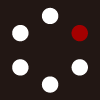
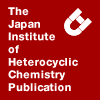
HETEROCYCLES
An International Journal for Reviews and Communications in Heterocyclic ChemistryWeb Edition ISSN: 1881-0942
Published online by The Japan Institute of Heterocyclic Chemistry
e-Journal
Full Text HTML
Received, 24th February, 2012, Accepted, 26th April, 2012, Published online, 8th May, 2012.
DOI: 10.3987/COM-12-S(N)1
■ A Diversified Approach to the Synthesis of Highly Functionalized Novel Azines and Azoles from 2H-Pyran-2-ones
Pushyamitra Mishra, Sandeep Kumar, Hardesh K. Maurya, Sanjay K. Gautam, Brijesh Kumar, Vishnu K. Tandon,* and Vishnu Ji Ram*
Department of Chemistry, University of Lucknow, Lucknow, U.P. 226007, India
Abstract
One-pot regioselective synthesis of 2,6-diarylpyrimidines (4), 2,6-disubstituted pyridines (5 and 6), 5-aryl-3-cyanomethyl-1H-pyrazoles (7) through base catalyzed ring transformation of suitably functionalized 2H-pyran-2-ones by arylamidines, cyanamide, ammonium carbonate, hydrazines respectively has been developed. Our synthetic approach opened a new avenue for the regioselective synthesis of 2,6-diarylpyrimidines and 2,6-disubstituted pyridines and cyanomethylpyrazoles. The procedure is very simple, efficient and economically viable. The protocol provides an efficient methodology for the preparation of new class of medicinally useful and highly functionalized azines and azoles from single precursor 2H-pyran-2-ones.INTRODUCTION
The pyridine and pyrimidine rings are extensively present in the biological system and their role has been greatly realized because of their presence as substructure in enzymes, vitamins, and biopolymers of therapeutic importance, involved in oxidation-reduction processes. The potent biological activity of various vitamins and drugs1-4 is primarily contributed due to the presence of pyridine and pyrimidine moieties in their molecular architecture. Besides various therapeutic applications, pyridines have contributed immensely in the preparative organic chemistry. 4-Dimethylaminopyridine (DMAP), a highly demanding reagent is used as catalyst in acylation reaction and also in activation of carboxylic acids without racemization of α-chiral center.5 The coordinating ability of pyridine and pyrimidine derivatives with various metal ions has made them highly sensitive analytical reagents, sensor systems, luminescent agents and building blocks for supramolecular,6 metallo-gridlike architeture7,8 and in novel inorganic-organic hybrid molecular wires.9 Alkene pendant pyridine polymers are industrially useful as acid scavengers3b and materials for chemical separations. Various functionalized pyrimidines are known to display anticonvulsant,10 anti-inflammatory,11 antibacterial12 and antimycotic13 activities. The wide-ranging applications of various pyridine and pyrimidine derivatives as drugs, catalysts and agrochemicals14 have significantly augmented the potential of both the ring systems to develop an expedient synthesis devoid from the shortfalls of the earlier procedures in terms of generality, multistep sequences and complex work-up.
Numerous protocols for the construction of pyridines and pyrimidines with varying substitution patterns around the ring are delineated in the chemical literature. The commonest approach for the synthesis of pyridines15 is the condensation of 1,5-diketones with ammonia followed by nitric acid oxidation. 2-Acetylfuran has also been used as a 1,5-dicarbonyl equivalent for the construction of congested pyridines and dipyridyls.16 Katritzky et al.17 have reported the synthesis of nicotinonitriles from the reaction of dienamine and ketone using substituted-1,2,3-benzotriazole as a reagent. 2-Amino-4-arylpyridine- 3,5-dinitriles have been prepared18 by base catalyzed reaction of malononitrile with an aldehyde. One of the most versatile approaches for the construction of unsymmetrical pyridines is through the condensation of 1,3-dicarbonyl compounds and 3-aminoenones or nitriles.1 2H-Pyran-2-ones have also been used as a diene equivalent for the preparation19 of congested ethyl nicotinates on reaction with aryl nitriles under Diels-Alder conditions. 2-Aminonicotinonitrile20 has also been synthesized through base catalyzed ring transformation of 6-aryl-4-methylsulfanyl-2H-pyran-2-ones by cyanamide. The reaction of deoxybenzoin, vinamidium species and ammonia is also one of the good methods for the preparation of trisubstituted pyridines as Cox-2 inhibitors.21,22 The synthesis of dihydro- or tetrahydropyridines has been achieved by interactions of 2-azadiene with a suitable dienophile. Using 1,2,4-triazines,23,24 a 2-azadiene equivalent, on reaction with suitable alkyne affords highly functionalized pyridines in excellent yields. A synthetic strategy for the construction of piperidine substituted nicotinic acid has been followed25 through the reaction of enamine with alkynone. Thiopyridines such as 2-amino-3,5-dicyano-6-sulfanylpyridines and corresponding 1,4-dihydropyridines have also been prepared26 from the reaction of an aldehyde, malononitrile and a thiol. Cyclotrimerization of a nitrile and two alkynes in the presence of cobalt (I) catalyst27 is an alternative approach for the construction of highly functionalized pyridines. A regioselective synthesis of 6-amino-5-benzoyl-1-substituted-2-(1H)-pyridones has also been reported recently from the reaction of a cyclic ketene aminal with propiolic acid ester.28,29
The pyrimidine ring, being an electron deficient, resists electrophilic substitution and facilitates nucleophilic addition and substitution reactions.30 A very common approach for the construction of pyrimidine rings is through condensation of 1,3-dicarbonyl compounds with amidines.31 However, 2,4,6-triarylpyrimidines are constructed stepwise.31 The use of formamide or an ortho ester in combination with ammonia32 as a potential surrogate NCN reagent has been reported in the synthesis of pyrimidines. Tris(formylamino)methanes,33 2-amino-2-formylmalonaldehyde34 and 3-methyl-5-nitro-3H-pyrimidin-4- one35 have also been used as 1,3-dicarbonyl equivalents in pyrimidine synthesis. Cyclocondensation of amidine with chalcones is one of the most versatile approach for the construction of pyrimidines36 in good yields.
Herein, we report an expedient, and efficient regioselective synthesis of highly congested 2-(2,6- diarylpyrimidin-4-yl)acetonitriles, 2-amino-4-methylsulfanyl-6-arylnicotinonitrile and 4-methylsulfanyl- 6-arylpyridin-2-amine in moderate yields through ring transformation of suitably functionalized 2H-pyran-2-ones. The methodology is general and flexible to introduce functional groups at appropriate positions by maneuvering the reactants used in the ring transformation reactions.
RESULTS AND DISCUSSION
Our synthetic strategy for the construction of highly congested pyrimidines37 is based on the ring transformation of 6-aryl-4-methylsulfanyl-2H-pyran-2-one-3-carbonitriles (2) by arylamidine using powdered KOH as a base in DMF at 30 °C. The 6-aryl-4-methylsulfanyl-2H-pyran-2-one-3-carbonitriles (2) have been conveniently prepared from the reaction of an aryl methyl ketone with methyl 2-cyano-3,3-dimethylthioacrylate (1) as described earlier37 (Scheme 1).
Thus, a mixture of 6-aryl-4-methylsulfanyl-2H-pyran-2-one-3-carbonitriles (2) and arylamidine (3) was stirred in DMF in the presence of powdered KOH at room temperature for 3-4 h. The progress of the reaction was monitored by TLC. Thereafter, reaction mixture was poured onto crushed ice with vigorous stirring and neutralized with 10% aqueous HCl. The resulting precipitate was filtered, washed with water and dried. The crude product was purified by column chromatography.
The major product isolated in moderate yield was identified as (2,6-diarylpyrimidin-4-yl)acetonitrile. As evident from the topography of 2H-pyran-2-ones (2), that the C-2, C-4, and C-6 positions are electrophilic in nature but position C-6 is highly susceptible to nucleophilic attack due to an extended conjugation and the presence of an electron-withdrawing -CN substituent at position 3 of the pyran ring. The reaction of 2 with arylamidine has possibly been initiated by the attack of the amino group of amidine at C-6 of the pyran ring with concomitant loss of carbon dioxide followed by recyclization with elimination of the methylmercaptan to yield 2, 6-diaryl-(pyrimidin-4-yl)acetonitrile (4) as a major product, Scheme 2.
We have also synthesized some highly congested pyridines using two different protocols. 2-amino-4-(methylsulfanyl)-6-arylnicotinonitrile (5) was prepared by the reaction of
6-aryl-4-methylsulfanyl-2H-pyran-2-one-3-carbonitriles (2) with ammonium carbonate. The reaction is possibly initiated through Michael addition of NH3 at C-6 of the pyran ring with concomitant loss of carbon dioxide followed by recyclization to yield 2-amino-4-(methylsulfanyl)-6-arylnicotinonitrile (5), Scheme 3.
In another attempt 6-aryl-4-methylsulfanyl-2H-pyran-2-one-3-carbonitriles (2) was treated with cyanamide in DMF using powdered KOH as a base. The reaction was carried under nitrogen atmosphere for 48 hours to yield 2-amino-6-aryl-4-methylsulfanylpyridine (6) in good yield, Scheme 4.
We wanted to make pyridones (8) from the reaction of lactone 2 and hydrazine in ethanol at reflux temperature for 4-6 hours but in lieu of anticipated product 8, 5(3)-aryl-3(5)-cyanomethylpyrazoles (7) were isolated in good yields, Scheme 5.
5-Aryl-3-cyanomethyl-1H-pyrazoles (7) and their 1-methyl derivatives are often used as precursors for the synthesis of 3-(2-aminoethyl)-5-phenyl-1H-pyrazoles and their 1-methyl analogs, which are known to have gastric secretion stimulatory activity without producing any pharmacological action towards histamine.38 The reported procedures for the synthesis of these pyrazole derivatives are multistep and cumbersome.39 In search of novel methodology, our attention was drawn to a report40 describing the synthesis of different pyrazoles by reaction of different hydrazines 6-aryl-4-methylsulfanyl-2H-pyran-2-one-3-carbonitriles (2) with hydrazine. 2-Pyranones have three electrophilic centers C-2, C-4 and C-6 in which C-4 and C-6 are more vulnerable to nucleophilic attack. Depending upon the attack of nucleophile either at C-4 or C-6 position, there are possibilities for the formation of two different products. Attack by hydrazine at C-6 leads to the formation of pyrazoles (7) in good yields. The plausible mechanism of the reaction is depicted in Scheme 6.
CONCLUSION
In conclusion, one-pot regioselective synthesis of 2,6-diarylpyrimidines (4), 2,6-disubstituted pyridines (5 and 6) and 5-aryl-3-cyanomethyl-1H-pyrazoles (7) through base catalyzed ring transformation of suitably functionalized 2H-pyran-2-ones by arylamidines, cyanamide, ammonium carbonate and hydrazines respectively have been developed. Our synthetic strategy for the regioselective synthesis of 2,6-diarylpyrimidines and 2,6-disubstituted pyridines and pyrazoles is highly facile. The procedure is extremely simple, efficient and economically viable. The protocol provides a new avenue for the construction of functionalized pyrimidines, pyridines and pyrazoles which can be further exploited for the synthesis of novel heterocycles.
EXPERIMENTAL
Materials and methods: The reagents and the solvents used in this study were of analytical grade and used without further purification. The melting points were determined on an electrically heated Townson Mercer melting point apparatus and are uncorrected. Commercial reagents were used without purification. 1H and 13C NMR spectra were measured on a Bruker WM-300 (300 MHz) using CDCl3 and DMSO-d6 solvents. Chemical shift are reported in parts per million shift (δ-value) from Me4Si (δ 0 ppm for 1H) or based on the middle peak of the solvent (CDCl3) (δ 77.00 ppm for 13C NMR) as the internal standard. Signal patterns are indicated as s, singlet; d, doublet; dd, double doublet; t, triplet; m, multiplet. Coupling constant (J) are given in Hertz. Infrared (IR) spectra were recorded on a Perkin-Elmer AX-1 spectrophotometer in KBr disc and reported in wave number (cm-1). Fast-atomic bombardment (FAB) and ESIMS spectrometers were used for mass spectra analysis. 13C NMR for those compounds which are poorly soluble in DMSO-d6 is not reported.
General procedure for the synthesis of N-(3/4-(6-(cyanomethyl)-2-arylpyrimidin-4-yl)phenyl)- acetamide (4): A mixture of 6-aryl-4-methylsulfanyl-2H-pyran-2-one-3-carbonitriles (2, 1mmol) and arylamidine (3,1mmol) was stirred in DMF in the presence of powdered KOH (1.2 mmol) at room temperature for 3-4 h. The progress of the reaction was monitored by TLC. Thereafter, reaction mixture was poured onto crushed ice with vigorous stirring and neutralized with 10% aqueous HCl. The resulting precipitate was filtered, washed with water and dried. The crude product was purified by column chromatography using CHCl3: MeOH as eluent.
N-(4-(6-(Cyanomethyl)-2-phenylpyrimidin-4-yl)phenyl)acetamide (4a): Yellow solid, IR (KBr) cm-1: 3377 (>N-H), 2922 (-CH2), 2223 (-CN), 1628 (>C=O, NHCO); 1H NMR (300 MHz, DMSO-d6): δ 2.09 (s, 3H, CH3), 3.57 (s, 2H, CH2), 6.82 (s, 1H, Ar-H), 7.14 (s, 3H, Ar-H), 7.72 (m, 3H, Ar-H), 8.00 (m, 3H, Ar-H), 10.32 (s, 1H, NH); ESI-HRMS: m/z calcd for C20H16N4O: 328.1324 (M+); found: 328.1324 (M+).
N-(3-(6-(Cyanomethyl)-2-phenylpyrimidin-4-yl)phenyl)acetamide(4b): Yellow solid, IR (KBr) cm-1: 3323 (>N-H), 3003 (CH2), 2224 (-CN), 1586 (>C=O, NHCO); 1H NMR (300 MHz, DMSO-d6): δ 2.08 (s, 3H, CH3), 3.73 (s, 2H, CH2), 7.18 (s, 2H, Ar-H), 7.51 (m, 2H, Ar-H), 7.78 (m, 2H, Ar-H), 7.87 (m, 2H, Ar-H), 8.12 (m, 2H, Ar-H), 10.19 (s, 1H, NH); ESI-HRMS: m/z calcd for C20H16N4O: 328.1324 (M+); found: 328.1306 (M+).
N-(4-(6-(Cyanomethyl)-2-(pyridin-4-yl)pyrimidin-4-yl)phenyl)acetamide (4c): Brown solid, IR (KBr) cm-1: 3455 (>N-H), 2922 (-CH2), 2199 (-CN), 1660 (>C=O, NHCO); 1H NMR (300 MHz, DMSO-d6): δ 2.06 (s, 3H, CH3), 3.99 (s, 2H, -CH2), 6.83 (s, 1H, Ar-H), 7.79 (d, J = 9 Hz, 2H, Ar-H), 8.01 (d, J = 9 Hz, 2H, Ar-H), 8.15 (d, J = 6 Hz, 2H, Ar-H), 8.35 (d, J = 2 Hz, 2H, Ar-H), 10.24 (s, 1H, NH); ESI-HRMS: m/z calcd for C19H15N5O: 329.1277 (M+); found: 329.1274 (M+).
N-(3-(6-(Cyanomethyl)-2-(pyridin-4-yl)pyrimidin-4-yl)phenyl)acetamide (4d): Light brown solid, IR (KBr) cm-1: 3450 (>N-H), 2930 (-CH2), 2238 (-CN), 1632 (>C=O, NHCO); 1H NMR (300 MHz, DMSO-d6): δ 2.06 (s, 3H, CH3), 3.99 (s, 2H, -CH2), 6.85 (s, 1H, Ar-H), 7.30 (m, 1H, Ar-H), 7.50 (m, 3H, Ar-H), 7.94 (m, 3H, Ar-H), 8.12 (s, 1H, Ar-H), 10.24 (s, 1H, NH); ESI-HRMS: m/z calcd for C19H15N5O: 329.1277 (M+); found: 329.1276 (M+), 330.1312.
General procedure for the synthesis of N-(3/4-(6-amino-4-(methylsulfanyl)pyridin)-2-yl)phenyl)- acetamide (5): A mixture of 6-aryl-4-methylsulfanyl-2H-pyran-2-one-3-carbonitriles (2,1 mmol) and ammonium carbonate (1 mmol) was refluxed in pyridine (10 mL) for 6-8 h. The progress of the reaction was monitored by TLC, The reaction mixture reduced to half by evaporating the excess pyridine. Thereafter it was poured onto crushed ice with vigorous stirring and neutralized with 10% aqueous HCl. The resulting precipitate was filtered, washed with water and dried. The crude product was purified by column chromatography using CHCl3: MeOH as eluent.
N-(4-(6-Amino-4-(methylsulfanyl)pyridin)-2-yl)phenyl)acetamide (5a): Brown solid, IR (KBr) cm-1: 3477, 3446 (>N-H ), 1610 (>C=O, NHCO); 1H NMR (300 MHz, DMSO-d6): δ 2.09 (s, 3H, CH3), 2.83 ( s, 3H, SCH3), 4.83 (s, 2H, NH2), 6.87 (s, 2H, Ar-H), 7.69 (d, J = 9 Hz, 2H, Ar-H), 7.89 (d, J = 9 Hz, 2H, Ar-H), 10.32 (s, 1H, NH); ESI-HRMS: m/z calcd for C14H15N3OS: 273.0936 (M+); found: 273.0966 (M+).
N-(3-(6-Amino-4-(methylsulfanyl)pyridin)-2-yl)phenyl)acetamide (5b): Brown solid, IR (KBr) cm-1: 3575, 3470 (>N-H ), 2322, 1610 (>C=O, NHCO); 1H NMR (300 MHz, DMSO-d6): δ 1.96 (s, 3H, CH3), 2.93 (s, 3H, SCH3), 4.49 (s, 2H, NH2), 6.51 (s, 1H, Ar-H), 7.13 (s, 2H, Ar-H), 7.30 (s, 1H, Ar-H), 7.49 (s, 1H, Ar-H), 7.69 (d, J = 8.1 Hz, 1H, Ar-H), 8.164 (d, J = 8.1 Hz, 1H, Ar-H) 10.014 (s, 1H, NH); ESI-HRMS: m/z calcd for C14H15N3OS: 274.0969 (M++1); found: 274.0968 (M++1).
General procedure for the synthesis of N-(3/4-(6-amino-5-cyano-4-(methylsulfanyl)pyridin)- 2-yl)phenyl)acetamide (6): A mixture of 6-aryl-4-methylsulfanyl-2H-pyran-2-one-3-carbonitriles (2,1 mmol) and cyanamide (1 mmol) was stirred in DMF (8 mL) in the presence of powdered KOH (1.2 mmol) for 48 h under nitrogen atmosphere. The progress of the reaction was monitored by TLC. Thereafter, reaction mixture was poured onto crushed ice with vigorous stirring and neutralized with 10% aqueous HCl. The resulting precipitate was filtered, washed with water and dried. The crude product was purified by column chromatography using CHCl3: MeOH as eluent.
N-(4-(6-Amino-5-cyano-4-(methylsulfanyl)pyridin)-2-yl)phenyl)acetamide (6a): Brown solid, IR (KBr) cm-1: 3477, 3446 (>N-H), 2182 (CN), 1610 (>C=O, NHCO); 1H NMR (300 MHz, DMSO-d6): δ 1.98 (s, 3H, CH3), 2.76 (s, 3H, SCH3), 4.45 (bs, 2H, Ar-NH2), 6.64 (s, 1H, Ar-H), 7.36 (s, 1H, Ar-H), 7.56 (s, 1H, Ar-H), 7.72 (s, 1H, Ar-H), 7.92 (s, 1H, Ar-H), 10.08 (s, 1H, NHCO); 13C NMR (300 MHz, DMSO-d6): δ 14.66, 24.04, 88.34, 100.95, 118.88, 119.21, 127.25 (2C), 134.06, 137.33, 151.32, 158.30 (2C), 161.65, 168.88; ESI-HRMS: m/z calcd for C15H14N4OS: 298.0888 (M+); found: 298.0883 (M+).
N-(3-(6-Amino-5-cyano-4-(methylsulfanyl)pyridin)-2-yl)phenyl)acetamide (6b): Brown solid, IR (KBr) cm-1: 3575, 3470 (>N-H), 1610 (>C=O, NHCO); 1H NMR (300 MHz, DMSO-d6): δ 1.98 (s, 3H, CH3), 2.83 (s, 3H, SCH3), 4.55 (s, 2H, NH2), 6.60 (s, 1H, Ar-H), 7.73 (s, 1H, Ar-H), 7.87 (s, 3H, Ar-H), 10.08 (s, 1H, NHCO); 13C NMR (100 MHz, DMSO-d6): δ 14.66, 24.04, 88.34, 100.95, 118.88, 119.21, 120.22, 124.24, 129.55 135.06, 138.33, 158.30 (2C), 161.65, 168.98; ESI-HRMS: m/z calcd for C15H14N4OS: 298.0888 (M+); found: 298.0883 (M+).
General procedure for the synthesis of N-(3/4-(3-(cyanomethyl)-1H-pyrazol-5-yl)phenyl)acetamide (7): A mixture of 6-aryl-4-methylsulfanyl-2H-pyran-2-one-3-carbonitriles (2,1 mmol) and hydrazine (1 mmol) was refluxed in ethanol (10 mL) for 3-4 h. The progress of the reaction was monitored by TLC. The reaction mixture cooled to room temperature. The precipitate was filtered, washed with alcohol and dried. The crude product was purified by column chromatography using CHCl3: MeOH as eluent.
N-(4-(3-(Cyanomethyl)-1H-pyrazol-5-yl)phenyl)acetamide (7a): Light brown solid, IR (KBr) cm-1: 3377 (>N-H), 1628, 1599 (>C=O, NHCO); 1H NMR (300 MHz, DMSO-d6): δ 1.96 (s, 3H, CH3), 3.88 (s, 2H, CH2), 6.48 (s, 1H, Ar-H), 7.62 (s, 4H, Ar-H), 10.05 (s, 1H, NH), 13.09 (s, 1H, N-H); 13C NMR (100 MHz, DMSO-d6): δ 16.66, 24.04, 100.95, 188.88, 119.21 (2C), 125.65 (2C), 126.25, 139.33, 145.33, 151.34, 168.48; ESI-HRMS: m/z calcd for C13H12N4O: 240.1011 (M+); found: 240.1015 (M+).
N-(3-(3-(Cyanomethyl)-1H-pyrazol-5-yl)phenyl)acetamide (7b): Brown solid, IR (KBr) cm-1: 3323 (>N-H), 1560 (>C=O, NHCO); 1H NMR (300 MHz, DMSO-d6): δ 1.96 (s, 3H, CH3), 3.88 (s, 3H, CH2), 6.38 (s, 1H, Ar-H), 7.69 (s, 4H, Ar-H), 10.05 (s, 1H, NH), 13.09 (s, 1H, N-H); ESI-HRMS: m/z calcd for C13H12N4O: 240.1011 (M+); found: 240.1010 (M+).
N-(4-(3-(Cyanomethyl)-1-(2,4-dinitrophenyl)pyrazol-5-yl)phenyl)acetamide (7c): Brown solid, IR (KBr) cm-1: 3323 (>N-H), 1560 (>C=O, NHCO); 1H NMR (300 MHz, DMSO-d6): δ 1.96 (s, 3H, CH3), 3.88 (s, 3H, CH2), 6.48 (s, 1H, Ar-H), 7.56 (s, 7H, Ar-H), 10.05 (s, 1H, NH); ESI-HRMS: m/z calcd for C19H14N6O5: 406.1026 (M+); found: 406.1022 (M+).
N-(3-(3-(Cyanomethyl)-1-(2,4-dinitrophenyl)pyrazol-5-yl)phenyl)acetamide (7d): Light brown solid, IR (KBr) cm-1: 3323 (>N-H), 1560 (>C=O, NHCO); 1H NMR (300 MHz, DMSO-d6): δ 1.96 (s, 3H, CH3), 3.88 (s, 2H, CH2), 6.48 (s, 1H, Ar-H), 7.56 (s, 7H, Ar-H), 10.05 (s, 1H, NHCO); ESI-HRMS: m/z calcd for C19H14N6O5: 406.1026 (M+); found: 406.1032 (M+).
ACKNOWLEDGEMENTS
Pushyamitra is thankful to CST, UP, India for sponsoring the Project, No. CST/SERPD//D-3433 and Sandeep is thankful to DST, New Delhi for INSPIRE Fellowship [IF10379]. Authors’ are also thankful to CDRI SAIF division, Lucknow, India for providing spectroscopic data.
References
1. J. A. Joule, G. Smith, and K. Mills, Heterocyclic Chemistry, 3rd Ed., Chapman and Hall, London, 1995, pp. 72-119.
2. Pharmaceutical Chemistry, Drug Synthesis, H. J. Roth and A. Kleeman, Eds., Prentice Hall Europe, London 1988, Vol. 1, 407.
3. MDDR: MDL, Drug Data Registry, by MDL informations Systems, Inc: San Leandro, California, U. S. A; G. D. Henry, Tetrahedron, 2004, 60, 6043. CrossRef
4. A.-H. Li, S. Moro, N. Forsyth, N. Melman, X.-D. Ji, and K. A. Jacobsen, J. Med. Chem., 1999, 42, 706; CrossRef B. Vacher, B. Bonnaud, F. Funes, N. Jubaudt, W. Koek, M. B. Assie, C. Cosi, and M. Kleven, J. Med. Chem., 1999, 42, 1648; CrossRef Z. S. Song, M. Zhao, R. Desmond, P. Devine, D. M. Tschaen, R. Tillyer, L. Frey, R. Heid, F. Xu, B. Foster, J. Li, R. Reamer, R. Volante, E. J. Grabowski, U. H. Dolling, and P. J. Reider, J. Org. Chem., 1999, 64, 9658. CrossRef
5. E. F. V. Scriven, Chem. Soc. Rev., 1983, 12, 129; CrossRef U. Ragnarsson and L. Grehen, Acc. Chem. Res., 1998, 31, 494. CrossRef
6. V. N. Kozhevnikov, D. N. Kozhevnikov, T. V. Nikitina, V. L. Rusinov, O. L. Chupakhin, M. Zabel, and B. König, J. Org. Chem., 2003, 68, 2882. CrossRef
7. D. J. Brown, In The Chemistry of Heterocyclic Compounds, Vol. 16, The Pyrimidines, ed. by A. Weissberger; Wiley-Interscience, New York, 1970; J. H. Lister, In The Chemistry of Heterocyclic Compounds, Vol. 24, Fused Pyrimidines, Part II, The Purines, ed. by A. Weissberger and E. C. Taylor, Wiley-Interscience: New York, 1971; M. G. Hoffmann, In Houben-Weyl, Methoden der Organischen Chemie, Vol. E9, E. Schaumann, Ed., G. Thieme Verlag, Stuttgart, 1996; D. T. Hurst, In An Introduction to the Chemistry and Biochemistry of Pyrimidines, Purines and Pteridines, Wiley: Chichester, 1980; J. T. Bojarski, J. L. Mokrosz, H. J. Barton, and M. H. Paluchowska, Adv. Heterocycl. Chem., 1985, 38, 229; CrossRef D. J. Brown, In Comprehensive Heterocyclic Chemistry, A. R. Katritzky and C. W. Rees, Eds., Pergamon Press: Oxford, New York, Toronto, Sydney, Paris, Frankfurt, 1984, Vol. 3, Chapter 2.13.
8. J.-M. Lehn, Supramolecular Chemistry-Concepts and Perspectives, VCH: Weinheim, 1995, Chapter 9; CrossRef G. S. Hanan, D. Volkmer, U. S. Schubert, J.-M. Lehn, G. Baum, and D. Fenske, Angew. Chem., Int. Ed. Engl., 1997, 36, 1842; CrossRef A. Semenov, J. P. Spatz, M. Möller, J.-M. Lehn, B. Sell, D. Schubert, C. H. Weidl, and U. S. Schubert, Angew. Chem. Int. Ed., 1999, 38, 2547. CrossRef
9. A. Harriman and R. Ziessel, Coord. Chem. Rev., 1998, 171, 31; CrossRef A. Harriman and R. Ziessel, Chem. Commun., 1996, 1707. CrossRef
10. N. D. Eddington, D. S. Cox, R. R. Roberts, R. J. Butcher, I. O. Edafiogho, J. P. Stables, N. Cooke, A. M. Goodwin, C. A. Smith, and K. R. Scott, Eur. J. Med. Chem., 2002, 37, 635; CrossRef N. D. Eddington, D. S. Cox, R. R. Roberts, J. P. Stables, C. B. Powell, and K. R. Scott, Curr. Med. Chem., 2000, 7, 417; CrossRef K. R. Scott, G. O. Rankin, J. P. Stables, M. S. Alexander, I. O. Edafiogho, V. A. Farrar, K. R. Kolen, J. A. Moore, L. D. Sims, and A. D. Tonnut, J. Med. Chem., 1995, 38, 4033; CrossRef I. O. Edafiogho, M. S. Alexander, J. A. Moore, V. A. Farrar, and K. R. Scott, Curr. Med. Chem., 1994, 1, 159.
11. G. Dannhardt, A. Bauer, and U. Nowe, Arch. Pharm., 1997, 330, 74. CrossRef
12. V. K. Ahluwalia, N. Kaila, and S. Bala, Indian J. Chem., Sect. B, 1987, 26B, 700.
13. A. Keutzberger and J. Gillessen, Arch. Pharm.,1985, 318, 370. CrossRef
14. G. Matolesy, Pesticidal Chemistry; Elsevier Scientific: Amsterdam Oxford 1988, pp. 427-430; S. A. Lang and Y.-I. Lin, Comprehensive Heterocyclic Chemistry, A. R. Katritzky and C. W. Rees, Eds. Pergmon Press: Oxford 1998, vol. 6, and pp. 1-30.
15. G. Jones, Comprehensive Heterocyclic Chemistry, Eds. A. R. Katritzky and C. W. Rees, Pergmon: New York 1984, vol. 2, pp. 395, Part 2. CrossRef
16. G. R. Pabst and J. Sauer, Tetrahedron Lett., 1998, 39, 6687; CrossRef G. R. Pabst, K. Schmid, and J. Sauer, Tetrahedron Lett., 1998, 39, 6691; CrossRef O. C. Pfuller, and J. Sauer, Tetrahedron Lett., 1998, 39, 8821; CrossRef G. R. Pabst, O. C. Pfuller, and J. Sauer, Tetrahedron Lett., 1998, 39, 8825. CrossRef
17. A. R. Katritzky, A. Denisenko, and M. Arend, J. Org. Chem., 1999, 64, 6076. CrossRef
18. A. S. Alvarez-Insua, M. Lora-Tamayo, and J. L. Soto, J. Heterocycl. Chem., 1970, 7, 1305. CrossRef
19. T. Jaworski and S. Kwiatkowski, Rocz. Chem., 1970, 44, 555.
20. Farhanullah, N. Agarwal, A. Goel, and V. J. Ram, J. Org. Chem., 2003, 68, 2983. CrossRef
21. I. W. Davies, J.-F. Marcoux, E. G. Corley, M. Journet, D.-W. Cai, M. Palucki, J. Wu, R. D. Larsen, K. Rossen, P. J. Pye, L. DiMichele, P. Dormer, and P. J. Reider, J. Org. Chem., 2000, 65, 8415. CrossRef
22. J.-F. Marcoux, E. G. Corley, K. Rossen, P. J. Pye, J. Wu, M. A. Robbins, I. W. Davies, R. D. Larsen, and P. J. Reider, Org. Lett., 2000, 2, 2339. CrossRef
23. S. P. Stanforth, B. Tarbit, and M. D. Watson, Tetrahedron Lett., 2002, 43, 6015. CrossRef
24. S. P. Stanforth, B. Tarbit, and M. D. Watson, Tetrahedron Lett., 2003, 44, 693. CrossRef
25. K. E. Bashford, M. B. Burton, B. S. Cameron, A. L. Cooper, R. D. Hogg, P. D. Kane, D. A. MacManus, C. A. Matrunola, C. J. Moody, A. A. B. Robertson, and M. R. Warne, Tetrahedron Lett., 2003, 44, 1627. CrossRef
26. N. M. Evdokimov, I. V. Magedov, A. S. Kireev, and A. Kornienko, Org. Lett., 2006, 8, 899. CrossRef
27. A. W. Fatland and B. E. Eaton, Org. Lett., 2000, 2, 3131. CrossRef
28. H. Schirok, C. Alonso-Alija, J. Benet-Buchholz, A. H. Göler, R. Grosser, M. Michels, and H. Paulsen, J. Org. Chem., 2005, 70, 9463; CrossRef Z.-T. Huang and Z.-R. Liu, Heterocycles, 1986, 24, 2247; CrossRef R. C. F. Jones and M. J. Smallridge, Tetrahedron Lett., 1988, 29, 5005; CrossRef R. C. F. Jones, P. Patel, S. C. Hirst, and M. J. Smallridge, Tetrahedron, 1988, 54, 6191. CrossRef
29. W. W. Wardhkhan and S. M. Agami, Egypt. J. Chem., 2001, 44, 315..
30. T. Eicher, S. Hauptmann, Chemie der Heterocyclen, Georg Thieme Verlag: Stuttgart, New York, 1994, pp. 398; T. L. Gilchrist, Heterocyclenchemie, Ed. H. Neunhoeffer, Wiley-VCH: New York, 1995, pp. 270.
31. For an efficient repetitive synthesis of (oligo)pyrimidines based upon vinamidinium salt amidine condensations, see: R. Gompper, H.-J. Mair, and K. Polborn, Synthesis, 1997, 696. CrossRef
32. H. Bredereck, R. Gompper, and G. Morlock, Chem. Ber., 1957, 90, 942. CrossRef
33. H. Bredereck, R. Gompper, H. G. Schuh, and G. Theilig, Angew. Chem., 1959, 71, 753. CrossRef
34. P. C. Iuhas, E. Georgescu, F. Georgescu, C. Draghici, and T. M. Caproiu, Rev. Roum. Chim., 2001, 46, 55.
35. N. Nishiwaki, T. Adachi, K. Matsuo, H.-P. Wang, T. Matsunaga, Y. Tohda, and M. Ariga, J. Chem. Soc., Perkin Trans. 1, 2000, 27. CrossRef
36. R. M. Dodson and J. K. Seyler, J. Org. Chem., 1951, 16, 461; CrossRef F. H. Al-Hajjar, and S. S. Sabri, J. Heterocycl. Chem., 1982, 19, 1087; CrossRef D. Simon, O. Lafont, C. C. Farnoux, and M. Miocque, J. Heterocycl. Chem., 1985, 22, 1551; CrossRef D. W. Boykin, A. Kumar, M. Bajic, G. Xiao, W. D. Wilson, B. C. Bender, D. R. McCurdy, J. E. Hall, and R. R. Tidwell, Eur. J. Med. Chem., 1997, 32, 965. CrossRef
37. V. J. Ram, M. Verma. F. A. Hussaini, and A. Shoeb, J. Chem. Res(S), 1991, 98; V. J. Ram, N. Haque, S. K. Singh, F. A. Hussaini, and A. Shoeb, Indian J. Chem., 1993, 32B, 924; V. J. Ram, M. Verma, F. A. Hussaini, and A. Shoeb, Liebigs Ann. Chem., 1991, 1229; CrossRef Y. Tominaga, A. Ushirogochi, Y. Matsuda, and G. Kobayashi, Chem. Pharm. Bull., 1984, 32, 3384. CrossRef
38. R. Pratap, B. Kumar, and V. J. Ram, Tetrahedron, 2007, 63, 10309. CrossRef
39. G. I. Rosiere and M. I. Grossman, Science, 1951, 113, 651. CrossRef
40. R. J. Jones, J. Am. Chem. Soc., 1949, 71, 3994; CrossRef R. J. Jones, M. J. Mann, and K. C. McLaughlin, J. Org. Chem., 1954, 14, 1428. CrossRef