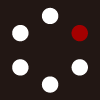
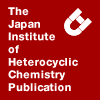
HETEROCYCLES
An International Journal for Reviews and Communications in Heterocyclic ChemistryWeb Edition ISSN: 1881-0942
Published online by The Japan Institute of Heterocyclic Chemistry
e-Journal
Full Text HTML
Received, 29th February, 2012, Accepted, 15th June, 2012, Published online, 21st June, 2012.
DOI: 10.3987/COM-12-S(N)2
■ Highly Enantioselective Synthesis of Isoquinuclidine by Diels-Alder Reaction of 1,2-Dihydropyridine Utilizing Chiral Bisoxazoline-Cu(II) Complex
N. D. M. Romauli Hutabarat, Chigusa Seki,* Takashi Shimizu, Masafumi Hirama, Yoshihito Kohari, Hiroto Nakano, Koji Uwai, Nobuhiro Takano, Eunsang Kwon, and Haruo Matsuyama*
Department of Applied Chemistry, Graduate School of Engineering, Muroran Institute of Technology, 27-1 Mizumoto, Muroran, Hokkaido 050-8585, Japan
Abstract
The enantioselective Diels-Alder (D-A) reaction between N-phenoxycarbonyl- or N-benzyloxycarbonyl-1,2-dihydropyridine (1a or 1b) and N(2)-acryloyl-N(1)-(1-naphthylmethyl)-5,5-dimethylpyrazolidin-3-one (2b) using (S,S)-bisoxazoline-Cu(II) catalyst (A, B, C or D) has been investigated. Utilizing (S,S)-t-Bu-bisoxazoline-Cu(II) catalyst C, the D-A reaction of 1a and 2 afforded the endo-(7S)-isoquinuclidines (3, 4 or 5) in good chemical yields with high enantioselectivity (up to 99% e.e.).INTRODUCTION
Syntheses of natural product-like compounds having isoquinuclidine skeleton, 2-azabicyclo[2.2.2]octane derivatives, via asymmetric synthesis have become one of the most important area of medicinal chemistry.1,2 For example, catharanthine is the prototypical structure of iboga alkaloid which is known to be the precursor of anticancer drug, vinblastine.3 Ibogaine is the medicine for alcohol or other drugs dependence.4 It is also reported that isoquinuclidines are valuable intermediates in the synthesis of oseltamivir phosphate (Tamiflu) (Figure 1).5 The chiral isoquinuclidine can be accomplished by the Diels-Alder (D-A) reaction of 1,2-dihydropyridines with dienophiles. In the asymmetric synthesis of isoquinuclidines, the previous reports have focused on dienophiles having a chiral auxiliary in diastereoselective process.6 In this paper, we describe the utility of (S,S)-bisoxazoline-Cu(II) catalyst7,8 for the enantioselective Diels-Alder reaction of 1,2-dihydropyridine9 1 and achiral N(2)-acryloyl-N(1)-arylmethylsubstituted-5,5-dimethylpyrazolidin-3-one 2 to synthesize the chiral isoquinuclidines in good yields with high enantioselectivity. The R group of pyrazolidinone also can be easily varied and can be effective for providing the face selection in the D-A reaction.10 We used the dienophile which has R group such as 1-benzyl, 1-naphthylmethyl or 2-naphthylmethyl group substituted at N(1) position of pyrazolidinone 2. (S,S)-Bisoxazoline-Cu(II) catalysts (A, B, C and D) were selected because of their successful application of C2-symmetric bisoxazoline-Cu(II) complexes as chiral chelating Lewis acids in numerous asymmetric reactions and also due to the possibility of the controlling selectivity with their structural diversity. The usefulness of bisoxazoline ligand with mild Lewis acid such as Cu(OTf)2 in the D-A reaction has been reported by Evans et al. in the reaction between cyclopentadiene and acrylimide in dichloromethane as a solvent and the D-A reaction afforded endo-cycloadduct with high stereoselectivity (endo/exo = 98:2).7b
RESULTS AND DISCUSSION
We investigated the asymmetric D-A reactions of N-phenoxycarbonyl-1,2-dihydropyridine 1a with the dienophile, N(2)-acryloyl-N(1)-arylmethylsubstituted-5,5-dimethylpyrazolidin-3-one (2a, 2b or 2c)3 at 0 οC in dichloromethane as a solvent in the presence of chiral (S,S)-bisoxazoline-Cu(II) catalyst (A, B, C or D). The results are summarized in Table 1. The (S,S)-bisoxazoline catalysts (A, B and D) were prepared according to the procedure reported by Evans et al.7b The D-A reaction of diene 1a (2 equiv.) and N(2)-acryloyl-N(1)-benzyl-5,5-dimethylpyrazolidin-3-one 2a (1 equiv.) was carried out at 0 οC using 30 mol% of catalyst A (R2= Me) to give the cycloaddition product endo-3 in 89% yield with moderate enantioselectivity (72% e.e., Table 1, entry 1). The 2 equiv. (or 3 equiv.) of 1,2-dihydropyridine 1a (or 1b) was used in the D-A reaction because it is reported that 1,2-dihydropyridines (1a and 1b) were unstable in the presence of Lewis acids.6g The D-A reaction of 1a with N(2)-acryloyl-N(1)- (1-naphthylmethyl)-5,5-dimethylpyrazolidin-3-one 2b using 30 mol% of catalyst A gave endo-4a in 99% yield with 85% e.e. (entry 2). The D-A reaction of 1a with N(2)-acryloyl-N(1)-2-naphthylmethyl- 5,5-dimethylpyrazolidin-3-one 2c using catalyst A afforded endo-5 in 96% yield (67% e.e., entry 3). The result of the D-A reaction of 1a and 2b shows that the stereoselectivity of endo-3, 4a and 5 increased according to the bulkiness of the group R2 of chiral ligand (A, B or C) as follows (Me < i-Pr < t-Bu); Table 1, entry 2, chiral catalyst A (R2= Me) 85% e.e.; entry 5, B (R2= i-Pr) 91% e.e.; entry 8, C (R2= t-Bu) 96% e.e. However, despite our expectations, the D-A reaction of 1a and 2 using benzyl-bisoxazoline D (R2= Bn) did not produce better stereoselectivity of endo-4a (entry 11, D, R2= Bn, 83% e.e.). From these enantioselectivities, we found that chiral ligand C (t-Bu-bisoxazoline) was suitable for this reaction as the catalyst. Among three types of dienophiles (2a, 2b, and 2c), 2b was found to be effective for providing higher enantioselectivity of the D-A cycloadduct.
We investigated the D-A reaction of 1a and 2b by decreasing the amount of catalyst C (R2= t-Bu) from 30 mol% to 20 mol%, 15 mol% or 10 mol% and the results were summarized in Table 2. Because the coordination between the copper and the chiral ligand was sensitive to moisture, the addition of activated molecular sieves 4A may be effective in enhancing the chemical yield and stereoselectivity in the D-A reaction. The D-A reaction of 1a and 2b using 30 mol% of catalyst C in the presence of MS-4A showed higher chemical yield and enantioselectivity of endo-4a (Table 2, entry 1, 91% yield with 98% e.e.) than that in the absence of MS-4A (Table 1, entry 8, 71% yield with 96% e.e.). In the D-A reaction of 1a and 2b using 15 mol% of catalyst C without MS-4A still afforded endo-4a with high streoselectivity but in low yield (Table 2, entry 3, 46% yield with 92% e.e.). In the D-A reaction with MS-4A, the chemical yield and enantioselectivity of endo-4a increased to 78% yield with 98% e.e. (Table 2, entry 3). However, when the amount of catalyst C was reduced to 10 mol% in the presence of MS-4A, the chemical yield and stereoselectivity of endo-4a was only 44% yield with 90% e.e. (entry 4).
In the D-A reaction of 1a and 2b using 15 mol% of Cu(OTf)2 without (S,S)-t-Bu-bisoxazoline, endo-4a was obtained in 87% yield (Table 3, entry 1). From this result, we considered that the complete formation of Cu(II)-complex with (S,S)-t-Bu-bisoxazoline is very important. When the excess amount of chiral ligand (S,S)-t-Bu-bisoxazoline than the amount of Cu(OTf)2 was used in the presence of MS-4A, the enantioselectivity of the D-A adduct endo-4a was increased (Table 3). The D-A reaction of 1a and 2b in the presence of 17 mol% of (S,S)-t-Bu-bisoxazoline and 15 mol% of Cu(OTf)2 gave endo-4a in 76% yield with 99% e.e. (Table 3, entry 2). This result gave slightly higher stereoselectivity than that in the presence of 15 mol% of t-Bu-bisoxazoline and 15 mol% of Cu(OTf)2, which endo-4a was obtained in 78% yield with 98% e.e. (Table 2, entry 3). Furthermore, the reaction in the presence of 18 mol% of t-Bu-bisoxazoline and 15 mol% of Cu(OTf)2 gave endo-4a in better yield (Table 3, entry 3, 82% yield with 99% e.e.). Similarly, in the reaction using 12 mol% of (S,S)-t-Bu-bisoxazoline and 10 mol% of Cu(OTf)2, the enantiomeric excess of endo-4a was increased to 98% e.e. (Table 3, entry 4), though the reaction in the presence of 10 mol% of t-Bu-bisoxazoline and 10 mol% of Cu(OTf)2 gave endo-4a in 44% yield with 90% e.e. (Table 2, entry 4). Next, we examined the effect of counter ion of catalyst in the D-A reaction. In the reaction using 18 mol% of t-Bu-bisoxazoline and 15 mol% of Cu(ClO4)2, endo-4a was obtained in 61% yield with 97% e.e. (Table 3, entry 6). Though the reaction was continued for 48 h, the chemical yield and stereoselectivity of endo-4a was 65% yield with 96% e.e. (Table 3, entry 7).
We also investigated the D-A reaction of N-benzyloxycarbonyl-1,2-dihydropyridine 1b with 2b. In the D-A reaction of 1b (2 equiv.) with N(2)-acryloyl-N(1)-(1-naphthylmethyl)-5,5-dimethylpyrazolidin-3-one 2b (1 equiv.) was carried out in the presence of 30 mol% of catalyst C gave endo-4b in 42% yield with 99% e.e. (Table 4, entry 1). In the D-A reaction of 1b with 2b, the dropwise addition of 1b (2 equiv.) to 2b (1 equiv.) was carried out in the presence of MS-4A using 30 mol% of catalyst C, and endo-4b was obtained in better yield (entry 2, 78% yield with 99% e.e.). We also investigated the D-A reaction by dropwise addition of 1b (3 equiv.) to 2b (1 equiv.) and the endo-4b was obtained in 75% yield with 99% e.e. (entry 3). The D-A reaction of 1b with 2b for 48 h, afforded endo-4b in 73% yield with 99% e.e. (entry 4). Since the presence of a small excess amount of (S,S)-t-Bu-bisoxazoline to Cu salt in the reaction between 1a and 2b improved the chemical yield and stereoselectivity of the cycloadduct endo-4a as shown in Table 3, we have examined the D-A reaction of 1b (3 equiv.) with 2b (1 equiv.) using 36 mol% of (S,S)-t-Bu-bisoxazoline and 30 mol% of Cu(OTf)2, however, the chemical yield with stereoselectivity of endo-4b was 72% yield with 99% e.e. (entry 5).
The absolute stereochemistry of D-A adduct 4a was established to be (1S,4R,7S) by comparing the sign of the optical rotation with the literature value of (7R)-benzyl ester 6, {lit.,3a [α]D -59.92 (c 2.52, DMSO)} as follows: the reaction of the endo-4a (98% e.e.) with benzyl alcohol using n-BuLi as a base (PhCH2OLi) in THF afforded the corresponding (7S)-benzyl ester 6 {[α]D +74.5 (c 0.39, DMSO), 98% e.e.} in 36% yield (Scheme 1).
From the absolute stereochemistry and high enentioselectivity (up to 99% e.e.) of (7S)-4a, we considered the plausible reaction mechanism of the asymmetric D-A reaction of 1a and 2b as shown in Figure 2. It is well established that the complex of (4S,4’S)-bisoxazoline-Cu(II) catalyst react via distorted square planar geometries upon complexation to bidentate substrates.7b Sibi et al. have reported the x-ray structures of pyrazolidinones and possibility of π−π interactions between aromatic relay groups and the olefin of the dienophile.10c
The role of the fluctional N(1) substituent with the bulky 1-naphthylmethyl group of achiral pyrazolidinone template in conjugation with chiral Lew acid, (4S,4’S)-t-Bu-bisoxazoline catalyst C (R2 = t-Bu), provided enantioselectivities up to 99% e.e. Some chiral Lewis acids might induce chirality such that the pyrazolidinone chirality works in concert with the chiral Lewis acid (matched case) and amplify enantioselectivity. In other cases, the induced chirality might display a mismatched, dissonant effect.
In the complex of dienophile 2b and (4S,4’S)-t-Bu-bisoxazoline catalyst C (R2 = t-Bu), the reaction might be through the intermediate 7 that has less steric interaction between N(1)-1-naphthylmethyl group and C-5 methyl hydrogen atoms of dienophile than that of the intermediate 8. Thus, 1,2-dihydropyridine 1a can approach from the re-face to give (7S)-4a (up to 99% e.e.) since the si-face of the N(2)-acryloyl group of dienophile 2b is shielded by the N(1)-1-naphthylmethyl group of dienophile 2b and C4-tert-butyl group of (4S,4’S)-t-Bu-bis(oxazoline) catalyst C.
In conclusion, we have developped the highly enantioselective Diels-Alder reaction of 1,2-dihydropyridine derivatives (1a and 1b) with achiral dienophile N(2)-acryloyl-N(1)-(1-naphthylmethyl)-5,5-dimethylpyrazolidin-3-one 2b using (S,S)-tert-Bu-substituted bisoxazoline-Cu(II) catalyst C, which provides an efficient methodology for obtaining the pharmacologically important chiral isoquinuclidine derivatives in good yield with high enantioselectivity (up to 99% e.e.).
EXPERIMENTAL
General. Melting points were measured using AS ONE ATM-01 melting point apparatus. 1H NMR spectra and 13C NMR spectra were recorded at 270 MHz on JEOL JNM-EX 270 FT NMR SYSTEM and 500 MHz and 125 MHz on a JEOL JNM-ECA. Chemical shifts of 1H NMR and 13C NMR are reported in parts per million downfield from tetramethylsilane (δ) as the internal standard and coupling constants are in hertz (Hz). Specific rotations were recorded at the sodium D line with a polarimeter. Purification of reaction product was carried out by flash chromatography on silica gel 60 (KANTO CHEMICALS, 40-100 µm). The enantiomeric excess (e.e.) of the cycloaddition products was determined by high performance liquid chromatography (HPLC) using the Chiralpak AD-H (25 cm). Bisoxazoline ligands (R2= Me, i-Pr, and Bn) were prepared according to the reported methods.10b (S,S)-t-Butyl-bisoxazoline ligand was purchased from Tokyo Chemical Ind. Co. Commercially available compounds were used without further purification.
1.1 Preparation of 1-(phenoxycarbonyl)-1,2-dihydropyridine (1a)9: The compound 1a has been previously prepared.6b A solution of pyridine (8.00 g, 65 mmol) and NaBH4 (2.06 g, 55 mmol) in 60 mL of absolute EtOH was stirred together at -78 °C and phenyl chloroformate (10.6 g, 65 mmol) was added slowly for l h. The reaction mixture was stirred for 24 h at -78 oC and then quenched with ice-water and the mixture was stirred until the H2 bubble stopped. The product was extracted with Et2O (100 mL x 3). The organic layer was dried over sodium sulfate, filtered, and concentrated. The product 1a was recrystallized from EtOH to give a white solid product in 50% yield (6.60 g, 32.8 mmol). The ratio of 1,2-dihydropyridine and 1,4-dihydropyridine (95:5) was measured by 1H NMR (δ 4.45-4.59 ppm for 1,2-dihydropyridine and δ 2.89 ppm for 1,4-dihydropyridine): white solid; mp 64-66 °C; 1H NMR [ppm] (500 MHz, CDCl3) δ 4.45 (q, J = 4.0, 1.9 Hz, 1.2H), 4.59 (br s, 0.8H), 5.23-5.29 (m, 1H), 5.55-5.61 (m, 1H), 5.89-5.92 (m, 1H), 6.81 (d, J = 7.4 Hz, 0.6 H), 6.88 (d, J = 8.0 Hz, 0.4 H), 7.13 (d, J = 8.0 Hz, 2H), 7.23 (t, J = 7.4 Hz, 1H), 7.38 (t, J = 7.7 Hz, 2H); 13C NMR [ppm] (125 MHz, CDCl3) δ 43.82, 105.85, 119.51, 121.57 (2C), 121.84, 125.37, 125.71, 126.06, 129.40 (2C), 150.92.
1.2 Preparation of 1-(benzyloxycarbonyl)-1,2-dihydropyridine (1b)9: To absolute EtOH (8 mL) solution of pyridine (1.89 g, 24 mmol) and NaBH4 (0.39 g, 10 mmol) was slowly added benzyl chloroformate (3.41 g, 20 mmol for 1a) for 1 h at -78 °C. The reaction mixture was stirred for 24 h at -78 °C and then quenched with ice-water and extract with Et2O. The organic layer was dried over sodium sulfate, filtered and concentrated. The residue was purified by column chromatography on silica gel (eluent, n-hexane/AcOEt = 20:1) to afford 1b as pale yellow oil in 54% yield (2.30 g, 10.0 mmol). The ratio of l,2-dihydropyridine and 1,4-dihydropyridine (99:1) was measured by 1H NMR (δ 4.38 ppm for 1,2-dihydropyridine and δ 2.89 ppm for 1,4-dihydropyridine): oil; 1H NMR [ppm] (500 MHz, CDCl3) δ 4.38 (s, 2H), 5.10 (t, J = 6.3 Hz, 1H), 5.18 (br s, 2H), 5.51-5.53 (m, 1H), 5.80-5.83 (m, 1H), 6.70 (d, J = 8.0 Hz, 0.6H), 6.78 (d, J = 7.4 Hz, 0.4H), 7.32-7.36 (m, 5H); 13C NMR [ppm] (125 MHz, CDCl3) δ 43.6, 43.85, 67.77, 105.02, 119.18, 121.86, 125.52, 128.10, 128.27, 128.47, 128.54,153.00.
1.3 General procedure for the preparation of bisoxazoline ligand10b: Zinc chloride (5 mmol), dimethylmalononitrile (10 mmol) and (S)-2-aminopropanol (22 mmol) {for Me-bisoxazoline ligand (R2 = Me) or L-valinol (22 mol) for i-Pr-bisoxazoline ligand (R2 = i-Pr) or L-alaninol (22 mmol) for Bn-bisoxazoline ligand (R2 = Bn)} in chlorobenzene (20 mL) was heated at reflux for 72 h. The reaction solution was cooled to room temperature and the solvent was evaporated. The residue was dissolved with CH2Cl2 (40 mL) and ammonium hydroxide (5 mL) and then the organic layer was washed with water (5 mL), dried over sodium sulfate, filtered, and concentrated under reduced pressure. The residual oil was purified by column chromatography (eluent, MeOH/AcOEt = 1:9).
1.3.1 2,2-Bis[2-(4S)-methyl-1,3-oxazolinyl]propane: 31% yield; colorless oil, [α]D25 -119.0 (c 1.02, CH2Cl2) (Lit.,7b [α]D25 -67.7 (c 1.0, CH2Cl2); 1H NMR and 13C NMR spectra were identified with the literature values.10b 1H NMR [ppm] (270 MHz, CDCl3) δ 1.25 (d, J = 6.4 Hz, 6H), 1.51 (s,6H), 3.80 (t, J = 7.5 Hz, 2H) , 4.19 (dd, J = 15.7, 6.8 Hz 2H) , 4.36 (t, J = 8.6 Hz, 2H); 13C NMR [ppm] (125 MHz, CDCl3) δ 21.20, 24.26, 38.30, 61.27, 74.35, 168.83.
1.3.2 2,2-Bis[2-(4S)-isopropyl-1,3-oxazolinyl]propane: 30% yield; colorless oil, [α]D25 -126.9 (c 1.02, CH2Cl2) (Lit.,10 [α]D25 -107.5 (c 1.0, CH2Cl2); 1H NMR and 13C NMR spectra were identified with the literature values.11 1H NMR [ppm] (270 MHz, CDC13) δ 0.85 (d, J = 6.7 Hz, 6H), 0.91 (d, J = 6.7, 6H), 1.51 (s, 6H), 1.77-1.84 (m, 2H), 3.92-4.02 (m, 4H), 4.15-4.22 (m, 2H); 13C NMR [ppm] (125 MHz, CDCl3) δ 17.29, 18.50, 24.41, 32.14, 38.52, 69.88, 71.43, 168.74.
1.3.3 2,2-Bis[2-(4S)-benzyl-1,3-oxazolinyl]propane: 24% yield; pale yellow oil, [α]D28 -76.6 (c 0.89, CH2Cl2); 1H NMR and 13C NMR spectra were identified with the literature values.11b 1H NMR [ppm] (500 MHz, CDCl3) δ 1.45 (s, 6H), 2.63-2.68 (dd, J = 13.7, 8.6 Hz, 2H), 3.09 (dd, J = 13.7, 4.5 Hz, 2H), 3.99-4.02 (m, 2H), 4.17 (t, J = 8.8 Hz, 2H), 4.37-4.42 (m, 4H), 7.18-7.30 (m, 10H); 13C NMR [ppm] (125 MHz, CDCl3) δ 24.14, 38.49, 41.26, 66.89, 71.97, 126.43, 128.40, 129.42, 137.61, 169.40.
1.4 General procedure for the preparation of pyrazolidin-3-ones 2a-c3: To a solution of acrylic acid (13 mmol) in THF (50 mL) using a 100 mL flask, triethylamine (25 mmol) was added. The mixture was stirred at -20 °C and acryloyl chloride (12 mmol) was added. The mixture was stirred for 1 h. LiCl (12.5 mmol) and 1-(1-naphylmethyl)-5,5-dimethylpyrazolidin-3-one (10 mmol) 2a, 1-(2-naphthylmethyl)-5,5-dimethylpyrazolidin-3-one (10 mmol) 2b or 1-benzyl-5,5-dimethylpyrazolidin-3-one 2c was added and the mixture was stirred for l0 min, followed by stirring at room temperature for 24 h. The reaction was quenched with 0.2 M HCl aq. and THF was removed under a reduced pressure. The reaction product was extracted with ethyl acetate, and the organic layer was washed with 0.2 M HCl aq., brine, and 1.0 M NaHCO3 aq. The organic layer was dried over Na2SO4, filtrated, and concentrated under reduced pressure. The residual oil was purified by dry silica gel column chromatography (eluent, toluene). n-Hexane was added for crystallization of pyrazolidin-3-one and the solid was filtered to afford 2a, 2b or 2c, respectively.
1.4.1 N(2)-Acryloyl-N(1)-benzyl-5,5-dimethylpyrazolidin-3-one (2a): 33% yield, white solid; mp 81-82 °C (Lit.,10b mp 82-83 oC); 1H NMR and 13C NMR spectra were identified with the literature values.10b 1H NMR (500 MHz, CDCl3) δ 1.29 (s, 6H), 2.57 (s, 2H), 4.07 (s, 2H), 5.67 (d, J = 10.9 Hz, 1H), 6.35 (dd, J =17.2, 1.1 Hz, 1H), 7.07 (dd, J = 16.6, 10.3 Hz, 1H), 7.23-7.30 (m, 3H), 7.38 (d, J =6.9 Hz, 2H); 13C NMR (125 MHz, CDCl3) δ 26.04, 43.48, 56.96, 60.98, 127.62, 128.13, 128.39, 129.59, 130.72, 136.85, 162.97, 174.38.
1.4.2 N(2)-Acryloyl-N(1)-(1-naphthylmethyl)-5,5-dimethylpyrazolidin-3-one (2b): 39% yield, white solid; mp 129-132 °C (Lit.,10b mp 107-109 oC); 1H NMR and 13C NMR spectra were identified with the literature values.10b 1H NMR (500 MHz, CDCl3) δ 1.42 (s, 6H), 2.75 (s, 2H), 4.44 (s, 2H), 4.99 (d, J = 9.1 Hz, 1H), 5.82 (d, J = 17.4 Hz, 1H), 6.32 (m, 1H), 7.34-7.37 (m, 1H), 7.45-7.50 (m, 2H), 7.55-7.58 (m, 1H), 7.77 (d, J = 8.0 Hz, 1H), 7.81 (d, J = 8.0 Hz, 1H), 8.17 (d, J = 8.6 Hz, 1H); 13C NMR (125 MHz, CDCl3) δ 25.90, 42.94, 55.21, 61.45, 123.19, 125.32, 125.59, 126.29, 127.44, 128.72, 129.37, 129.42, 131.49, 132.30, 133.59, 163.43, 173.99.
1.4.3 N(2)-Acryloyl-N(1)-(2-naphthylmethyl)-5,5-dimethylpyrazolidin-3-one (2c): 64% yield, white solid; mp l15-116 °C. IR (Nujol) 1507, 1682, 1743 cm-1. 1H NMR (270 MHz, CDCl3) δ 1.30 (s, 6H), 2.61 (s, 2H), 4.23 (s, 2H), 5.65 (d, J = 10.3 Hz, 1H), 6.29 (d, J = 16.6 Hz, 1H), 7.10-7.15 (m, 1H), 7.43-7.47 (m, 2H), 7.62 (d, J = 8.6 Hz, 1H), 7.78-7.80 (m, 4H); 13C NMR (125 MHz, CDCl3) δ 26.14, 43.54, 57.17, 61.02, 125.91, 126.04, 127.33, 127.62, 127.79, 128.10, 128.17, 128.23, 130.83, 132.78, 133.16, 134.72, 162.91, 174.33. MS m/z 309.15 [M++1]. Anal. Calcd for: C, 74.00; H, 6.54; N, 9.08. Found: C, 74.00; H, 6.60; N, 9.17.
1.5 General procedure for D-A reaction of l,2-dihydropyridine (1a or 1b) with N(2)- acryloyl-N(1)-arylmethyl-5,5-dimethylpyrazolidinone 2a-c using (S,S)-bisoxazoline-Cu(II) (A, B, C, or D): To the appropriate chiral ligand (0.15 mmol) in anhydrous CH2Cl2 (2 mL), Cu(OTf)2 (0.15 mmol) was added. The reaction mixture was stirred for 2 h at room temperature under nitrogen atmosphere. Dienophile 2 (0.50 mol) dissolved in anhydrous CH2C12 (2 mL) was added to the above solution and then diene 1 (1.0 mmol) was added. The reaction mixture was stirred for 24 h at 0 °C. The reaction was quenched with saturated K2CO3 aq. (2 mL) and the product was extracted with CHCl3. The organic layer was dried over sodium sulfate, filtered, and concentrated under a reduced pressure. The residue was purified by flash column chromatography on silica gel (eluent, from n-hexane/AcOEt = 4:1 to n-hexane/AcOEt = 2:1). The reaction conditions, chemical yields and enantioselectivity are shown in Table 1. In the D-A reaction with molecular sieves 4A, the catalyst and molecular sieves 4A (230 mg) were stirred together for 2 h in anhydrous CH2C12 (2 mL), followed by the addition of dienophile 2 and then 1,2-dihydropyridine 1. The reaction conditions, chemical yields and enantioselectivity are shown in Table 2. In the reaction using Cu(ClO4)2 as a Lewis acid, a mixture of chiral t-Bu-oxazoline (0.090 mmol), CuCl2 (0.075 mmol) and AgClO4 (0.15 mmol) was stirred in anhydrous CH2Cl2 (2 mL) for 2 h under the dark conditions. The mixture was then filtered though a syringe filter and the filtrate containing chiral catalyst C’ was added to a solution of dienophile 2. The reaction conditions, chemical yields and enantioselectivity are shown in Table 3. The enantiomeric excess (% e.e.) of endo-3, 4a, or 5 was determined by HPLC analysis using a DAICEL Chiralpak AD-H column; eluent, 50% 2-propanol/n-hexane; flow rate, 0.5 mL/min, detection at 254 nm; retention time, tR = 14 min (major, 7S), tR= 17 min (minor, 7R). The enantiomeric excess (% e.e.) of endo-4b was determined by HPLC analysis using a DAICEL Chiralpak AD-H column; eluent, 50% 2-propanol/n-hexane; flow rate, 0.5 mL/min, detection at 254 nm; retention time, tR = 20 min (major, 7S), tR = 24 min (minor, 7R).
1.5.1 Diels-Alder adduct (7S)-3: White solid (recrystallized from AcOEt/n-hexane), mp 166-169 °C. (Lit.,3b mp 165-168 °C), [α]D20 +79.0 (c 0.25, CHCl3, 98% e.e.). 1H NMR [ppm] (500 MHz, CDCl3) δ 1.12-1.27 (m, 6H), 1.53-1.64 (m, 1H), 2.04-2.10 (m,1H), 2.53-2.59 (m, 1H), 2.64-2.69 (m,1H), 2.84 (br s, 1H), 3.05 (d, J = 10.3 Hz, 0.5H), 3.18 (d, J = 10.3 Hz, 0.5H), 3.35 (d, J = 9.6 Hz, 0.5H), 3.50 (d, J = 8.6 Hz, 0.5H), 3.99 (br s, 1H), 4.04 (br s, 2H). 5.06 (br s, 1H), 6.33-6.44 (m, 2H), 7.12 (d, J = 7.4 Hz, 1H), 7.16-7.24 (m, 3H), 7.28-7.31 (m, 2H), 7.33-7.38 (m, 2H), 7.43-7.44 (m, 2H); 13C NMR [ppm] (125 MHz, CDCl3) δ 25.77, 26.64, 27.48, 30.72, 43.47, 45.52, 46.75, 47.22, 57.08, 60.91, 121.77, 121.82, 125.17, 127.43, 127.48, 128.35, 128.85, 128.96, 129.17, 129.22, 130.80, 133.66, 137.56, 151.32, 153.37, 169.67, 173.91.
1.5.2 Diels-Alder adduct (7S)-4a: White solid (recrystallized from AcOEt/n-hexane), mp 134-138 °C. (Lit.,3b mp 170-172 °C), [α]D20 +92.9 (c 0.25, CHCl3, 99% e.e.). 1H NMR [ppm] (500 MHz, CDCl3) δ 1.28-1.41 (m, 6H), 1.61 (s, 2H), 2.68-2.70 (m, 2H), 2.84 (d, J = 17.2 Hz, 1H), 2.90-3.01 (m, 2H), 3.58 (m, 1H), 4.31 (m, 1H), 4.58 (m, 1H), 4.84-4.88 (m, 1H), 6.10 (t, J = 6.6 Hz, 0.5H), 6.17 (t, J = 6.6Hz, 0.5H), 6.28 (t, J = 7.1Hz, 1H), 7.12 (d, J = 8.0 Hz, 1H), 7.17-7.26 (m, 2H), 7.35-7.42 (m, 3H), 7.48-7.57 (m. 2H), 7.64 (m, 1H), 7.78 (t, J = 8.0 Hz, 1H), 7.86 (t, J = 8.6 Hz, 1H), 8.18-8.22 (m, 1H); 13C NMR [ppm] (125 MHz,CDCl3) δ 25.44, 26.74, 30.25, 30.50, 43.15, 46.43, 46.84, 47.36, 54.96, 61.26, 121.70, 123.25, 123.38, 125.13, 125.15, 125.33, 125.42, 125.73, 125.84, 126.38, 128.48, 128.74, 129.23, 129.26, 131.75, 133.65, 134.32, 151.40, 151.35, 153.13, 173.87.
1.5.3 Diels-Alder adduct (7S)-4b: White solid (recrystallized from AcOEt/n-hexane), mp 70-72 °C, [α]D20 +62.7 (c 0.50, CHCl3, 99% e.e.). IR (Nujol) 1225, 1497, 1688, 1743, 2963 cm-1. 1H NMR [ppm] (500 MHz, CDCl3) δ 1.19-1.39 (m, 6H), 1.65 (s,3H), 2.58 (s, 1H), 2.67 (d, J = 16.6 Hz, 1H), 2.76-2.98 (m, 3H), 4.28-4.29 (m, 0.5H), 4.49-4.56 (m, 1H), 4.64 (s, 1H), 4.87 (s, 1H), 5.10-5.26 (m, 2H), 6.04 (t, J = 6.87Hz, 0.5H), 6.14 (t, J = 6.8Hz, 0.5H), 6.20-6.24 (m, 1H), 7.31-7.42 (m, 6H), 7.43-7.55 (m, 2H), 7.67-7.72 (m, 1H), 7.80 (dd, J = 31.8, 8.3 Hz, 2H), 8.16 (dd, J = 23.7, 8.30 Hz, 1H); 13C NMR [ppm] (125 MHz, CDCl3) δ 25.47, 26.72, 30.27, 30.52, 43.13, 46.35, 46.42, 46.67, 55.07, 61.24, 66.71, 123.14, 123.24, 125.31, 125.41, 125.68, 125.84, 126.28, 126.38, 127.74, 127.90, 128.07, 128.21, 128.39, 128.71, 131.63, 133.64, 136.78, 136.89, 154.39, 154.75, 173.80. MS m/z 524.25 [M++1]. Anal. Calcd for: C, 73.40; H, 6.35; N, 8.02. Found: C, 73.34; H, 6.11; N, 7.96.
1.5.4 Diels-Alder adduct (7S)-5: White solid (recrystallized from AcOEt/n-hexane), mp 154-156 °C. [α]D20 +72.1 (c 0.25, CHCl3, 89% e.e.). IR (Nujol) 1205, 1595, 1716, 2969 cm-1. 1H NMR [ppm] (500 MHz, CDCl3) δ 1.12-1.29 (m, 6H), 1.47-1.49 (m, 1H), 2.04 (m, 1H), 2.69 (d, J = 24.6 Hz, 3H), 2.99 (d, J = 10.8 Hz, 0.5H), 3.12 (d, J = 10.3 Hz, 0.5H), 3.31 (d, J =10.3 Hz, 0.5H), 3.46 (d, J = 9.74 Hz, 0.5H), 3.98 (br s, 1H), 4.13-4.22 (m, 2H), 5.06 (d, J = 23.7 Hz, 1H), 6.09 (t, J =7.4 Hz, 1H), 6.17 (t, J = 6.9 Hz, 0.5H), 6.27 (t, J = 6.6 Hz, 0.5H), 7.11 (d, J = 8.0 Hz, 1H), 7.14-7.20 (m, 2H), 7.32-7.37 (m, 2H), 7.44-7.46 (m, 2H), 7.64-7.67 (m, 1H), 7.77-7.82 (m, 4H); 13C NMR [ppm] (125 MHz, CDCl3) δ 25.97, 27.49, 30.31, 30.61, 43.49, 45.70, 46.64, 47.19, 57.30, 60.01, 121.74, 121.80, 125.12, 125.79, 125.96, 127.08, 127.23, 127.44, 127.58, 127.65, 127.73, 127.94, 129.12, 129.16, 132.28, 134.09, 135.00, 151.27, 153.32, 169.68, 173.86. MS m/z 510.23 [M++1]. Anal. Calcd for: C, 73.06; H, 6.13; N, 8.25. Found: C, 73.24; H, 6.15; N, 8.14.
1.6 Conversion from Diels-Alder adduct 4a to benzy1 ester (7S)-6: n-BuLi (0.29 mL, 1.65 M in n-hexane, 0.48 mmol) was added to a cooled (-78 °C) solution of benzy1 alcohol (0.064 mL, 0.62 mmol) in 5 mL of THF. The resulting solution was strirred for 5 min and a solution of adduct 4a (0.30 mmol, 98% e.e.) in THF (1 mL) was added. The solution was warmed to 0 oC and stirred for 4 h. The mixture was then quenched with saturated NaHCO3 aq. and the solvent was concentrated under reduced pressure. Water was added to the residue and extracted with CHCl3. The combined organic layer was dried over anhydrous Na2SO4, filtered, and concentrated. The residue was purified by column chromatography on silica gel (eluent, 25% AcOEt in n-hexane) and benzyl ester (7S)-6 was obtained in 36% yield (39 mg, 0.11 mmol). The enantiomeric excess (98% e.e.) of benzyl ester (7S)-6 {[α]D27 +74.5 (c 0.39, DMSO)} as shown in Scheme 1 was determined by HPLC analysis using a DAICEL Chiralpak AD-H column; eluent, 5% 2-propanol/n-hexane; flow rate, 0.8 mL/min, detection at 254 nm; retention time, tR= 33.1 min (major, 7S), tR= 38.5 min (minor, 7R). The absolute stereochemistry of Diels-Alder adduct 4a was established to be (1S,4R,7S) by comparing the sign of the optical rotation with the literature value of (7R)-benzyl ester 6, { lit.,3a [α]D -59.92 (c 2.52, DMSO)}.
1.6.1 Benzyl ester (7S)-6: yield 39 mg, oil, 36%; [α]D27 +74.5 (c 0.39, DMSO; 98% e.e.); {Lit.,3a benzyl ester (7R) [α]D 25-59.92 (c 2.52, DMSO)}; The 1H NMR and 13C NMR spectra of (7S)-6 were identified with the literature values.3a 1H NMR (270 MHz, CDCl3) δ 1.93-1.95 (m, 2H), 2.89 (br s, 1H), 3.04 (d, J = 10.5 Hz, 0.5H), 3.15 (d, J = 10.0 Hz, 0.5H), 3.22-3.25 (m, 1H), 3.34 (d, J = 10.5 Hz, 0.5H), 3.48 (d, J =10.2 Hz, 0.5H), 5.04-5.12 (m, 2H), 5.15-5.24 (m, 1H), 6.31-6.36 (m, 1H), 6.45-6.50(m, 1H), 7.07-7.12 (m, 2H), 7.16-7.19 (m, 1H), 7.24-7.32 (m, 7H); 13C NMR [ppm] (125 MHz, CDCl3) δ 25.93, 30.60, 44.81, 46.91, 47.52, 66.56, 121.65, 212.72, 125.25, 128.07, 128.16, 128.21, 128.51, 128.55, 129.17, 129.21, 130.54, 135.69, 151.22, 153.58, 153.61, 172.33.
ACKNOWLEDGMENTS
We are grateful to Ms. Yoko Nakano, Ms. Satomi Ahiko, and Mr. Hiroyuki Momma (Research and Analytical Center for Giant Molecules, Graduate School of Sciences, Tohoku University) for the elemental analysis and mass spectra measurements.
References
1. (a) M. E. Kuehne and I. Marko, ‘Syntheses of Vinblastine-Type Alkaloids. The Alkaloids. Antitumor Bisindole Alkaloids from Catharanthus roseus (L)’, Vol. 37, ed. by A. Brossi and M. Suffness, Academic Press, Inc, San Diego, 1990, pp. 77-131; CrossRef (b) P. Popik and P. Skolnick, ‘The Alkaloids. Chemistry and Biology: Pharmacology of Ibogaine and Ibogaine-related Alkaloids’, Vol. 52, ed. by G. A. Cordell, Academic Press, Inc, San Diego, 1999, pp. 197-231; (c) E. McCoy and S. E. O’Connor, J. Am. Chem. Soc., 2006, 128, 14276. CrossRef
2. G. R. Krow, O. H. Cheung, Z. Hu, Q. Huang, J. Hutchinson, N. Liu, K. T. Nguyen, S. Ulrich, J. Yuan, Y. Xiao, D. M. Wypij, F. Zuo, and P. J. Carrol, Tetrahedron, 1999, 55, 7747. CrossRef
3. The detailed syntheses of pyrazolidinone 2a, 2b and 2c, see: (a) H. Nakano, N. Tsugawa, and R. Fujita, Tetrahedron Lett., 2005, 46, 5677; CrossRef (b) H. Nakano, N. Tsugawa, K. Takahashi, Y. Okuyama, and R. Fujita, Tetrahedron, 2006, 62, 10879. CrossRef
4. M. O. F. Khan, M. S. Levi, B. L. Tekwani, N. H. Wilson, and R. F. Borne, Bioorg. Med. Chem., 2007, 15, 3919. CrossRef
5. N. Sato, T. Akiba, S. Yokoshima, and T. Eukuyama, Angew. Chem. Int. Ed., 2007, 46, 5734. CrossRef
6. For 1,2-dihydropyridine possessing a chiral center: (a) Y. Matsumura, Y. Nakamura, T. Maki, and O. Onomura, Tetrahedron Lett., 2000, 41, 7685; CrossRef Dihydropyridine having a chiral auxiliary attached to the nitrogen: (b) M. Mehmandoust, C. Marazano, R. Singh, B. Gillert, M. Cesario, J.-L. Fourrey, and B. C. Das, Tetrahedron Lett., 1988, 29, 4423; CrossRef (c) D. C. dos Santos, R. P. de Freitas Gil, L. Gil, and C. Marazano, Tetrahedron Lett., 2001, 42, 6109; CrossRef (d) C. Marazano, Y. Yannic, M. Mehmandoust, and B. C. Das, Tetrahedron Lett., 1990, 31, 1995; CrossRef Diels-Alder reaction in which the dienophile has a chiral auxiliary: (e) C. Kouklovsky, A. Pouihes, and Y. Langlois, J. Am. Chem. Soc., 1990, 112, 6672; CrossRef (f) M. M. Campbell, M. Sainsbury, P. A. Searle, and G. M. Davis, Tetrahedron Lett., 1992, 33, 3181; CrossRef (g) M. Hirama, Y. Kato, C. Seki, H. Matsuyama, N. Oshikiri, and M. Iyoda, Chem. Lett., 2008, 37, 924; CrossRef (h) M. Hirama, Y. Kato, C. Seki, H. Nakano, M. Takeshita, N. Oshikiri, M. Iyoda, and H. Matsuyama, Tetrahedron, 2010, 66, 7618; CrossRef (i) M. Hirama, C. Suttibut, N. D. M. R. Hutabarat, C. Seki, N. Sakuta, T. Tsuchiya, Y. Kohari, H. Nakano, K. Uwai, N. Takano, M. Yasui, Y. Okuyama, M. Osone, M. Takeshita, and H. Matsuyama, Heterocycles, 2012, 84, 377; CrossRef (j) C. Seki, M. Hirama, N. D. M. R. Hutabarat, J. Takada, C. Suttibut, H. Takahashi, T. Takaguchi, Y. Kohari, H. Nakano, K. Uwai, N. Takano, M. Yasui, Y. Okuyama, M. Takeshita, and H. Matsuyama, Tetrahedron, 2012, 68, 1774; CrossRef Enantioselective Diels-Alder reaction of dihydropyridines: Pd-POZA catalyst: (k) ref. 3(a); (l) ref. 3(b); Cr(III) salen complex catalyst: (m) N. Takenaka, Y. Huang, and V. H. Rawal, Tetrahedron, 2002, 58, 8299; CrossRef Chiral oraganocatalysts: (n) H. Nakano, K. Osone, M. Takeshita, E. Kwon, C. Seki, H. Matsuyama, N. Takano, and Y. Kohari, Chem. Commun., 2010, 4827; CrossRef (o) C. Suttibut, Y. Kohari, K. Igarashi, H. Nakano, M. Hirama, C. Seki, H. Matsuyama, K. Uwai, N. Takano, Y. Okuyama, K. Osone, M. Takeshita, and E. Kwon, Tetrahedron Lett., 2011, 52, 4745. CrossRef
7. For previews of uses of bisoxazoline-derived ligands: (a) D. A. Evans, S. J. Miller, and T. Lectka, J. Am. Chem. Soc., 1993, 115, 6460; CrossRef (b) D. A. Evans, S. J. Miller, T. Lectka, and P. v. Matt, J. Am. Chem. Soc., 1999, 121, 7559; CrossRef (c) D. A. Evans, K. A. Woerpel, M. M. Hinman, and M. M. Faul, J. Am. Chem. Soc., 1991, 113, 726; CrossRef (d) E. J. Corey, N. Imai, and H-Y. Zhang, J. Am. Chem. Soc., 1993, 113, 728; CrossRef (e) A. Sakakura, R. Kondo, and K. Ishihara, Org. Lett., 2005, 7, 1971. CrossRef
8. For general reviews of chiral bis(oxazoline), see: (a) R. Rasappan, D. Laventine, and O. Reiser, Coordination Chemistry Reviews, 2008, 252, 702; CrossRef (b) D. Carmona, M. P. Lamata and L. A. Oro, Coordination Chemistry Reviews, 2000, 200-202, 717; CrossRef (c) L. C. Diaz, J. Braz. Chem. Soc., 1997, 8, 289; CrossRef (d) A. K. Ghosh, P. Mathivanan, and J. Cappiello, Tetrahedron: Asymmetry, 1998, 9, 1; CrossRef (e) G. Desimoni, G. Faita, and K. A. Jorgensen, Chem. Rev., 2006, 106, 3561. CrossRef
9. F. W. Fowler, J. Org. Chem., 1972, 37, 1321. CrossRef
10. (a) M. P. Sibi and J. Ji, J. Am. Chem. Soc., 1996, 118, 9200; CrossRef (b) M. P. Sibi, L. Venkatraman, M. Liu, and C. P. Jasperse, J. Am. Chem. Soc., 2001, 123, 8444; CrossRef (c) M. P. Sibi, L. M. Stanley, X. Nie, L. Venkatraman, M. Liu, and C. P. Jasperse, J. Am. Chem. Soc., 2007, 129, 395. CrossRef
11. (a) D. A. Evans, K. A. Woerpel, B. Nosse, A. Schall, Y. Shinde, E. Jezek, M. M. Haque, R. B. Chhor, and O. Reiser, Organic Syntheses, 2006, 83, 97; CrossRef (b) X. Hui, J. Huang, S. Chiou, and H. Gau, J. Chin. Chem. Soc., 2006, 53, 421.