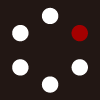
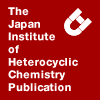
HETEROCYCLES
An International Journal for Reviews and Communications in Heterocyclic ChemistryWeb Edition ISSN: 1881-0942
Published online by The Japan Institute of Heterocyclic Chemistry
e-Journal
Full Text HTML
Received, 13th January, 2012, Accepted, 15th February, 2012, Published online, 20th February, 2012.
DOI: 10.3987/REV-12-728
■ Cyclic Triolborate Salts: Novel Reagent for Organic Synthesis
Yasunori Yamamoto*
Division of Chemical Process Engineering, Graduate School of Engineering, Hokkaido University, Kita 13 Nishi 8, Kita-ku, Sapporo, Hokkaido 060-8628, Japan
Abstract
This review summarizes metal-catalyzed C-C and C-N bond-forming reactions using novel heteroaryltriolborate salts for palladium- or copper-catalyzed cross-coupling reactions with arylhalides or amines and for rhodium-catalyzed addition reactions to α,β-unsaturated carbonyl compounds or imines.CONTENTS
1. Introduction
2. Synthesis of Heteroaryltriolborates
3. Palladium-catalyzed cross-coupling reaction
4. Copper-catalyzed N-arylation
5. Rhodium-catalyzed asymmetric 1,4-addition reaction
6. Rhodium-catalyzed asymmetric addition to imines
7. Conclusion
1. INTRODUCTION
Over the past three decades, it has become increasingly clear that organoboron compounds are valuable reagents capable of undergoing many catalytic C-C bond formations in organic synthesis. Boronic acids are convenient reagents that are generally thermally stable and are inert to water and oxygen, and it is easy to remove the inorganic by-products from the reaction mixture, making the reactions suitable for industrial processes.1 These were followed by discoveries of various bond-forming reactions including a diastereoselective addition of allylboronates to aldehydes,2 Suzuki-Miyaura cross-coupling reactions,3 copper-promoted arylation of N-H, S-H, and O-H bonds,4 and metal-catalyzed addition to electron-deficient alkenes and carbon-heteroatom double bonds.5
The C-B bond of organoboronic acids is totally covalent and inert to ionic reactions, but nucleophilicity of organic groups on a boron atom is significantly enhanced by quaternization by an anionic ligand. Thus, tetracoordinated ate-complexes are a key species that has been successfully used for addition and coupling reactions of organoboron compounds including metal-catalyzed reactions of organoboronic acids. Air- and water-stable trifluoroborates [RBF3]M (M = K and NnBu3) are ate-complexes that are advantageous over boronic acids in preparation and handling of pure and water-stable crystalline materials.6 However, their metal-catalyzed bond-forming reactions are very slow in the absence of bases because of the low nucleophilicity of organic groups due to high electronegativity of fluorine atoms. Thus, sodium trihydroxyborates [RB(OH)3]Na have recently been synthesized as isolated discrete species for cross-coupling in anhydrous solvents.7 There have been attempts to synthesize stable ate-complexes such as ArB(OR)3Li,8 [RC≡CBR’2(OR)]Li,9,10 [Ar4B]Na,11 and (HetAr)2B(OBu)2MgBr12 in order to overcome the limitations of in situ preparation of such ate-complexes from organoboranes and aqueous bases. Recently, we have developed cyclic aryltriolborates [ArB(OCH2)3CCH3]M (M = Li, Na, K, and NBu4) that have high levels of stability in air and water.13,14 High performance for bond-forming reactions was demonstrated in palladium-catalyzed cross-coupling,15 copper-catalyzed N-arylation16 and rhodium-catalyzed asymmetric addition reactions.17 Here, we summarize the chemistry of heteroaryltriolborates, but this review is mainly restricted to our own efforts in metal-catalyzed reactions.
2. SYNTHESIS OF HETEROARYLTRIOLBORATES
Kinetic study on the reaction of substituted arylboronic acids showed that electron-withdrawing substituents accelerate the deboronation (Table 1).18 Although there is no large effect between meta- and para-substituted phenylboronic acids, substituents at the ortho position may greatly increase the rate of deboronation. Even if there is no major steric hindrance, the reaction under aqueous conditions gives undesirable results due to competitive hydrolytic B-C bond cleavage. As shown in Table 1, such cleavage is significantly accelerated by adjacent heteroatoms in the boronic acids. 2-Pyridylboronic acid is a typical boron compound that undergoes very rapid cleavage with water during coupling reaction.19
A recent advance in this field is the use of pinacol ester,20 N-phenyldiethanolamine ester,21 triisopropoxyborate,8a,22 or N-methyliminodiacetic acid (MIDA) boronate.23 Therefore, we developed heteroaryltriolborates for metal-catalyzed organic synthesis.13 It is remarkable that a 2-pyridylboron compound affords a high yield of the coupling product.
We have reported three methods for the synthesis of aryltriolborates.13 Azeotropic removal of water upon treatment of boronic acid with 1,1,1-tris(hydroxymethyl)ethane gave an ester intermediate. It was easily converted into trioborate by treatment with an alkali metal hydroxide or metal hydride (method A). The corresponding lithium salts were synthesized by alkylation of B(OiPr)3 with aryllithiums, followed by ester exchange with triol. By pouring into hexane, aryltriolborates were obtained high yields as air-stable
solids (method B). This protocol afforded high yields of 2-pyridylboronates sensitive to B-C bond cleavage with water. Similarly, 3-Methyl-2-pyridyltriolborate was synthesized by arylation of B(OiPr)3 with aryllithiums followed by ester exchange with triol (Table 2).
Recently, synthesis of pinacol boronic esters has been achieved by palladium-, nickel-, and copper-catalyzed coupling reactions between arylhalides or triflates and pinacolborane or diborons such as B2pin2 (pin = pinacol). More recently, direct borylation of C-H bonds by HBpin or B2pin2 has become a convenient, economical, and environmentally benign process for synthesis of aromatic boron compounds.1 We have reported a simple and practical synthetic method to convert pinacol boronic esters to triolborates (method C).13c
An ORTEP plot of triolborate showed that the molecular structure contains a bicyclo[2,2,2]octane ring that includes a tetrahedral boron atom (Figure 1). The C-B bond (1.60 Å) is slightly longer than that of neutral PhB(OH)2 (1.56 Å) as a result of sp3 hybridization of the boron atom.7,24 Triolborates are bench-stable ate-complexes that can be handled and stored without special precautions. The corresponding hetreroaryltriolborate salts were synthesized in high yields as solids (Table 2).
3. PALLADIUM-CATALYZED CROSS-COUPLING REACTION
Heteroaromatic derivatives are an important class of compounds due to the frequent occurrence of these fragments in natural products, pharmaceuticals, and functional organic materials. Cross-couplings of heteroaromatic rings result in high yields in reactions between typical arylboronic acids and haloheteroarenes, but a reverse combination between heteroarylboronic acids and haloarenes often fails to give biaryls.19 3- and 4-Pyridyl-,25,26 indolyl-,27 and other heteroarylboronic acid derivatives28-33 have recently been made by numerous groups in the development of highly active catalyst systems. We developed
cross-coupling reactions of heteroaryltriolborates with haloarenes for the synthesis of biaryls.13,15 Although the use of heteroarylboronic acids often results in very low yields due to competitive hydrolytic B-C bond cleavage with water under typical conditions using aqueous bases, triolborates possessing a 2-pyridyl, 3-pyridyl and other heteroarenes afforded biaryl in high yields at 50-120 ºC in anhydrous DMF (Tables 3 and 4). There was a strong accelerating effect of copper salts for reactions of 2- and 3-pyridylborate derivatives, whereas 2-thiophenyl derivatives reacted smoothly, resulting in high yields in absence of the copper salts. Although the best ligand for each combination of two aryl rings did not show clear correlation with chemical properties of triolborates or haloarenes for oxidative addition or transmetalation, the best yield was obtained when Ph3P, JohnPhos, dppp, or XantPhos was used for Pd(OAc)2. Under these reaction conditions, biaryls possessing a heteroaryl ring were synthesized in high yields. Bromides and chloride were used at 80 ˚C for substrates possessing an electron-withdrawing group (Table 4, entries 1-5 and 11-14), but electron-rich 4-tolyl and 4-methoxyphenyl derivatives reacted very slowly at 80 ˚C. Thus, iodides were used at 100 ˚C for these two substrates (entries 6 and 7). The reaction took place smoothly when PdCl2dppp was used as a catalyst (entries 8 and 9). 6-Methyl-2-pyridylborate had strong resistance for an unknown reason (entry 10), though unsubstituted 2-pyridylborate smoothly coupled with representative halides.
3-Methyl-2-pyridylboronic acid is a typical example that undergoes very rapid cleavage with water. We further examined the efficiency of copper salts in this coupling reaction. By examination of phosphine-based ligands, BIPHEP and CuCl gave the best results, and cross-coupling reaction of 3-methyl-2-pyridyltriolborate with 1-bromo-2-methoxynaphthalene and 4-bromo-1,3,5-trimethyl-1H-pyrazole gave corresponding biaryls in high yields (entries 15 and 16).
4. COPPER-CATALYZED N-ARYLATION
Copper-mediated N-arylations with arylboron, -lead, -bismuth, -stannane or -silicon compounds4 are practical and straightforward methods that have been employed for various syntheses of those compounds. Among the extensive works in N-arylation, coupling between an N-H, O-H or S-H bond and arylboronic acids, discovered independently by Chan,4a Evans,4b and Lam4c in 1998, was a reaction that attracted much attention because of the very mild conditions, the reaction smoothly taking place typically in air at room temperature. Cross-coupling reaction of pyridine-derived boronic acids has proven to be a great challenge; however, only a few relevant studies can be found in the literature.8,20-23,25 Reaction velocities in coupling of representative boron compounds such as PhB(OH)2, [PhBF3]K and potassium phenyltriolborate with piperidine are shown in Scheme 3. The reaction of potassium phenyltriolborate was 3-times faster than that of PhB(OH)2 because of easier transmetalation of the ate-complex of triolborate than that of neutral ones. On the other hand, the analogous ate-complex of [PhBF3]K resulted in very slow reaction due to its low nucleophilicity and poor solubility in toluene.
Arylboronic acids undergo coupling with water as a side reaction to provide phenol and diaryl ether by-products. Catalytic arylation of the N-H bond of piperidine provided arylamines (9, 11, 13, and 15) in high yields in the presence of Cu(OAc)2 (10 or 20 mol%) under an atmosphere of oxygen (Scheme 4). Potassium 2-pyridyltriolborates (7 and 10) gave the desired product in 70 and 67% yields. Similarly, potassium 3-pyridyltriolborates (12 and 14) were also suitable and formed 13 and 15 in good yields (85 and 83%) (Scheme 4).
The application of this methodology to arylation of imidazoles with potassium aryltriolborates was also explored by using trimethylamine N-oxide as a reoxidant and DMF as a solvent (Table 5). Such coupling of tolyltriolborate with various imidazoles formed arylimidazoles in yields of no less than 83%, except for 2-phenylimidazole, which resulted in 43% yield due to steric hindrance of the phenyl group. Representative arylborates were suitable for arylation of imidazoles in 70-90% yields.
5. RHODIUM-CATALYZED ASYMMETRIC 1,4-ADDITION REACTION
Rhodium complexes have been successfully used for organoboranes, -silicones, or -stannanes, and palladium(II) complexes have been successfully used for arylboron, -silicone and -bismuth compounds.5 Among these extensive studies on asymmetric C–C bond formation, asymmetric 1,4-addition reactions of arylboronic acids catalyzed by rhodium(I) complexes ligated by a BINAP,36 CHIRAPHOS,37 or BIPAM38 have attracted much attention due to their low toxicity, compatibility to a broad range of functional groups and high stability in water and air (Scheme 5). Although excellent yields and selectivities have been achieved by using typical arylboronic acids possessing no heteroatoms, there has been little use of pyridine and thiophene analogues34,35 due to the high coordination ability of heteroatoms to catalysts, slow transmetalation and insertion of electron-deficient heteroaryl rings, and facilitating C–B bond cleavage with water. Triolborates have shown several advantages over their boronic acids or related potassium trifluoroborates including 1) high nucleophilicity of aryl rings attached to the boron atom for smooth transmetalation to metal catalysts and 2) high solubility in organic solvents, allowing the use of water-free solvents for preventing hydrolytic B–C bond cleavage. The reaction resulted in very low yields when arylboronic acids, their pinacol esters or potassium trifluoroborates were used. However, the
corresponding lithium heteroaryltriolborates afforded 1,4-adducts with high enantioselectivities up to 97% in the presence of a rhodium(I) catalyst chelated with a chiral binap ligand (Table 6).
We have demonstrated the efficiency of lithium triolborates for the first 1,4-addition of pyridyl and 2-thienyl groups to α,β-unsaturated carbonyl compounds (Table 7). Lithium 6-methoxy-3-pyridyltriolborate underwent 1,4-addition to five-, six- and seven-membered enones in good yields and with high enantiomeric excess under optimized conditions (entries 1–3). The conditions also worked well for acyclic ketone and ester substrates (entries 4 and 5). An electron-rich 6-methoxy-2-pyridyltriolborate gave a 1,4-addition product. Thus, the presence of a donating methoxy group was effective for increasing the reaction rate by accelerating the insertion of the enone into the C–Rh bond. The presence of a substituent at the position adjacent to the nitrogen will also work to block coordination to the catalyst. The enantioselectivity was very low when a binap or chiraphos catalyst was used (ca. 60% ee), but 93% ee was finally achieved when (R,R)-Me-BIPAM38 was used as the chiral auxiliary in the presence of 10 mol% of powdered KOH (entry 6). Me-BIPAM was also good for an acyclic substrate, 63% yield and 81% ee being achieved (entry 7).
Thienylation of cyclic and acyclic enones with 3-thienylborate proceeded without any difficulties in the presence of a rhodium/(S)-binap catalyst (entries 8-10). The enantioselectivities were in the range of 91 to 95% ee. However, the corresponding reaction of a 2-thienyl analogue failed to give a 1,4-addition product. This effect of heteroatoms might be due to the higher sensitivity of 2-thienylborate than 3-thienyl derivative to water and the lower nucleophilicity of the 2-position than that of the 3-position in the thienyl ring. Indeed, the reaction proceeded smoothly when electron-rich 5-methoxy-2-thienylborate was used for cyclic and acyclic substrates (entries 11 and 12).
The furyl rings are excellent synthons of a CO2H group in various syntheses of carboxylic acids. However, attempts at arylation of enones with heteroarylboronic acids such as 2-furylboronic acids were unsuccessful. The best selectivity for the BINAP catalyst was obtained with 5-methylfurylborate, whereas methoxyfuryl, benzo[b]furyl and an unsubstituted one resulted in lower selectivities. Furthermore, no desired product was obtained by boronic acid, pinacol ester derivative and potassium trifluoroborate.17b
Lithium 5-methyl-2-furanyltriolborate was smoothly added to five-, six- and seven-membered unsaturated ketones in good yields and with excellent enantiomeric excess under the conditions thus optimized (Table 8). High yields and high selectivities were also obtained for acyclic ketone possessing primary and secondary alkyl substituents at the β-carbon. The addition to typical unsaturated esters such as methyl crotonate was very slow, but electron-deficient phenyl crotonate gave 1,4-adduct in 70% yield and 94% ee. Thus, ozonolysis in MeOH smoothly occurred at -78 ˚C to yield corresponding chiral 3-keto-carboxylic acids in high yields without producing a by-product and with no racemization of their stereochemistry.
6. RHODIUM-CATALYZED ASYMMETRIC ADDITION TO IMINES
We tried the addition reaction of 2-furylboronic acid derivatives to N-tosylimines. Again the best selectivity was obtained with lithium 5-methyl-2-furyltriolborate, whereas 2-furylboronic acid or 2-furyltriolborate resulted in lower selectivities than that of 5-methylfurylborate.17c Furthermore, no desired product was obtained with lithium 5-methoxyfuryltriolborate. Finally, 5-methyl-2-furyltriolborate was obtained in 62% yield and 99% ee using 3 mol% Rh(acac)(C2H4)2 / 3.3 mol% (R,R)-N-Me-BIPAM in toluene at 100 ºC for 16 h.
Lithium 5-methyl-2-furyltriolborate was smoothly added to N-tosyl-arylimines in moderate yields and with excellent enantiomeric excess (Table 9). Ozonolysis of 18 in methanol smoothly occurred at -78 ºC to yield the corresponding arylglycine derivatives in 87% yield (Scheme 5).
6. CONCLUSION
Our hetroaryltriolborate salts gave a simple access to heteroaryl compounds via palladium- and copper-catalyzed cross-coupling reaction and rhodium-catalyzed asymmetric addition reaction to unsaturated carbonyl compounds or imines. Since such ate-complexes are key species for various reactions of boron compounds, studies towards their applications to other bond-forming reactions are in progress.
ACKNOWLEDGEMENT
I thank Wako Pure Chemical Industries, Ltd., for technical support in cross-coupling reaction of triolborates. I would like to express my sincere gratitude to Dr. X.-Q. Yu, Dr. G.-Q. Li, Ms. M. Takizawa, Mr. Y. Takahashi, Mr. J. Sugai, Mr. S. Kiyomura, and Mr. T. Shirai. I also thank to Prof. N. Miyaura for helpful discussion.
References
1. (a) N. Miyaura and Y. Yamamoto, 'Comprehensive Organometallic Chemistry III' Vol. 9, ed. by R. H. Crabtree and M. P. Mingos, Elsevier, 2007, pp. 146-244; (b) N. Miyaura, Bull. Chem. Soc. Jpn., 2008, 81, 1535; CrossRef (c) D. G. Hall, 'Boronic Acids: Second, Completely Revised and Enlarged Edition,' Wiley-VCH, Weinheim, 2011.
2. R. W. Hoffmann and H.-J. Zeiss, Angew. Chem., Int. Ed. Engl., 1979, 18, 306; CrossRef For review: (b) J. W. Kennedy and D. G. Hall, 'Boronic acids, ' ed. by D. G. Hall, Wiley-VCH, Weinheim, 2005, pp. 241-277.
3. (a) N. Miyaura, K. Yamada, and A. Suzuki, Tetrahedron Lett., 1979, 36, 3437; CrossRef For reviews: (b) N. Miyaura and A. Suzuki, Chem. Rev., 1995, 95, 2457; (c) N. Miyaura, 'Advances in Meta-Organic Chemistry,' Vol. 6, ed. by L. S. Liebeskind, JAI Press, London, 1998, pp. 187-243; (d) N. Miyaura, Topics in Current Chemistry, 2002, 219, 11; CrossRef (e) A. Suzuki and H. C. Brown, 'Organic Syntheses via Boranes,' Vol. 3, Aldrich Chemical Co., Milwaukee, 2003; (f) N. Miyaura, 'Metal-Catalyzed Cross-Coupling Reactions, Second, Completely Revised and Enlarged Edition,' Vol. 1, ed. by A. de Meijere and F. Diederich, Wiley-VCH, Weinheim, 2004, pp. 41-124; CrossRef (g) A. Suzuki and Y. Yamamoto, Chem. Lett., 2011, 40, 894. CrossRef
4. (a) D. M. T. Chan, K. L. Manaco, R.-P. Wang, and M. P. Winters, Tetrahedron Lett., 1998, 39, 2933; CrossRef (b) D. A. Evans, J. L. Katz, and T. R. West, Tetrahedron Lett., 1998, 39, 2937; CrossRef (c) P. Y. S. Lam, C. G. Clark, S. Saubern, J. Adams, M. P. Winters, D. M. T. Chan, and A. Combs, Tetrahedron Lett., 1998, 39, 2941; CrossRef For reviews: (d) S. V. Ley and A. W. Thomas, Angew. Chem., Int. Ed., 2003, 42, 5400; (e) D. M. T. Chan and P. Y. S. Lam, 'Boronic acids,' ed. by D. G. Hall, Wiley-VCH, Weinheim, 2005, pp. 205-240.
5. (a) M. Sakai, H. Hayashi, and N. Miyaura, Organometallics, 1997, 16, 4229; CrossRef For reviews: (b) K. Fagnou and M. Lautens, Chem. Rev., 2003, 103, 169; (c) T. Hayashi and K. Yamasaki, Chem. Rev., 2003, 103, 2829; CrossRef (d) K. Yoshida and T. Hayashi, 'Modern Rhodium-Catalyzed Organic Reactions,' ed. by P. A. Evans, Wiley-VCH, 2005, pp. 55-78; CrossRef (e) Y. Yamamoto, T. Nishikata, and N. Miyaura, J. Synth. Org. Chem. Jpn. (Engl. Ed.), 2006, 64, 1112; (f) Y. Yamamoto, T. Nishikata, and N. Miyaura, Pure and Appl. Chem., 2008, 80, 807; CrossRef (g) N. Miyaura, Synlett, 2009, 2039; CrossRef (h) G. Berthon-Gelloz and T. Hayashi, 'Boronic acids, Second, Completely Revised and Enlarged Edition,' ed. by D. G. Hall, Wiley-VCH, Weinheim, 2011, pp. 263-313.
6. For reviews: (a) S. Darses and J.-P. Genêt, Eur. J. Org. Chem., 2003, 4313; (b) G. A. Molander and N. Ellis, Acc. Chem. Res., 2007, 40, 275; CrossRef (c) H. A. Stefani, R. Cella, and S. Adriano, Tetrahedron, 2007, 63, 3623; CrossRef (d) S. Darses and J.-P. Genêt, Chem. Rev., 2008, 108, 288; CrossRef (e) G. A. Molander and B. Canturk, Chem. Rev., 2009, 48, 9240; (f) G. A. Molander and L. Jean-Gérard, 'Boronic acids, Second, Completely Revised and Enlarged Edition,' ed. by D. G. Hall, Wiley-VCH, Weinheim, 2011, pp. 507-550.
7. A. N. Camidge, V. H. M. Goddard, H. Gopee, N. L. Harrison, D. L. Hughes, C. J. Schubert, B. M. Sutton, G. L. Watts, and A. J. Witehead, Org. Lett., 2006, 8, 4071. CrossRef
8. (a) K. L. Billingsley and S. L. Buchwald, Angew. Chem. Int. Ed., 2008, 47, 4695; CrossRef (b) R. R. Mullens, Tetrahedron Lett., 2009, 50, 6783. CrossRef
9. (a) J. A. Soderquist, K. Matos, and A. Rane, Tetrahedron Lett., 1995, 36, 2401; CrossRef (b) A. Fürstner and G. Seidel, Tetrahedron, 1995, 51, 11165. CrossRef
10. C. H. Oh and S. H. Jung, Tetrahedron Lett., 2000, 41, 8513. CrossRef
11. (a) P. G. Ciattini, E. Morera, and G. Ortar, Tetrahedron Lett., 1992, 33, 4815; CrossRef (b) N. A. Bumagin and V. V. Bykov, Tetrahedron, 1997, 53, 14437. CrossRef
12. B. A. Haag, C, Sämann, A. Jana, and P. Knochel, Angew. Chem. Int. Ed., 2011, 50, 7290. CrossRef
13. (a) Y. Yamamoto, M. Takizawa, X.-Q. Yu, and N. Miyaura, Angew. Chem. Int. Ed., 2008, 47, 928; CrossRef (b) Y. Yamamoto, J. Sugai, M. Takizawa, and N. Miyaura, Org. Synth., 2011, 88, 79; (c) G.-Q. Li, S. Kiyomura, Y. Yamamoto, and N. Miyaura, Chem. Lett., 2011, 40, 702. CrossRef
14. (a) Y. Yamamoto and N. Miyaura, Wako Junyaku Jiho, 2008, 76, 2; (b) Y. Yamamoto and N. Miyaura, Wako Organic Square, 2009, 27, 2; (c) Y. Yamamoto and N. Miyaura, Organometallic News, 2010, 72.
15. (a) Y. Yamamoto, M. Takizawa, X.-Q. Yu, and N. Miyaura, Heterocycles, 2010, 80, 359; CrossRef (b) G.-Q. Li, Y. Yamamoto, and N. Miyaura, Synlett, 2011, 1769; CrossRef (c) G.-Q. Li, Y. Yamamoto, and N. Miyaura, Tetrahedron, 2011, 67, 6804. CrossRef
16. X.-Q. Yu, Y. Yamamoto, and N. Miyaura, Chem. Asian J., 2008, 3, 1517. CrossRef
17. (a) X.-Q. Yu, Y. Yamamoto, and N. Miyaura, Synlett, 2009, 994; CrossRef (b) X.-Q. Yu, T. Shirai, Y. Yamamoto, and N. Miyaura, Chem. Asian J., 2011, 6, 932; CrossRef (c) Y. Yamamoto, Y. Takahashi, K. Kurihara, and N. Miyaura, Aust. J. Chem., 2011, 64, 1447. CrossRef
18. (a) H. G. Kuivila, J. F. Reuwer, Jr., and J. A. Mangravite, Can. J. Chem., 1963, 41, 3081; CrossRef (b) H. G. Kuivila, J. F. Reuwer, Jr., and J. A. Mangravite, J. Am. Chem. Soc., 1964, 86, 2666; CrossRef (c) R. D. Brown, A. S. Buchanan, and A. A. Humffray, Aust. J. Chem., 1965, 18, 1521; CrossRef (d) F. C. Fischer and E. Havinga, Recl. Trav. Chim. Pays-Bas, 1974, 93, 21; CrossRef (e) B. P. Roques, D. Florentin, and M. Callanquin, J. Heterocycl. Chem., 1975, 12, 195; CrossRef (f) D. Florentin, M. C. Fournié-Zaluski, M. Callanquin, and B. P. Roques, J. Heterocycl. Chem., 1976, 13, 1265; CrossRef (g) S. Gronowitz, V. Bobšik, and K. Lawitz, Chemica Scripta., 1984, 23, 120; (h) T. Watanabe, N. Miyaura, and A. Suzuki, Synlett, 1992, 207. CrossRef
19. (a) E. Tyrrell and P. Brookes, Synthesis, 2003, 469; CrossRef (b) L.-C. Campeau and K. Fagnou, Chem. Soc. Rev., 2007, 36, 1058. CrossRef
20. (a) A. Bouillon, J.-C. Lancelot, J. Sopkova de Oliveira Santos, V. Collot, P. R. Bovy, and S. Rault, Tetrahedron, 2003, 59, 10043; CrossRef (b) I. A. I. Mkhalid, D. N. Coventry, D. Albesa-Jove, A. S. Batsanov, J. A. K. Howard, R. N. Perutz, and T. B. Marder, Angew. Chem. Int. Ed., 2006, 45, 489; CrossRef (c) J. Z. Deng, D. V. Paone, A. T. Ginnetti, H. Kurihara, S. D. Dreher, S. A. Weissman, S. R. Stauffer, and C. S. Burgey, Org. Lett., 2009, 11, 345; CrossRef (d) D. X. Yang, S. L. Colletti, K. Wu, M. Song, G. Y. Li, and H. C. Shen, Org. Lett., 2009, 11, 381. CrossRef
21. (a) P. B. Hodgson and F. H. Salingue, Tetrahedron Lett., 2004, 45, 685; CrossRef (b) P. Gros, A. Doudouh, Y. Fort, Tetrahedron Lett., 2004, 45, 6239; CrossRef (c) C. Gütz and A. Lützen, Synthesis, 2010, 85. CrossRef
22. (a) B. T. O’Neill, D. Yohannes, M. W. Bundesmann, and E. P. Arnold, Org. Lett., 2000, 2, 4201; CrossRef (b) L. Ackermann and H. K. Potukuchi, Synlett, 2009, 2852. CrossRef
23. (a) D. M. Knapp, E. P. Gillis, and M. D. Burke, J. Am. Chem. Soc., 2009, 131, 6961; CrossRef (b) G. R. Dick, D. M. Knapp, E. P. Gillis, and M. D. Burke, Org. Lett., 2010, 12, 2314. CrossRef
24. (a) S. J. Rettig and J. Trotter, Can. J. Chem., 1977, 55, 3071; (b) M. K. Cyrański, A. Jezierska, P. Klimentowska, J. J. Panek, and A. Sporzyński, J. Phys. Org. Chem., 2008, 21, 472. CrossRef
25. (a) A. Bouillon, J.-C. Lancelot, V. Collot, P. R. Bovy, and S. Rault, Tetrahedron, 2002, 58, 2885; CrossRef (b) A. Bouillon, J.-C. Lancelot, V. Collot, P. R. Bovy, and S. Rault, Tetrahedron, 2002, 58, 3323; CrossRef (c) A. Bouillon, J.-C. Lancelot, V. Collot, P. R. Bovy, and S. Rault, Tetrahedron, 2002, 58, 4369; CrossRef (d) H. Matondo, S. Souirti, and M. Baboulène, Synth. Commun., 2003, 33, 795; CrossRef (e) C. L. Cioffi, W. T. Spencer, J. J. Richards, and R. J. Herr, J. Org. Chem., 2004, 69, 2210; CrossRef (f) K. L. Billingsley, K. W. Anderson, and S. L. Buchwald, Angew. Chem. Int. Ed., 2006, 45, 3484; CrossRef (g) N. Kudo, M. Perseghini, and G. C. Fu, Angew. Chem. Int. Ed., 2006, 45, 1282; CrossRef (h) R. Kuwano and J.-Y. Yu, Heterocyles, 2007, 74, 233; CrossRef (i) X.-L. Fu, L.-L. Wu, H.-Y. Fu, H. Chen, and R.-X. Li, Eur. J. Org. Chem., 2009, 2051; CrossRef (j) K. M. Clapham, A. S. Batsanov, M. R. Bryce, and B. Tarbit, Org. Biomol. Chem., 2009, 7, 2155. CrossRef
26. (a) M. Terashima, H. Kakimi, M. Ishikura, and K. Kamata, Chem. Pharm. Bull., 1983, 31, 4573; CrossRef (b) M. Ishikura, M. Kamada, and M. Terashima, Heterocycles, 1984, 22, 265; CrossRef (c) M. Ishikura, M. Kameda, and M. Terashima, Synthesis, 1984, 936; CrossRef (d) M. Ishikura, T. Mano, I. Oda, and M. Terashima, Heterocycles, 1984, 22, 2471; CrossRef (e) M. Ishikura, M. Kameda, T. Ohta, and M. Terashima, Heterocycles, 1984, 22, 2475; CrossRef (f) M. Ishikura, T. Ohta, and M. Terashima, Chem. Pharm. Bull., 1985, 33, 4755; CrossRef (g) M. Ishikura, I. Oda. and M. Terashima, Heterocycles, 1985, 23, 2375; CrossRef (h) M. Ishikura, I. Oda, and M. Terashima, Heterocyles, 1987, 26, 1603. CrossRef
27. (a) E. Vazquez, I. W. Davies, and J. F. Payack, J. Org. Chem., 2002, 67, 7551; CrossRef (b) N. Pagano, J. Maksimoska, H. Bregman, D. S. Williams, R. D. Webster, F. Xue, and E. Meggers, Org. Biomol. Chem., 2007, 5, 1218; CrossRef (c) T. Kinzel, Y. Zhang, and S. L. Buchwald, J. Am. Chem. Soc., 2010, 132, 14073; CrossRef (d) M. Ishikura, Heterocycles, 2011, 83, 247. CrossRef
28. K. Billingsley and S. L. Buchwald, J. Am. Chem. Soc., 2007, 129, 3358. CrossRef
29. C. A. Fleckenstein and H. Plenio, J. Org. Chem., 2008, 73, 3236. CrossRef
30. (a) N. Primas, A. Bouillon, J.-C. Lancelot, H. El-Kashef, and S. Rault, Tetrahedron, 2009, 65, 5739; CrossRef (b) N. Primas, A. Bouillon, J.-C. Lancelot, and S. Rault, Tetrahedron, 2009, 65, 6348; CrossRef (c) A. Thakur, K. Zhang, and J. Louie, Chem. Commun., 2012, 48, 203. CrossRef
31. (a) G. A. Molander and B. Biolatto, J. Org. Chem., 2003, 68, 4302; CrossRef (b) G. A. Molander, B. Canturk, and L. E. Kennedy, J. Org. Chem., 2009, 74, 973. CrossRef
32. K. M. Clapham, A. S. Batsanov, R. D. R. Geenwood, M. R. Bryee, A. E. Smith, and B. Tarbit, J. Org. Chem., 2008, 73, 2176. CrossRef
33. N. Henry, C. Enguehard-Gueiffier, I. Thery, and A. Gueiffier, Eur. J. Org. Chem., 2008, 4824. CrossRef
34. A. C. Spivey, L. Shukla, and J. F. Hayler, Org. Lett., 2007, 9, 891. CrossRef
35. (a) M. Pucheault, S. Darses, and J.-P. Genêt, Eur. J. Org. Chem., 2002, 3552; CrossRef (b) K. Yoshida and T. Hayashi, Heterocycles, 2003, 59, 605. CrossRef
36. Y. Takaya, M. Ogasawara, T. Hayashi, M. Sakai, and N. Miyaura, J. Am. Chem. Soc., 1998, 120, 5579. CrossRef
37. T. Itoh, T. Mase, T. Nishikata, T. Iyama, H. Tachikawa, Y. Kobayashi, Y. Yamamoto, and N. Miyaura, Tetrahedron, 2006, 62, 9610. CrossRef
38. (a) Y. Yamamoto, K. Kurihara, N. Sugishita, K. Ohshita, D. Piao, and N. Miyaura, Chem. Lett., 2005, 34, 1224; CrossRef (b) K. Kurihara, N. Sugishita, K. Ohshita, D.-G. Piao, Y. Yamamoto, and N. Miyaura, J. Organomet. Chem., 2007, 692, 428. CrossRef