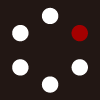
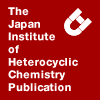
HETEROCYCLES
An International Journal for Reviews and Communications in Heterocyclic ChemistryWeb Edition ISSN: 1881-0942
Published online by The Japan Institute of Heterocyclic Chemistry
e-Journal
Full Text HTML
Received, 18th January, 2012, Accepted, 20th February, 2012, Published online, 28th February, 2012.
DOI: 10.3987/COM-12-12430
■ Synthesis and Crystal Structure of Novel Chiral N-Dichloroacetyl-2-substituted-5-methyl-1,3-oxazolidines
Shuang Gao, Ying Fu, Li-Xia Zhao, Zhi-Yong Xing, and Fei Ye*
Department of Applied Chemistry, College of Sciences, Northwest Agricultural University, Harbin 150030, Heilongjiang, China
Abstract
Chiral 1-amino-2-propanols 4-5 were prepared by racemic 1-amino-2-propanol and chiral tartaric acid. Then, chiral N-dichloroacetyl-2-substituted-5-methyl-1,3-oxazolidines 6-11 were synthesized by the reaction of chiral 1-amino-2-propanol with ketone and dichloroacetyl chloride. The structures of the compounds were characterized by IR, 1H NMR, 13C NMR, MS and elemental analysis. The configuration of 7 was determined by X-ray crystallography.A variety of substituted oxazolidines were reported to possess wide range of biological activities, such as antifungal, insecticidal, antiviral, herbicidal and growth accelerator for plants.1 N-dichloroacetyl oxazolidine compounds have been investigated for potential use as herbicide safeners that can be protecting maize against the injury of thiocarbamate and chloroacetanilide herbicides effectively.2 Examples of enantioselectivity in biological activity were recently recognized among synthetic agrochemicals, these findings attracted our attention towards the synthesis of chiral N-dichloroacetyl oxazolidines.
Optically active amino alcohols were increasingly used as starting materials for the synthesis of oxazolidine derivatives. Accordingly, many synthetic methods including optical resolution of their racemic mixtures, reduction of α-amino acids, ring opening of epoxides by amines had been reported.3 All these methods of chiral resolution were simple and effective. General methods for preparation of chiral oxazolidines involved the use of expensive catalysts.4 Page reported the synthetic method for a number of chiral oxazolidines from ketones and amino alcohols by using microwave irradiation and scandium triflate as catalyst.5 Lee reported that 1,3-oxazolidines were synthesized in good yields by copper-catalyzed addition of ethyl diazoacetate to imines in the presence of acetone.6 Banphavichit obtained N-2-hydroxybenzyloxazolidines by multicomponents Mannich-type reaction of phenols, paraformaldehyde and β-aminoalcohols in the presence of LiCl.7 In addition, Raadt used cyclopentanone as a model compound to reacte with a range of enantiomerically pure amino alcohols for the synthesis of chiral spirooxazolidines with good yields.8 Transition metal catalyzing aminohydroxylation of alkenes with oxaziridines was demonstrated to provide stereoselective access to oxazolidines.9 However, all these methods suffered from some drawbacks, especially in the use of expensive reagents or catalysts. Also, these methods involved multistep operations which lower the overall yields. In continuation of our interest in the synthesis of N-dichloroacetyl oxazolidines,10 we reported herein the synthesis of a series of novel chiral N-dichloroacetyl-2-substituted-5-methyl-1,3-oxazolidines 6-11 via a sequential procedure involving cyclization and acylation without any expensive reagent or catalyst, the synthesis of the title compounds have been accomplished in one pot with high yield.
The chiral amino alcohol (R)-(-)-1-amino-2-propanol 4 was prepared by racemic 1-amino-2-propanol 1 and D-tartaric acid in 45% yield, and (S)-(+)-1-amino-2-propanol 5 was obtained in 42% yield when D-tartaric acid was replaced by L-tartaric acid (Scheme 1).
In order to obtain crystals with moderate yields and high optical purity, the crystallization temperature was optimized at 10-20 °C. Crystallization time also influenced the yields. According to the results, the best crystallization time to obtain (R)-(D)-2 and (S)-(L)-3 crystals was 24 hours.
Chiral N-dichloroacetyl-2-substituted-5-methyl-1,3-oxazolidines 6-11 were synthesized by cyclization of (R)-(-)-1-amino-2-propanol 4 or (S)-(-)-1-amino-2-propanol 5 with ketones, then acylation was followed with dichloroacetyl chloride (Scheme 2). The structures of compounds were listed in Table 1.
The intermediates 4 condensed with ketones to afford chiral 1,3-oxazolidines without any catalyst. Low reaction temperature, -5-0 °C, was employed because this reaction was exothermic. In order to improve the yield of chiral N-dichloroacetyl-5-methyl-1,3-oxazolidines, sodium hydroxide solution was chosen as the attaching acid agent, which reacted with by-product HCl. The compounds 6-8 were gave 59-66% yield, respectively. By the same procedure, 5 was transformed to 9-11 in 56-68% yield, respectively.
The structures of the compounds 6-11 had been confirmed by elemental analyses and spectral data. The IR spectrum of compounds 6-11 showed bands at 1670―1680 cm-1 due to the presence of C=O. The 1H NMR spectra of 6-11 exhibited a single signal in the range δ 6.02―6.96 ppm for proton of Cl2HC-CO-. In the 13C NMR spectra, the signals were observed in the region of δ 159.55―159.68 ppm for the carbon of C=O, at δ 96.41―98.13 ppm for the carbon of O-C-N, at δ 66.97―67.83 ppm for the carbon of CHCl2. Compound 6 and 9, 7 and 10, 8 and 11 were also confirmed by mass spectra which showed the molecular ion peak at m/z 227, m/z 267 and m/z 241, respectively. The single crystal of 7 was obtained by dissolving it in the solvent of ethanol followed by slow evaporation. The structure of 7 was confirmed by X-ray crystallographic analysis and the molecular structure was shown in Figure 1 and Figure 2. Data collection was carried out using a Rigaku R-AXIS RAPID imaging plate diffractometer with graphite-monochromated Mo Ka radiation (λ = 0.71073 Å) at 293(2) K. The structure was solved by direct methods and refined by full-matrix least-squares methods on F2 using the SHELXS-97 crystallographic software package.11
The X-ray structure analysis indicated that compound 7 contained one chiral center at C7 with the R configuration. It was obviously that there was p-π conjunctive effect between O2, N1 and C10, which resulted in shorter bond length of C10-N1 [1.336(3) Å] than the typical C-N bond length [1.472 Å]. The infinite chains were connected by Van der Waals forces, which stabled the crystal structure. Selected bond lengths and angles for 7 were given in Table 2. It was clear that the molecular configuration of 7 was in good agreement with related structures.
In conclusion, we provided a simple, practical and inexpensive method for the synthesis of series of novel chiral N-dichloroacetyl-2-substituted-5-methyl-1,3-oxazolidines derivatives from ketones and chiral 1-amino-2-propanols. The advantages of this method were the readily available starting materials and the mild reaction conditions with high diastereoselectivity products.
EXPERIMENTAL
Melting points were determined on Beijng Taike melting point apparatus (X-4) and uncorrected. The Infrared (IR) spectra were recorded in KBr on a KJ-IN-27G infrared spectrophotometer. The 1H NMR and 13C NMR spectra were recorded on a Bruker AVANCE 300 MHz nuclear magnetic resonance spectrometer at 300 MHz and 75 MHz or a Bruker BioSpin GmbH 400 MHz nuclear magnetic resonance spectrometer at 400 MHz and 100 MHz, respectively, with CDCl3 or DMSO-d6 as the solvent and TMS as the internal standard. Mass spectra were recorded on a Waters Xevo TQ mass spectrometer. Elemental analyses were taken on a FLASH EA1112 Elemental Analysis Instrument. All reagents were of analytical grade.
General procedure for the preparation of (R)-(-)-4 and (S)-(+)-5.12
D-Tartaric acid or L-tartaric acid (30.0 g, 0.2 mol) was dissolved in 40 mL of water, racemic 1-amino-2-propanol (30.0 g, 0.4 mol) was added dropwise under stirring in ice bath. The diastereomeric salt (R)-(D)-2 or (S)-(L)-3 were crystallized when the solutions were kept at room temperature for 24 h. The mixture was filtered and the crystals were washed by ice-cold EtOH and then dried. Afterward, (R)-(D)-2 and (S)-(L)-3 (20 g) were dissolved in a small amount of distilled water and added Ca(OH)2 to pH 10.6 with stirring. The mixture was filtered and the solvent was evaporated under reduce pressure to obtain chiral intermediate (R)-(-)-4 or (S)-(+)-5, respectively.
Typical procedure for the preparation of compound 6-11.
A mixture of chiral amino alcohol (R)-4 (5.0 g, 0.067 mol), acetone (0.067 mol), and benzene (20 mL) was stirred at 33-34 °C for 1 h. After the reaction was finished, the mixture was followed by cooling to 0 °C and addition of 33% aqueous sodium hydroxide solution (10 mL). Then dichloroacetyl chloride (11.2 g, 0.076 mol) was added dropwise with stirring and cooling in an ice bath. The reaction mixture was stirred for 3 h. The organic phase was rinsed with water until pH 7. The organic layer was dried over anhydrous magnesium sulfate and the solvent was removed by distillation under the normal pressure. The crude products of N-dichloroacetyl-2-substituted-5-methyl-1,3-oxazolidines 6-8 were recrystallized with EtOAc and light petroleum. By the same procedure, (S)-(+)-5 was transformed to 9-11.
(R)-1-Amino-2-propanol (4). Yield 45%. Colorless oil. [α]D20 (c 1, H2O) -15.8°; IR (KBr, cm-1) ν: 3276 (O-H), 3319 (N-H), 2974 (C-H); 1H NMR (400 MHz, CDCl3) δ: 3.60-3.67 (m, 1H, -CH-O), 2.66-2.70 (m, 1H, -OH), 2.34-2.48 (m, 4H, -CH2-NH2), 1.07-1.08 (d, J = 6.4 Hz, 3H, -CH3); Anal. Calcd for C3H9NO: C 47.97, H 12.08, N 18.65. Found: C 48.08, H 12.19, N 18.50.
(S)-1-Amino-2-propanol (5). Yield 42%. Colorless oil. [α]D20 (c 1, H2O) +17.5°; IR (KBr, cm-1) ν: 3271 (O-H), 3319 (N-H), 2974 (C-H); 1H NMR (400 MHz, CDCl3) δ: 3.68-3.72 (m, 1H, -CH-O), 2.72-2.76 (m, 1H, -OH), 2.50-2.55 (m, 4H, -CH2-NH2), 1.13-1.15 (d, J = 6.4 Hz, 3H, -CH3); Anal. Calcd for C3H9NO: C 47.97, H 12.08, N 18.65. Found: C 47.90, H 12.20, N 18.57.
(R)-N-Dichloroacetyl-2,2,5-trimethyl-1,3-oxazolidine (6). Yield 59%. White crystals. mp 88-89 °C. [α]D20 (c 2, CHCl3) -5.2°; IR (KBr, cm-1) ν: 3006 (C-H), 1670 (C=O), 1448 (Cl2HC-CO-), 1149 (N-C-O); 1H NMR (300 MHz, CDCl3) δ: 6.03 (s, 1H, Cl2CH-), 4.25-4.32 (m, 1H, -N-CH2-C), 3.96-4.01 (dd, J = 5.2, 9.4 Hz, 1H, -N-CH2-C), 3.24-3.30 (t, J = 9.6 Hz, 1H, -C-CH-O-), 1.65 (s, 3H, CH3-C-), 1.58 (s, 3H, -C-CH3), 1.36-1.38 (d, J = 6.0 Hz, 3H, -C-CH3); 13C NMR (75 MHz, CDCl3) δ: 159.57, 96.41, 70.72, 66.97, 52.21, 25.51, 23.13, 17.76. MS: m/z 227.1 (M+ and base peak). Anal. Calcd for C8H13Cl2NO2: C 42.50, H 5.80, N 6.19. Found: C 42.74, H 5.89, N 6.17.
(R)-N-Dichloroacetyl-2-methyl-1-oxa-4-aza-spiro-4.5-decane (7). Yield 62%. White crystals. mp 164-165 °C. [α]D20 (c 2, CHCl3) -15.6°; IR (KBr, cm-1) ν: 3050 (C-H), 1680 (C=O), 1440 (Cl2HC-CO-), 1100 (N-C-O); 1H NMR (300 MHz, CDCl3) δ: 6.02 (s, 1H, Cl2CH-), 4.19-4.26 (m, 1H, -N-CH2-C), 3.92-3.97 (dd, J = 5.3, 9.3 Hz, 1H, -N-CH2-C), 3.21-3.27 (t, J = 9.5 Hz, 1H, -C-CH-O-), 1.51-2.64 (m, 10H, -(CH2)5-), 1.36-1.38 (d, J = 6.0 Hz, 3H, -C-CH3); 13C NMR (75 MHz, CDCl3) δ: 159.68, 98.13, 70.39, 67.23, 52.27, 33.56, 30.09, 24.54, 23.08, 22.99, 17.88. MS: m/z 267.1 (M+ and base peak). Anal. Calcd for C11H17Cl2NO2: C, 49.64; H, 6.44; N, 5.26. Found: C, 49.74; H, 6.48; N, 5.22.
(5R)-N-Dichloroacetyl-2,5-dimethyl-2-ethyl-1,3-oxazolidine (8). Yield 66%. White crystals. mp 118-119 °C. [α]D20 (c 2, CHCl3) -10.5°; IR (KBr, cm-1) ν: 2966 (C-H), 1674 (C=O), 1423 (Cl2HC-CO-), 1151 (N-C-O); 1H NMR (400 MHz, DMSO-d6) δ: 6.96 (s, 1H, Cl2CH-), 4.23-4.28 (m, 1H, -N-CH2-C), 4.00-4.04 (dd, J = 5.2, 9.6 Hz, 1H, -N-CH2-C), 3.01-3.06 (t, J = 9.8 Hz, 1H, -C-CH-O-), 1.61-2.22 (m, 2H, C-CH2-C-), 1.45 (s, 3H, CH3-C-), 1.25-1.26 (d, J = 5.6 Hz, 3H, -C-CH3), 0.69-0.73 (t, J = 7.4 Hz, 3H, -C-CH3); 13C NMR (100 MHz, DMSO-d6) δ: 159.55, 97.60, 70.48, 67.82, 53.31, 29.62, 22.95, 17.74, 7.31. MS: m/z 241.1 (M+ and base peak). Anal. Calcd for C9H15Cl2NO2: C 45.02, H 6.30, N 5.83. Found: C 45.09, H 6.32, N 5.88.
(S)-N-Dichloroacetyl-2,2,5-trimethyl-1,3-oxazolidine (9). Yield 56%. White crystals. mp 86-87 °C. [α]D20 (c 2, CHCl3) +6.7°; IR (KBr, cm-1) ν: 3005 (C-H), 1670 (C=O), 1450 (Cl2HC-CO-), 1149 (N-C-O); 1H NMR (300 MHz, CDCl3) δ: 6.02 (s, 1H, Cl2CH-), 4.24-4.31 (m, 1H, -N-CH2-C), 3.95-4.00 (dd, J = 5.3, 9.4 Hz, 1H, -N-CH2-C), 3.23-3.30 (t, J = 9.6 Hz, 1H, -C-CH-O-), 1.64 (s, 3H, CH3-C-), 1.57 (s, 3H, -C-CH3), 1.35-1.37 (d, J = 6.0 Hz, 3H, -C-CH3); 13C NMR (75 MHz, CDCl3) δ: 159.56, 96.41, 70.72, 66.97, 52.21, 25.51, 23.13, 17.76. MS: m/z 227.1 (M+ and base peak). Anal. Calcd for C8H13Cl2NO2: C 42.50, H 5.80, N 6.19. Found: C 42.71, H 5.78, N 6.07.
(S)-N-Dichloroacetyl-2-methyl-1-oxa-4-aza-spiro-4.5-decane (10). Yield 65%. White crystals. mp 165-166 °C. [α]D20 (c 2, CHCl3) +14.7°; IR (KBr, cm-1) ν: 3050 (C-H), 1680 (C=O), 1450 (Cl2HC-CO-), 1050 (N-C-O); 1H NMR (300 MHz, CDCl3) δ: 6.02 (s, 1H, Cl2CH-), 4.18-4.26 (m, 1H, -N-CH2-C), 3.92-3.97 (dd, J = 5.3, 9.2 Hz, 1H, -N-CH2-C), 3.21-3.27 (t, J = 9.5 Hz, 1H, -C-CH-O-), 1.48-2.63 (m, 10H, -(CH2)5-), 1.35-1.37 (d, J = 6.0 Hz, 3H, -C-CH3); 13C NMR (75 MHz, CDCl3) δ: 159.68, 98.11, 70.39, 67.23, 52.27, 33.56, 30.09, 24.54, 23.08, 22.99, 17.88. MS: m/z 267.1 (M+ and base peak). Anal. Calcd for C11H17Cl2NO2: C 49.80, H 6.46, N 5.28. Found: C 50.04, H 6.50, N 5.39.
(5S)-N-Dichloroacetyl-2,5-dimethyl-2-ethyl-1,3-oxazolidine (11). Yield 68%. White crystals. mp 115-116 °C. [α]D20 (c 2, CHCl3) +12.5°; IR (KBr, cm-1) ν: 3030 (C-H), 1674 (C=O), 1423 (Cl2HC-CO-), 1151 (N-C-O); 1H NMR (400 MHz, DMSO-d6) δ: 6.90 (s, 1H, Cl2CH-), 4.15-4.24 (m, 1H, -N-CH2-C), 3.94-3.98 (dd, J = 5.6, 9.6 Hz, 1H, -N-CH2-C), 2.95-3.00 (t, J = 9.8 Hz, 1H, -C-CH-O-), 1.56-2.16 (m, 2H, C-CH2-C-), 1.38 (s, 3H, CH3-C-), 1.19-1.20 (d, J = 5.6 Hz, 3H, -C-CH3), 0.63-0.67 (t, J = 7.4 Hz, 3H, -C-CH3); 13C NMR (100 MHz, DMSO-d6) δ: 159.55, 97.60, 70.48, 67.83, 53.31, 29.63, 22.96, 17.75, 7.31. MS: m/z 241.1 (M+ and base peak). Anal. Calcd for C9H15Cl2NO2: C 45.02, H 6.30, N: 5.83. Found: C 45.05, H 6.27, N 5.89.
SUPPLEMENTARY MATERIAL
Crystallographic data for the structural analysis of 7 has been deposited with the Cambridge Crystallographic Data Centre (CCDC 857744). Copies may be obtained free of charge on application to CCDC, 12 Union Road, Cambridge CB2 1EZ, UK; by quoting the publication citation and the deposit numbers. [Fax: (+44) 1223-336-033; E-mail: deposit@ccdc.cam.ac.uk, http://www.ccdc.cam.ac.uk]
ACKNOWLEDGEMENTS
This work was supported by the National Nature Science Foundation of China (No. 31101473), the China Postdoctoral Science Foundation funded project (2011M500634), the Research Science Foundation in Technology Innovation of Harbin (2010RFQYN108), the Science and Technology Research Project of Heilongjiang Education Department and The Postdoctoral Start Foundation of Heilongjiang.
References
1. (a) Q. Y. Ren, W. Y. Mo, L. Gao, H. W. He, and Y. C. Gu, J. Heterocycl. Chem., 2010, 47, 171; (b) A. G. Korepin, P. V. Galkin, E. K. Perepelkina, N. M. Glushakova, and I. L. Eremenko, Russ. Chem. Bull., 2009, 58, 2103; CrossRef (c) A. Zarghi, H. Arefi, O. G. Dadrass, and S. Torabi, Med. Chem. Res., 2010, 19, 782; CrossRef (d) J. M. Wang, F. D. Rochon, Y. Yang, L. Hua, and M. M. Kayser, Tetrahedron: Asymmetry, 2007, 18, 1115. CrossRef
2. K. K. Hatzios and N. Burgos, Weed Sci., 2004, 52, 454. CrossRef
3. (a) N. Yahiro, Chem. Lett., 1982, 11, 1479; CrossRef (b) P. Juszczyk, R. Kasprzykowska, and A. S. Kolodziejczyk, Lett. Pept. Sci., 2003, 10, 79; CrossRef (c) Shivani, B. Pujala, and A. K. Chakraborti, J. Org. Chem., 2007, 72, 3713. CrossRef
4. (a) C. Andres, A. Maestro, R. Pedrosa, A. Perez-Encabo, and M. Vicente, Synlett, 1992, 45; CrossRef (b) D. X. Chen, X. Y. Chen, T. P. Du, L. H. Kong, R. W. Zhen, S. C. Zhen, Y. H. Wen, and G. G. Zhu, Tetrahedron Lett., 2010, 51, 5131; CrossRef (c) S. K. Chung and J. M. Lee, Tetrahedron: Asymmetry, 1999, 10, 1441. CrossRef
5. P. C. B. Page, G. A. Parkes, B. R. Buckley, H. Heaney, M. Gholizadeh, and J. S. Wailes, Tetrahedron Lett., 2008, 49, 6951. CrossRef
6. S. H. Lee, J. Yang, and T. D. Han, Tetrahedron Lett., 2001, 42, 3487. CrossRef
7. V. Banphavichit, W. Bhanthumnavin, and T. Vilaivan, Tetrahedron, 2007, 63, 8727. CrossRef
8. A. de Raadt, B. Fetz, H. Griengl, M. F. Klinger, I. Kopper, B. Krenn, D. F. Münzer, R. O. Ott, P. Plachota, H. J. Weber, G. Braunegg, W. Moer, and R. Saf, Eur. J. Org. Chem., 2000, 3835. CrossRef
9. D. J. Michaelis, C. J. Shaffer, and T. P. Yoon, J. Am. Chem. Soc., 2007, 129, 1866. CrossRef
10. Y. Fu, L. Yang, F. Ye, and S. Gao, Heterocycles, 2011, 83, 2607. CrossRef
11. G. M. Sheldrick, Acta Cryst. A., 2008, 64, 112. CrossRef
12. J. M. Turner, Biochem. J., 1967, 104, 112.