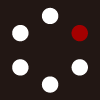
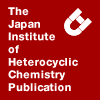
HETEROCYCLES
An International Journal for Reviews and Communications in Heterocyclic ChemistryWeb Edition ISSN: 1881-0942
Published online by The Japan Institute of Heterocyclic Chemistry
e-Journal
Full Text HTML
Received, 4th February, 2012, Accepted, 15th March, 2012, Published online, 16th March, 2012.
DOI: 10.3987/REV-12-733
■ Multicomponent Coupling Reaction of Arynes for Construction of Heterocyclic Skeletons
Hiroto Yoshida* and Ken Takaki
Department of Applied Chemistry, Graduate School of Engineering, Hiroshima University, 1-4-1 Kagamiyama, Higashi-Hiroshima 739-8527, Japan
Abstract
Recent progress on the multicomponent coupling reactions of arynes, directed toward straightforward construction of heterocyclic skeletons, by use of 2-(trimethylsilyl)aryl triflates as aryne precursors is reviewed.CONTENTS
1. Introduction
2. Isocyanide-based multicomponent coupling reaction
3. Imine-based multicomponent coupling reaction
4. Carbonyl compound-based multicomponent coupling reaction
5. Miscellaneous
6. Conclusion
1. INTRODUCTION
Arynes are highly strained and kinetically unstable molecules that have been recognized as useful reactive intermediates in organic synthesis.1 Owing to their low-lying LUMO arising from an efficient mixing of the π* orbital with the σ* orbital,2 arynes exhibit salient electrophilicity,3 and even neutral nucleophiles of diminished nucleophilicity readily add to arynes to produce zwitterions as depicted in Scheme 1. Subsequent capture of the resulting anionic and cationic moieties in the zwitterions by third components (electrophiles) leads to multicomponent coupling reactions of high synthetic significance, however, the reaction modes were rather restricted before utilization of 2-(trimethylsilyl)aryl triflates (1) as aryne precursors, which enables arynes to be generated under neutral conditions.4
As shown in Scheme 2, previous multicomponent coupling reactions were mainly conducted under basic conditions by use of haloarenes as aryne precursors,5,6 which usually limits substrate scope on nucleophiles and electrophiles, and thus those directed toward construction of heterocycles were rare.7
This review aims to summarize the enhanced versatility of the aryne multicomponent coupling reactions with 1, focusing on those involving construction of heterocyclic skeletons, because the multicomponent coupling would be much more attractive from a synthetic standpoint in gaining molecular complexity and diversity. Although a review on the similar topic was reported by Guitián in 2007,8 it chiefly covered intramolecular and two-component reactions, being distinct from the present one.9
2. Isocyanide-based multicomponent coupling reaction
In 2004, we reported that the reaction of arynes (from 1) with isocyanides (nucleophile) and aldehydes (electrophile) provided multicomponent coupling products, iminodihydroisobenzofuran 2, in good yield.10 Variously substituted aldehydes could smoothly take part in the reaction to afford the respective products (Scheme 3), and 1-(trimethylsilyl)-2-naphthyl triflate and 2-(trimethylsilyl)-1-naphthyl triflate, both of which would generate 1,2-naphthalyne, were found to be converted into 2a exclusively, confirming the intermediacy of an aryne in the reaction (Scheme 4).
Regioselectivity in the reaction of unsymmetrical arynes was also investigated: 3-methylbenzyne and 3-methoxybenzyne furnished a single product, whose imino moiety was attached to the sterically less hindered position of the aryne. Similarly, a fluoro substituent at the 4-position of an aryne controlled the regioselectivity to give a product having the imino moiety at the para position of the fluoro substituent, whereas the reaction of 4-methylbenzyne resulted in the formation of almost equal amounts of regioisomeric products (Scheme 5).
Although the yields became somewhat lower than those with aldehydes, such ketones as acetophenones, trifluoroacetophenone and benzil could be coupled with benzyne and t-octyl isocyanide to give the products, and furthermore, treatment of benzoquinones under the reaction conditions offered a spiro type of iminodihydroisobenzofurans in moderate yield (Scheme 6).11 The major isomer in the reaction of 2,6-di-t-Bu-benzoquinone came from cyclization at the less congested carbonyl group.
Direct synthesis of N-heterocycles, iminoisoindolines (3), has been achieved by employing sulfonylimines as electrophiles (Scheme 7).12 Thus, when benzylidenetosylamine was allowed to react with benzyne and t-octyl isocyanide under similar conditions to the above, the multicomponent coupling product was formed in 64% yield. In addition, the reaction was applicable to N-(arylmethylidene)tosylamines bearing an electron-donating or electron-withdrawing substituent, giving modest to good yields of 3.
As shown in Scheme 8, the reaction of 3-methoxybenzyne with a sulfonylimine proceeded with the same regioselectivity as that with an aldehyde, whereas opposite regioselectivity was observed in the reaction of 3-methylbenzyne, despite using the same isocyanide (cf. Scheme 5), which can be attributed to reversibility in the nucleophilic addition of t-octyl isocyanide to 3-methylbenzyne.
A variety of phenyl esters possessing an aliphatic or aromatic substituent were also found to serve as electrophiles in the multicomponent coupling reaction with arynes and isocyanides, affording phenoxy-substituted iminoisobenzofurans (4) (Scheme 9).13 Besides simple phenyl esters, α-chloroester, dihydrocoumarin and diphenyl carbonate were smoothly convertible into 4, which demonstrates the broad substrate scope of the reaction.
Benzo-annulated carbocycles could be readily fabricated depending upon the multicomponent coupling: treatment of electron-deficient alkynes such as methyl propiolate, dimethyl acetylenedicarboxylate and phenylacetylene with arynes and isocyanides led to the efficient formation of iminoindenes (5) (Scheme 10).13
The above isocyanide-based multicomponent coupling reactions would be triggered by nucleophilic addition of an isocyanide to an aryne, as depicted in Scheme 11. Subsequent nucleophilic attack of the resulting zwitterion (6) to an electrophile (carbonyl compound, sulfonylimine and electron-deficient alkyne) gives 7, which then undergoes intramolecular cyclization to provide the product.
The regiochemical outcome in the reaction of unsymmetrical arynes can be rationalized by electronic and steric effects of the substituents (Scheme 12). Since a fluorine moiety in 4-fluorobenzyne exerts a strong electron-withdrawing inductive effect (-I effect), the developing negative charge at the closer position to the fluorine moiety is stabilized markedly in the transition state for the nucleophilic attack, which results in the preferential addition of an isocyanide to the para position of the fluorine moiety. In contrast, a methyl group in 4-methylbenzyne does not induce an electronic difference in the triple bond, leading to the formation of equal amounts of regioisomers. The exclusive introduction of the isocyanide into the less congested site of 3-methoxybenzyne or 1,2-naphthalyne can be attributed to disfavored steric repulsion between the substituent (MeO, or peri-hydrogen) in the aryne and the incoming isocyanide. In addition, the methoxy group in 3-methoxybenzyne exerts -I effect, which also directs the nucleophilic attack toward the meta position of the methoxy moiety. In the case of 3-methylbenzyne, the steric effect would compete with an electron-donating effect (+I effect) of the methyl group, which prefers generation of the aryl anion at the meta position, and thus no consistency in the regioselectivities was observed (Scheme 5 vs Scheme 8).
Although one carbon end of arynes was protonated and zwitterion 6 did not participate in ring construction, the reaction with benzyl isocyanides and terminal alkynes provides a unique way of assembling six-membered N-heterocyclic frameworks.14,15 Thus, when the reaction of arynes, benzyl isocyanides and terminal alkynes (excess) was carried out in toluene/MeCN (4:1), tetrasubstituted pyridines (8) were formed in good yield (Scheme 13). Aryl alkynes having an electron-donating or electron-withdrawing substituent were facilely transformed into the respective pyridines, while the reaction with alkyl alkynes resulted in low yield. As shown in Scheme 14, an initially generated zwitterion (6) is converted into alkynyl imine 9 through proton abstraction (from an alkyne) and carbon–carbon bond formation. Subsequent isomerization of 9 into allenyl imine 10, followed by [4+2] cycloaddition between 10 and a second alkyne produces 8.
Modification in the reaction conditions completely altered the reaction pathway: the use of excess aryne in toluene/MeCN (1:3) led to the selective formation of isoquinolines (11) arising from the [4+2] cycloaddition between 10 and an aryne.14 Similarly to the synthesis of 8, aryl alkynes were readily convertible into 11, and furthermore the reaction could also be applied to ethyl propiolate (Scheme 15).
3. Imine-based multicomponent coupling reaction
A zwitterion (12) arising from nucleophilic addition of imines to arynes was found to be an excellent molecular scaffold for constructing heterocyclic skeletons, and diverse benzoxazinone derivatives (13) could be synthesized straightforwardly by capturing 12 with CO2 (Scheme 16).16 A variety of imines and arynes took part in the multicomponent coupling reaction to give the benzoxazinones of structural diversity (Scheme 17), whereas the reaction with N-t-Bu or N-Ph imine did not give the desired product at all, which indicates that steric congestion or decreased nucleophilicity of the imine nitrogen strictly retards the reaction. Nucleophilic attack of imines to 3-methoxybenzyne or 4-fluorobenzyne occurred like that of isocyanides, and thus the imine nitrogen was preferentially attached to the meta position of MeO or the para position of F.
4. Carbonyl compound-based multicomponent coupling reaction
A carbonyl oxygen of aldehydes also served as the nucleophilic site toward arynes to produce zwitterion 14. In marked contrast to the cases with imines, 14 readily underwent intramolecular cyclization to give benzoxete 15, which then formed ortho-quinone methide 16 via valence isomerization (Scheme 18). Subsequent [4+2] cycloaddition between the exo-dienyl moiety of the resulting ortho-quinone methides and second arynes provided xanthene derivatives (17).17 As depicted in Scheme 19, 2-benzyloxy-1-naphthaldehyde was smoothly coupled with two molar amounts of benzyne to afford 9-(2-bezyloxy-1-naphthyl)xanthene in 70% yield. Other electron-rich aryl aldehydes were also transformable into the respective xanthenes efficiently through formal two-fold insertion of arynes into the carbon–oxygen double bonds, whereas the reaction was retarded by use of electron-deficient aldehydes. Facile formation of 17 in the reaction of electron-rich aldehydes is attributable to enhanced nucleophilicity of the carbonyl oxygen atom toward an aryne, which enables efficient generation of 16.
ortho-Quinone methides 18, arising from the formal [2+2] cycloaddition between arynes and DMF, were found to facilely undergo [4+2] cycloaddition with ester enolates or ketenimine anions to produce diverse coumarins (19) in a straightforward manner (Scheme 20).18 The reaction of active methylene compounds bearing an ester or a phosphoryl group furnished good yields of the coumarins, and furthermore ethyl pyridylacetates and aryl (or heteroaryl) acetonitriles proved to serve efficaciously as third components in the reaction (Scheme 21). In addition, the use of β-diketones or trifluorobutanone has been found to produce 2H-chromenes (20) via the similar [4+2] cycloaddition with ketone enolates (Scheme 22).19
5. Miscellaneous
We have also reported a convenient method for simultaneous introduction of two functionalities (amino and hydroxymehtyl moieties) into 1,2-positions of aromatic rings based on the multicomponent coupling reaction of arynes, aminosilanes and aldehydes.20,21 The use of methyl 2-formylbenzoates as electrophiles in the reaction provided straightforward access to amino-substituted phthalides (21),20 which are formed through trapping of zwitterion 22 with an aldehyde, followed by intramolecular cyclization (Schemes 23 and 24).
Although zwitterions generated from arynes and nucleophiles were not involved as key intermediates, the multicomponent coupling reaction of arynes, six-membered N-heteroaromatics (pyridine and isoquinoline) and α-bromocarbonyl compounds directly provided pyrido[2,1-a]isoindoles (23) through formal [3+2] cycloaddition between arynes and in situ-formed 1,3-dipoles (24) (Schemes 25 and 26).22-24
6. CONCLUSION
Arynes have been demonstrated to serve as efficient relay species between nucleophiles and electrophiles, enabling diverse heterocyclic skeletons to be constructed in a straightforward manner. The success in the aryne multicomponent coupling reactions directed toward synthesis of heterocycles would be attributable to the high functional group compatibility of the method for aryne generation utilizing 2-(trimethylsilyl)aryl triflates, which expands substrate scope on nucleophiles and electrophiles to a great extent. Owing to the simplicity in designing the aryne multicomponent coupling, the concepts would be applicable to construction of other heterocyclic skeletons by combining nucleophiles and electrophiles of different type.
References
1. H. Pellissier and M. Santelli, Tetrahedon, 2003, 59, 701; CrossRef A. M. Dyke, A. J. Hester, and G. C. Lloyd-Jones, Synthesis, 2006, 4093; CrossRef R. Sanz, Org. Prep. Proced. Int., 2008, 40, 215; CrossRef T. Kitamura, Aust. J. Chem., 2010, 63, 987; CrossRef H. Yoshida, J. Ohshita, and A. Kunai, Bull. Chem. Soc. Jpn., 2010, 83, 199. CrossRef
2. N. G. Rondan, L. N. Domelsmith, K. N. Houk, A. T. Bowne, and R. H. Levin, Tetrahedron Lett., 1979, 20, 3237. CrossRef
3. S. V. Kessar, 'Comprehensive Organic Synthesis,' Vol. 4, ed. by B. M. Trost, I. Fleming, Pergamon, Oxford, 1991, pp. 483–515. CrossRef
4. Y. Himeshima, T. Sonoda, and H. Kobayashi, Chem. Lett., 1983, 1211. CrossRef
5. H. Tomori, J. M. Fox, and S. L. Buchwald, J. Org. Chem., 2000, 65, 5334. CrossRef
6. I. Larrosa, M. I. Da Silva, P. M. Gómez, P. Hannen, E. Ko, S. R. Lenger, S. R. Linke, A. J. P. White, D. Wilton, and A. G. M. Barrett, J. Am. Chem. Soc., 2006, 128, 14042. CrossRef
7. Synthesis of N-heterocycles by a multicomponent coupling reaction of arynes using aryl halides as aryne precursors has been reported, see: G. A. Kraus and T. Wu, Tetrahedron, 2010, 66, 569. CrossRef
8. D. Peña, D. Pérez, and E. Guitián, Heterocycles, 2007, 74, 89. CrossRef
9. For other reactions of arynes (pericyclic reactions, intramolecular cyclizations, etc.) for construction of heterocyclic skeletons, see refs 1, 3, and 8.
10. H. Yoshida, H. Fukushima, J. Ohshita, and A. Kunai, Angew. Chem. Int. Ed., 2004, 43, 3935. CrossRef
11. H. Yoshida, H. Fukushima, T. Morishita, J. Ohshita, and A. Kunai, Tetrahedron, 2007, 63, 4793. CrossRef
12. H. Yoshida, H. Fukushima, J. Ohshita, and A. Kunai, Tetrahedron Lett., 2004, 45, 8659. CrossRef
13. K. M. Allan, C. D. Gilmore, and B. M. Stoltz, Angew. Chem. Int. Ed., 2011, 50, 4488. CrossRef
14. F. Sha and X. Huang, Angew. Chem. Int. Ed., 2009, 48, 3458. CrossRef
15. For similar multicomponent coupling reactions which do not include construction of heterocyclic skeletons, see: M. Jeganmohan and C.-H. Cheng, Chem. Commun., 2006, 2454; CrossRef M. Jeganmohan, S. Bhuvaneswari, and C.-H. Cheng, Chem. Asian J., 2010, 5, 153. CrossRef
16. H. Yoshida, H. Fukushima, J. Ohshita, and A. Kunai, J. Am. Chem. Soc., 2006, 128, 11040. CrossRef
17. H. Yoshida, M. Watanabe, H. Fukushima, J. Ohshita, and A. Kunai, Org. Lett., 2004, 6, 4049. CrossRef
18. H. Yoshida, Y. Ito, and J. Ohshita, Chem. Commun., 2011, 47, 8512. CrossRef
19. E. Yoshioka, S. Kohtani, and H. Miyabe, Angew. Chem. Int. Ed., 2011, 50, 6638. CrossRef
20. H. Yoshida, T. Morishita, H. Fukushima, J. Ohshita, and A. Kunai, Org. Lett., 2007, 9, 3367. CrossRef
21. T. Morishita, H. Fukushima, H. Yoshida, J. Ohshita, and A. Kunai, J. Org. Chem., 2008, 73, 5452. CrossRef
22. C. Xie, Y. Zhang, and P. Xu, Synlett, 2008, 3115. CrossRef
23. X. Huang and T. Zhang, Tetrahedron Lett., 2009, 50, 208. CrossRef
24. Other multicomponent coupling reactions of arynes reported by us, see: H. Yoshida, T. Moroshita, and J. Ohshita, Org. Lett., 2008, 10, 3845; CrossRef H. Yoshida, Y. Asatsu, Y. Mimura, Y. Ito, J. Ohshita, and K. Takaki, Angew. Chem. Int. Ed., 2011, 50, 9676 CrossRef