
[6-15N]-2-Chloro-2'-deoxyadenosine ([6-15N]-Cladribine, 2)
Compound 16 (36.0 mg, 0.09 mmol) was dissolved in 2N NH3/MeOH, then kept for 12 h at room temperature. After condensation of the solution, the residue was purified by silica gel column chromatography (25% MeOH in CH2Cl2) to give crystals 2 (23.7 mg, 0.08 mmol, 91%). 1H-NMR (400MHz, DMSO-d6): δ 8.29 (1H, s, H-8), 7.75 (2H, d, J = 90.8 Hz, NH2), 6.19 (1H, dd, J = 6.4 and 6.4 Hz, H-1′), 5.24 (1H, m, 3′-OH), 4.89 (1H, m, 5′-OH), 4.32 (1H, m, H-3′), 3.79 (1H, m, H-4′), 3.52−3.55 (1H, m, H-5′a), 3.43-3.46 (1H, m, H-5′b), 2.54−2.61 (1H, m, H-2′a), 2.18−2.24 (1H, ddd, J = 9.2, 6.0 and 3.2 Hz, H-2′b); 13C-NMR (100MHz, DMSO-d6): δ 156.7 (d, J = 21.0 Hz, C6), 152.9 (d, J = 3.0 Hz, C2), 150.0, 139.8, 118.1 (d, J = 3.0 Hz, C5), 87.9, 83.5, 70.7, 61.6, 40.1; 15N-NMR (40MHz, CD3OD): δ 83.7 (s, 15NH2); HRMS (ESI) Calcd for C10H12CIN415NNaO3 [M+Na]+: 309.04912. Found 309.04885; mp 215.9− 219.0 °C.
References
1. X. Gao and R. A. Jones, J. Am. Chem. Soc., 1987, 109, 1275. CrossRef
2. J. Kelly, D. A. Ashburn, R. Michalczyk, and L. A. Sikls, III, J. Labelled Comp. Radiopharm., 1995, 36, 631. CrossRef
3. B. L. Gaffney, P. P. Kung, and R. A. Jones, J. Am. Chem. Soc., 1990, 112, 6748. CrossRef
4. S. Takamatsu, T. Maruyama, S. Katayama, N. Hirose, and K. Izawa, Tetrahedron Lett., 2001, 42, 2321. CrossRef
5. T. Maruyama, S. Takamatsu, S. Kozai, Y. Satoh, and K. Izawa, Chem. Pharm. Bull., 1999, 47, 966. CrossRef
6. P. Hentosh and D. M. Peffley, Expert Opinion on Drug Metabolism & Toxicology, 2010, 6, 75. CrossRef
7. D. N. E. Van, S. Cardoen, F. Offner, and F. Bontemps, Int. J. Oncol., 2005, 27, 1113.
8. D. Ohta, S. Kanaya, and H. Suzuki, Current Opinion in Biotech., 2010, 21, 35. CrossRef
9. P. M. Joyner, R. M. Matheke, L. M. Smith, and R. H. Cichewicz, J. Proteome Res., 2010, 9, 404. CrossRef
10. Z. Janeba, P. Francom, and M. J. Robins, J. Org. Chem., 2003, 68, 989. CrossRef
11. V. Nair, D. A. Young, and R. J. DeSilvia, J. Org. Chem., 1987, 52, 1344. CrossRef
12. D. H. R. Barton and S. W. McCombie, J. Chem. Soc., Perkin Trans. 1, 1975, 16, 1574. CrossRef
13. M. J. Robins, J. S. Wilson, and F. Hansske, J. Am. Chem. Soc., 1983, 105, 4059. CrossRef
14. E. Lee-Ruff, M. Ostrowski, A. Ladha, D. V. Stynes, I. Vernik, J.-L. Jiang, W.-Q. Wan, S.-F. Ding, and S. Joshi, J. Med. Chem., 1996, 39, 5276. CrossRef
15. E. M. B. Janke, H.-H. Limbach, and K. Weisz, J. Am. Chem. Soc., 2004, 126, 2135. CrossRef
16. A. K. Pathak, V. Pathak, L. E. Seitz, K. N. Tiwari, M. S. Akhtar, and R. C. Reynolds, Tetrahedron Lett., 2001, 42, 7755. CrossRef
17. M. V. Baud, C. Chavis, M. Lucas, and J. L. Imbach, Tetrahedron Lett., 1990, 31, 4437. CrossRef
18. V. Nair and T. B. Sells, Synlett, 1991, 753. CrossRef
19. S. Debarge, J. Balzarini, and A. R. Maguire, J. Org. Chem., 2011, 76, 105. CrossRef
20. V. L. Worthington, W. Fraser, and C. H. Schwalbe, Carbohyd. Res., 1995, 275, 275. CrossRef