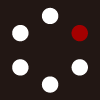
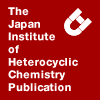
HETEROCYCLES
An International Journal for Reviews and Communications in Heterocyclic ChemistryWeb Edition ISSN: 1881-0942
Published online by The Japan Institute of Heterocyclic Chemistry
e-Journal
Full Text HTML
Received, 2nd November, 2011, Accepted, 8th December, 2011, Published online, 15th December, 2011.
DOI: 10.3987/COM-11-12385
■ PEG-400-Promoted and Ultrasound Assisted Rap-Stoermer Reaction for Efficient Synthesis of Benzofuran-2-yl(carbazolyl)methanone Derivatives
Yang Li, Yan Yan, and Wentao Gao*
Institute of Superfine Chemicals, School of Chemistry and Chemical Engineering, Bohai University, 19 Keji Road, Jinzhou 121000, China
Abstract
A facile synthesis of hitherto unreported (9-methyl-9H-carbazole-3,6-diyl)bis(benzofuran-2-yl-methanone)s (3a-f) is described, featuring the PEG-400-promoted and ultrasound-asisted Rap-Stoermer reaction of 3,6-dichloroacetyl-9-methyl-9H-carbazole (1) with a variety of salicylaldehydes as well as 2-hydroxy-1-naphthaldehyde (2) in the presence of K2CO3 as the base in acetonitrile. This procedure offers easy access to benzofuran-2-yl(carbazolyl)methanone derivatives in short reaction time and the products are achieved in good yields.Carbazole especially heterocycle-containing carbazole derivatives, are embodied in many naturally occurring products1-3 and display broad spectrum of useful biological activities such as anti-tumor, antimitotic, and antioxidative activities.4-6 Therefore, a number of methodologies for the construction of heterocycle-containing carbazole have been reported in recent years.7-11 Most heterocycle-containing carbazoles reported in literatures contain a common heterocyclic ring moiety fused with a carbazole ring such as pyridocarbazoles,12,13 thienocarbazoles,14,15 pyranocarbazoles, pyrrolocarbazoles,16,17 indolocarbazoles,18-20 and synthetic analogues thereof. However, there are very few reports where the heterocyclic moiety is substituted with a carbazole unit and hence the synthesis of such compounds is desirable.21,22
On the other hand, compounds containing methanone linker between heterocyclic rings and benzofuran at the C-2 position had been investigated and reported to display important biological properties as antimicrobial,23 anticonvulsant, anti-inflammatory activities,24 and anti-tumor25 activities. On account of these findings, extensive synthetic efforts have been devoted to the development of more novel and interesting benzofuran-2-yl(heteroaryl)methanone derivatives.26-30
Recently, we have reported on the synthesis of symmetrical 3,6-bis(quinolin-2-yl)-9-ethyl-9H-carbazole31 as well as 1,1'-bis(quinolin-2-yl)ferrocenes32 via a Friedländer condensation reaction. Thus, in light of the above findings and in the context of our ongoing studies on combinatorial chemistry and in diversifying our work on the synthesis of more novel heterocyclic compounds, we wish to report herein the synthesis of a series of symmetrical (9-methyl-9H-carbazole-3,6-diyl)bis(benzofuran-2-yl-methanone)s.
Scheme 1 is outlined the synthetic route developed in our laboratory for the one-pot synthesis of (9-methyl-9H-carbazole-3,6-diyl)bis(benzofuran-2-yl-methanone)s (3a-f) through the Rap-Stoermer reaction of 3,6-dichloroacetyl-carbazole (1) with substituted salicylaldehydes. A mixture of the substrate 1, substituted salicylaldehyde, 15 mol% PEG-400, and potassium carbonate was sonicated in acetonitrile within 4 hours at 70 ºC. After completion of the reaction (monitored by TLC) and work up, the products were isolated in good yields of 52-79% by silica gel column chromatography. To our knowledge, this is the first application of the Rap-Stoermer reaction in order to access the benzofuran-2-yl(carbazolyl)methanone derivatives.
Our recent work has demonstrated one-pot synthesis reaction of various substituted salicylaldehydes with 2-chloromethyl-33 or 2-bromomethyl-quinoline34 in refluxing MeCN in the presence of KOH as catalyst and K2CO3 as base to afford 2-(1-benzofuran-2-yl)quinolines in good yields. However, we failed to achieve the one-pot synthesis reaction of 3,6-dichloroacetyl-carbazole (1) with salicylaldehydes under the same procedure, and none of the expected products were detected. Thus, in order to synthesize the targeted compounds through a facile and direct methodology, we devised a route that made use of the Rap-Stoermer reaction,35 which appeared to suit us since it could open the way to the direct construction of new compounds containing methanone linker between the carbazole ring and benzofuran at the C-2 position via base-mediated reaction of salicylaldehydes with α-haloketones. Our initial investigation towards the Rap-Stoermer reaction was conducted according to reported methods under solvent-free36 or solvent-free microwave irradiation37 conditions. Unfortunately, following both methods, the Rap-Stoermer reaction in our case could not occur or gave the intractable complex mixtures observed on TLC that we could not separate any desired product in appreciable yield. Our attempts to follow the route as described by Shang et al.38 using 4-dimmmethylaminopyridine (DMAP) as catalyst in water were also frustrated by very low yields together with undefined mixtures. After many trials, we found that when the Rap-Stoermer reaction was carried out in PEG-400 (15 mol%) as additive in the presence of K2CO3 as the base in refluxing MeCN, the desired products could be obtained, but still plagued by low yields of 24-40%. Moreover, we found that we could not further improve the yields by increasing the amount of PEG-400 or the reaction time. In addition, in the case of employing neat PEG-400, the yields were also very low. As a result, attempts to find other alternative approach are still very desirable.
Recently, Palimkar et al.39 have reported a facile ultrasound promoted synthesis of benzo[b]furans derivatives. Accordingly, the versatility of the ultrasound technique prompted us to further experiment with this approach. To our delight, we found that the ultrasonic irradiation did afford a significant amelioration in the preparation of the desired (9-methyl-9H-carbazole-3,6-diyl)bis-(benzofuran-2-ylmethanone)s (3a-f) versus the classical ways. When the Rap-Stoermer reaction was adopted in conjunction with ultrasonic irradiation, an improvement in terms of yields and reaction time could be achieved. In addition, we also observed that if the ultrasonic-assisted Rap-Stoermer reaction was performed in the absence of PEG-400, the desired products could not be obtained in appreciable yields, which indicated that both PEG-400 and ultrasonication together promoted this reaction. Presumably, the reaction may be the phenomenon of cavitation produced by ultrasound,40,41 coupled with the fact that PEG-400 could stabilize the transition state,19 thereby resulting in an increase in the reaction rate. Through an effort to optimize the reaction conditions, we found that the best results were achieved when the ultrasound-assisted reaction was conducted at a temperature of 70 ºC with 15 mol% PEG-400. The use of 15 mol% PEG-400 is sufficient to push this reaction forward and the use of more than the amount resulted in a lower yield, which might be due to the fact that the transition state is solvated in the more amount of PEG-400. In addition, we also attempted to other solvents such as DMF, acetone, CHCl3, acetone and dioxane. But the yields could not be improved further. Some representative results are summarized in Table 1.
The structures of all the newly synthesized compounds 3a-f were elucidated from their spectral (1H and 13C NMR) and analytical data as described for 3a. Its 1H NMR spectrum exhibited no signal attributable to chloroacetyl protons. Particularly characteristic was the presence of two symmetrical furan protons singlet at 7.61 ppm along with the fourteen aromatic protons between 7.35-8.96 ppm, which is consistent with the attachment of the nascent symmetrical two benzofuran ring moieties to the carbazole substrate. The 1H decoupled 13C NMR spectrum was also in good agreement with the assigned structure, which revealed the presence of typical carbonyl carbon at 183.7 along with fourteen distinct resonances due to the carbazole and benzofuran ring carbons. Further, the structure was confirmed by its ESI-MS (positive-ion mode) spectrum, which exhibited a characteristic quasi-molecular ion peak cluster (M+H)+ at m/z 470.1 In addition, the obtained elemental analysis values were in agreement with theoretical values. Similarly, the other synthesized compounds exhibited similar spectral characteristics except the substituents which exhibited characteristic signals with appropriate chemical shifts.
EXPERIMENTAL
The melting points were measured on WRS-1B digital melting points apparatus and are uncorrected. The progress of the reaction was monitored by TLC. Infrared spectra were recorded on KBr pellets on an FT/IR-430 spectrophotometer. 1H NMR and 13C NMR spectra were recorded on a Brucker AVANCE NMR spectrometer using CDCl3 or DMSO-d6 as the solvent. The reported chemical shifts (δ values) are given in parts per million downfield from tetrammethylsilane (TMS) as the internal standard. Elemental analyses were estimated on an Elementar Vario EL-III element analyzer. The Mass spectra were determined using a MSD VL ESI1 spectrometer. The reaction process was monitored by thin-layer chromatography (TLC) on silica gel GF254 using EtOAc/petroleum ether (1/6).
Procedure for the synthesis of 3,6-dichloroacetyl-9-methyl-9H-carbazole (1). A mixture of anhydrous aluminium chloride (100 mmol, 13.3 g) in dry CH2Cl2 (80 mL) was cooled to 0−5 ºC. Chloroacetyl chloride (60 mmol, 6.78 g) dissolved in dry CH2Cl2 (20 mL) was added drop-wise to the mixture with vigorous stirring at such a rate that the temperature was maintained below 5 ºC. Subsequently, a solution of 9-methyl-9H-carbazole (20 mmol, 3.62 g) in dry CH2Cl2 (30 mL) was also added to the mixture under 0−5 ºC. When the addition was complete, the reaction mixture was stirred at 0−5 oC for 48 h. The reaction mixture was then poured into cooled water and extracted with CH2Cl2. The combined organic layer was washed with 5% aqueous NaHCO3 (3×30 mL) and subsequently with water (3×30 mL) and dried over Na2SO4. After CH2Cl2 was removed in vacuo, the crude product was purified by silica gel column chromatography with EtOAc/petroleum ether (1:1) as eluent to give the pure yellow product in 66% yield, mp 209−210 ºC. IR (KBr) ν/cm-1: 3058, 2931, 2830, 1669, 1588, 1543, 1441, 1249, 1019; 1H NMR (600 MHz, CDCl3): δ (ppm): 3.98 (s, 3H), 4.86 (s, 4H), 7.51 (d, J = 8.4 Hz, 2H), 8.18 (dd, J = 8.4, 1.2 Hz, 2H), 8.77 (d, J = 1.2 Hz, 2H); 13C NMR (150 MHz, CDCl3): 29.77, 37.94, 110.76, 119.46, 123.58, 123.87, 127.91, 144.28, 189.76. Anal. Calcd for C17H13Cl2NO2: C, 61.10; H, 3.92; N, 4.19. Found: C, 60.91; H, 3.83; N, 4.00.
General procedure for the synthesis of (9-methyl-9H-carbazole-3,6-diyl)bis-(benzofuran-2-yl-methanone)s (3a–f). To a stirred solution of 3,6-dichloroacetyl-9-methyl-9H-carbazole (1) (334 mg, 1 mmol) and substituted salicylaldehyde (2a–e) or 2-hydroxyl-1-naphthaldehyde (2f) (2.2 mmol) in 4 mL of MeCN, was added K2CO3 (552 mg, 4 mmol) and PEG-400 (15% mmol). The resulting mixture was sonicated at 70 ºC in 4 h. After the reaction was complete (TLC), the mixture was cooled to room temperature, poured into water and filtered to give the crude product, which was then purified by silica gel column chromatography with EtOAc/petroleum ether (1:6) as eluent to give the pure product 3a–f in 52-79% yields.
(9-Methyl-9H-carbazole-3,6-diyl)bis(benzofuran-2-yl-methanone) (3a). This compound was obtained as yellow crystals, mp 215–216 ºC; IR (KBr) ν/cm-1: 3071, 2972, 1643, 1591, 1436, 1062, 809, 747; 1H NMR (600 MHz, CDCl3): δ (ppm): 3.99 (s, 3H), 7.35 (t, J = 7.8 Hz, 2H), 7.51 (t, J = 7.8 Hz, 2H), 7.56 (d, J = 8.4 Hz, 2H), 7.61 (s, 2H), 7.68 (d, J = 8.4 Hz, 2H), 7.76 (d, J = 7.8 Hz, 2H), 8.33 (dd, J = 8.4, 1.2 Hz, 2H), 8.96 (d, J = 1.2 Hz, 2H); 13C NMR (150 MHz, CDCl3): 29.70, 108.97, 112.52, 115.88, 122.85, 123.22, 123.37, 123.91, 127.10, 128.04, 128.59, 129.42, 144.44, 152.80, 155.89, 183.66; ESI-MS m/z: 470.1 (M+1)+. Anal. Calcd for C31H19NO4: C, 79.31; H, 4.08; N, 2.98. Found: C, 79.51; H, 3.97; N, 2.77.
(9-Methyl-9H-carbazole-3,6-diyl)bis((5-methylbenzofuran-2-yl)methanone) (3b). This compound was obtained as yellow crystals, mp 211–213 ºC; IR (KBr) ν/cm-1: 3065, 2963, 1640, 1591, 1432, 1022, 816, 775; 1H NMR (600 MHz, CDCl3): δ (ppm): 2.53 (s, 6H), 3.98 (s, 3H), 7.17 (d, J = 7.8 Hz, 2H), 7.47 (s, 2H), 7.54 (d, J = 8.4 Hz, 2H), 7.56 (s, 2H), 7.62 (d, J = 7.8 Hz, 2H), 8.31 (d, J = 7.8 Hz, 2H), 8.95 (s, 2H); 13C NMR (150 MHz, CDCl3): δ (ppm): 15.68, 22.09, 29.73, 108.94, 112.49, 114.87, 116.20, 120.64, 122.67, 122.84, 123.34, 123.69, 124.68, 125.67, 127.07, 128.54, 130.97, 139.03, 144.37, 152.49, 153.81, 156.48, 183.47; ESI-MS m/z: 498.2 (M+1)+. Anal. Calcd for C33H23NO4: C, 79.66; H, 4.66; N, 2.82. Found: C, 79.75; H, 4.57; N, 2.64.
(9-Methyl-9H-carbazole-3,6-diyl)bis((5-(tert-butyl)benzofuran-2-yl)methanone) (3c). This compound was obtained as yellow crystals, mp 125–127 ºC; IR (KBr) ν/cm-1: 3076, 2979, 1640, 1591, 1432, 1023, 816, 776; 1H NMR (600 MHz, CDCl3): δ (ppm): 1.40 (s, 18H), 3.98 (s, 3H), 7.55 (d, J = 8.4 Hz, 2H), 7.57–7.59 (m, 6H), 7.74 (s, 2H), 8.32 (d, J = 8.4 Hz, 2H), 8.94 (s, 2H); 13C NMR (150 MHz, CDCl3): δ (ppm): 29.69, 31.67, 34.83, 108.97, 111.68, 116.30, 119.03, 122.80, 123.37, 126.48, 126.84, 128.54, 129.53, 144.39, 147.10, 152.94, 154.27, 183.74; ESI-MS m/z: 582.1 (M+1)+. Anal. Calcd for C39H35NO4: C, 80.53; H, 6.06; N, 2.41. Found: C, 80.67; H, 6.01; N, 2.05.
(9-Methyl-9H-carbazole-3,6-diyl)bis((5-bromobenzofuran-2-yl)methanone) (3d). This compound was obtained as yellow crystals, mp 245–248 ºC; IR (KBr) ν/cm-1: 3063, 2982, 1642, 1591, 1434, 1022, 814, 772, 622; 1H NMR (600 MHz, CDCl3): δ (ppm): 4.01 (s, 3H), 7.54−7.61 (m, 8H), 7.90 (s, 2H), 8.53 (s, 2H), 8.34 (d, J = 8.4 Hz, 2H); 13C NMR (150 MHz, CDCl3): δ (ppm): 29.77, 45.87, 57.79, 109.11, 114.05, 114.67, 117.01, 122.92, 123.48, 125.68, 128.69, 128.98, 129.17, 130.99, 144.61, 153.81, 154.52, 183.30; ESI-MS m/z: 626.3, 627.9, 629.8 (M+1)+. Anal. Calcd for C31H17Br2NO4: C, 59.36; H, 2.73; N, 2.23. Found: C, 59.41; H, 2.65; N, 2.37.
(9-Methyl-9H-carbazole-3,6-diyl)bis((5-(tert-butyl)-7-fluorobenzofuran-2-yl)methanone) (3e). This compound was obtained as yellow crystals, mp 228−230 ºC; IR (KBr) ν/cm-1: 3065, 2972, 1641, 1592, 1435, 1192, 1024, 815, 778; 1H NMR (600 MHz, CDCl3): δ (ppm): 1.39 (s, 18H), 4.00 (s, 3H), 7.29 (dd, J = 12.6, 1.2 Hz, 2H), 7.51 (d, J = 1.8 Hz, 2H), 7.57 (d, J = 8.4 Hz, 2H), 7.61 (d, J = 2.4 Hz, 2H), 8.37 (dd, J = 8.4, 1.2 Hz, 2H), 8.99 (d, J = 1.2 Hz, 2H); 13C NMR (150 MHz, CDCl3): δ (ppm): 29.74, 31.57, 35.05, 109.14, 112.16, 112.26, 114.48, 115.77, 122.86, 123.60, 128.68, 129.14, 130.02, 141.24, 141.32, 144.56, 146.86, 148.53, 148.76, 153.99, 183.02; ESI-MS m/z: 618.1 (M+1)+. Anal. Calcd for C39H33F2NO4: C, 75.83; H, 5.38; N, 2.27. Found: C, 75.97; H, 5.44; N, 2.11.
(9-Methyl-9H-carbazole-3,6-diyl)bis(naphtho[2,1-b]furan-2-yl-methanone) (3f). This compound was obtained as orange crystals, mp 261–263 ºC. IR (KBr) ν/cm-1: 3071, 2972, 1642, 1590, 1436, 1232, 1065, 808, 765 cm-1; 1H NMR (600 MHz, CDCl3): δ (ppm): 3.97 (s, 3H), 7.51 (d, J = 8.4 Hz, 2H), 7.56 (t, J = 7.8 Hz, 2H), 7.60 (t, J = 7.8 Hz, 2H), 7.73 (d, J = 9.0 Hz, 2H), 7.85 (s, 2H), 8.01 (d, J = 8.4 Hz, 2H), 8.12 (d, J = 9.0 Hz, 2H), 8.17 (d, J = 7.8 Hz, 2H), 8.44 (dd, J = 8.4, 1.2 Hz, 2H), 8.89 (s, 2H); 13C NMR (150 MHz, CDCl3): δ (ppm): 29.77, 109.02, 112.94, 114.88, 123.01, 123.14, 123.64, 123.73, 125.48, 127.41, 128.19, 128.56, 128.94, 129.55, 129.74, 130.65, 143.48, 152.74, 154.47, 183.08; ESI-MS m/z: 570.0 (M+1)+. Anal. Calcd for C39H23NO4: C, 82.24; H, 4.07; N, 2.46. Found: C, 82.48; H, 3.79; N, 2.47.
ACKNOWLEDGEMENTS
The authors would like to thank the financial support from the Foundation of Liaoning Province Key Laboratory of Applied Chemistry (Grant No. 2008s001).
References
1. H.-J. Knölker and K. R. Reddy, Chem. Rev., 2002, 102, 4303. CrossRef
2. M. Itoigawa, Y. Kashiwada, C. Ito, H. Furukawa, Y. Tachibana, K. F. Bastow, and K. H. Lee, J. Nat. Prod., 2000, 63, 893. CrossRef
3. C. Ito, S. Katsuno, M. Itoigawa, N. Ruangrungsi, T. Mukainaka, M. Okuda, Y. Kitagawa, H. Tokuda, H. Nishino, and H. Furukawa, J. Nat. Prod., 2000, 63, 125. CrossRef
4. M. Prudhomme, Eur. J. Med. Chem., 2003, 38, 123. CrossRef
5. L. Hu, Z. Li, Y. Li, J. Qu, Y. Ling, J. Jiang, and D. W. Boykin, J. Med. Chem., 2006, 49, 6273. CrossRef
6. Y. Tachibana, H. Kikuzaki, N. H. Lajis, and N. Nakatani, J. Agric. Food Chem., 2003, 51, 6461. CrossRef
7. M. Chakrabarty, N. Ghosh, and Y. Harigaya, Tetrahedron Lett., 2004, 45, 4955. CrossRef
8. V. Dhayalan, J. A. Clement, R. Jagan, and A. K. Mohanakrishnan, Eur. J. Org. Chem., 2009, 531. CrossRef
9. V. Gaddam and R. Nagarajan, Org. Lett., 2008, 10, 1975. CrossRef
10. J. M. Pedersen, W. R. Bowman, M. R. J. Elsegood, A. J. Fletcher, and P. J. Lovell, J. Org. Chem., 2005, 70, 10615. CrossRef
11. M. S. Mudadu, A. N. Singh, and R. P. Thummel, J. Org. Chem., 2008, 73, 6513. CrossRef
12. M. F. Martínez-Esperón, D. Rodríguez, L. Castedo, and C. Saá, Tetrahedron, 2008, 64, 3674. CrossRef
13. M. L. Bennasar and T. R. F. Ferrando, J. Org. Chem., 2006, 71, 1746. CrossRef
14. I. C. F. R. Ferreira, M. J. R. P. Queiroz, and G. Kirsch, Tetrahedron Lett., 2003, 44, 4327. CrossRef
15. S. C. Pelly, C. J. Parkinson, W. A. L. van Otterlo, and C. B. de Koning, J. Org. Chem., 2005, 70, 10474. CrossRef
16. M. Laronze, M. Boisbrun, S. Léonce, B. Pfeiffer, P. Renard, O. Lozach, L. Meijer, A. Lansiaux, C. Bailly, J. Sapi, and J. Y. Laronze, Bioorg. Med. Chem., 2005, 13, 2263. CrossRef
17. C. Ayats, R. Soley, F. Albericio, and M. Álvarez, Org. Biomol. Chem., 2009, 7, 860. CrossRef
18. T. Janosik, N. Wahlström, and J. Bergman, Tetrahedron, 2008, 64, 9159. CrossRef
19. R. Gu, K. Robeyns, L. Van Meervelt, S. Toppet, and W. Dehaen, Org. Biomol. Chem., 2008, 6, 2484. CrossRef
20. K. M. Meragelman, L. M. West, P. T. Northcote, L. K. Pannell, T. C. McKee, and M. R. Boyd, J. Org. Chem., 2002, 67, 6671. CrossRef
21. R. Meesala and R. Nagarajan, Tetrahedron Lett., 2006, 47, 7557. CrossRef
22. T. K. Chaitanya and R. Nagarajan, Tetrahedron Lett., 2007, 48, 2489. CrossRef
23. S. Alper-Hayta, M. Arisoy, Ö.Temiz-Arpaci, I. Yildiz, E. Aki, S. Özkan, and F. Kaynak, Eur. J. Med. Chem., 2008, 43, 2568. CrossRef
24. K. M. Dawood, H. Abdel-Gawad, E. A. Rageb, M. Ellithey, and H. A. Mohamed, Bioorg. Med. Chem., 2006, 14, 3672. CrossRef
25. I. Hayakawa, R. Shioya, T. Agatsuma, H. Furukawa, S. Naruto, and Y. Sugano, Bioorg. Med. Chem. Lett., 2004, 14, 4383. CrossRef
26. Y. Hu, Y. Zhang, Z. Yang, and R. Fathi, J. Org. Chem., 2002, 67, 2365. CrossRef
27. M. R. Saberi, T. K. Vinh, S. W. Yee, B. J. N. Griffiths, P. J. Evans, and C. Simons, J. Med. Chem., 2006, 49, 1016. CrossRef
28. Y. Liu, J. Qian, S. Lou, and Z. Xu, J. Org. Chem., 2010, 75, 6300. CrossRef
29. O. Saku, M. Saki, M. Kurokawa, K. Ikeda, T. Takizawa, and N. Uesaka, Bioorg. Med. Chem. Lett., 2010, 20, 1090. CrossRef
30. S. Parekh, D. Bhavsar, M. Savant, S. Thakrar, A. Bavishi, M. Parmar, H. Vala, A. Radadiya, N. Pandya, J. Serly, J. Molnár, and A. Shah, Eur. J. Med. Chem., 2011, 46, 1942. CrossRef
31. Y. Li and W. T. Gao, Beilstein J. Org. Chem., 2010, 6, 966. CrossRef
32. W. T. Gao, X. P. Chen, and Y. Li, Heterocycles, 2010, 81, 1923. CrossRef
33. W. T. Gao, J. Liu, Y. Jiang, and Y. Li, Beilstein J. Org. Chem., 2011, 7, 210. CrossRef
34. W. T. Gao, C. H. Zhang, and Y. Li, J. Braz. Chem. Soc., 2010, 21, 806. CrossRef
35. R. Stoermer, Ann. Chem., 1900, 312, 237.
36. K. Yoshizawa, S. Toyota, F. Toda, and I. Csöregh, Green Chem., 2003, 5, 353. CrossRef
37. M. L. N. Rao, D. K. Awasthi, and D. Banerjee, Tetrahedron Lett., 2007, 48, 431. CrossRef
38. Y. J. Shang, C. Wang, X. W. He, K. Ju, M. Zhang, S. Y. Yu, and J. P. Wu, Tetrahedron, 2010, 66, 9629. CrossRef
39. S. S. Palimkar, V. S. More, and K. V. Srinivasan, Ultrason. Sonochem., 2008, 15, 853. CrossRef
40. J. L. Luche, Synthetic Organic Sonochemitry, Plenum Press, New York, 1998.
41. T. J. Mason and D. Peter, Pratical Sonochemistry seconded., Eills Horwood, London, 2002.