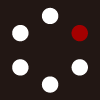
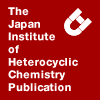
HETEROCYCLES
An International Journal for Reviews and Communications in Heterocyclic ChemistryWeb Edition ISSN: 1881-0942
Published online by The Japan Institute of Heterocyclic Chemistry
e-Journal
Full Text HTML
Received, 19th March, 2012, Accepted, 24th April, 2012, Published online, 2nd May, 2012.
DOI: 10.3987/COM-12-12468
■ Floral Pigments Isolated from the Sky-Blue Flowers of Oxypetalum caeruleum
Norio Saito, Kenjiro Toki, Toshio Honda, and Fumi Tatsuzawa*
Laboratoryof Olericultural and Floricultural Science, Faculty of Agriculture, Iwate University, 3-18-8 Ueda, Morioka, Iwate 020-8550, Japan
Abstract
Three anthocyanins (pigments 1 - 3) were isolated from the sky-blue flowers of Oxypetalum caeruleum ‘Caerulea’ as the floral anthocyanins. These three anthocyanins were elucidated to be cyanidin 3-O-[2-O-(β-xylopyranosyl)-β-glucopyranoside]-7-O-β-glucopyranoside (1), cyanidin 3-sambubioside (2), and cyanidin 3-O-[2-O-(β-xylopyranosyl)-β- glucopyranoside]-7-O-[6-O-(trans-feruloyl)-β-glucopyranoside] (3), respectively, by chemical and spectroscopic means. Pigment 1 was present in the flowers as a main anthocyanin, and pigment 3 was a new anthocyanin in plants. Three flavonols glycosides were also isolated from these flowers, and identified to be the 3-O-(2-O-(β-xylopyranosyl)-β-glucopyranoside)s of quercetin (pigment 4) and kaempferol (pigment 5) and kaempferol 3-glucoside (pigment 6). Moreover, the bluing effect on the flower color of O. caeruleum ‘Caerulea’ was discussed.INTRODUCTION
Oxypetalum caerulea ‘Caerulea’(Asclepiadaceae) is a popular cut-flower plant with attractive sky-blue flowers in Japan. In our continuing investigation on flower color variation due to anthocyanins in the ornamental plants, we were interested in the chemical investigation of the sky-blue floral pigments of O. caeruleum ‘Caerulea’, since this species has not been thoroughly studied to date.1-4 In this study, cyanidin 3,7-glycosides were found in the sky-blue flowers of this plant as its main anthocyanins as in the case of those pigments in the blue flowers of Corydalis flexuosa5 and Meconopsis grandis,6,7 together with three flavonols glycosides. In this paper, we wish to report the isolation and structure elucidation of anthocyanins and flavonol glycosides from the sky-blue flowers of O. caerulea ‘Caerulea’.
RESULTS AND DISCUSSION
Three anthocyanin peaks and three flavonols peaks were observed in 5% HOAc-H2O extract from the sky-blue flowers of O. caeruleum ‘Caerulea’ as major peaks by HPLC analysis with monitoring at 530 nm for anthocyanins and at 350 nm for flavonols. The proportion of these anthocyanin peaks were 74.6% (pigment 1), 10.5% (pigment 2), and 4.8% (pigment 3) based on a percentage of the total absorbance of anthocyanin peaks, and those of flavonols peaks were 6.4% (pigment 4), 41.2% (pigment 5), and 14.7% (pigment 6) based on a percentage of the total absorbance of flavonols peaks.
These six pigments 1 - 6 were purified using Diaion HP-20 resin column chromatography (CC), paper chromatography and preparative HPLC described previously.5 Chromatographic and spectroscopic properties of these pigments are shown in Table 1 and in Experimental Section. The FAB mass measurement of pigments 1 – 6 gave their molecular ions [M]+ m/z for anthocyanins and [M+H]+ m/z for flavonols at 743 (pigment 1), 581 (pigment 2), 919 (pigment 3), at 597 (pigment 4), 581 (pigment 5), and 449 (pigment 6), respectively.
Acid hydrolysis of anthocyanins (pigments 1 – 3) resulted in cyanidin, glucose, and xylose. Moreover, ferulic acid was detected in the hydrolysate of pigment 3 by analysis of TLC and HPLC. Acid hydrolysis of flavonols (pigments 4 – 6) resulted in kaempferol for pigments 5 and 6 as their aglycone, and quercetin for pigment 4. On their sugar components, glucose was observed in all hydrolysates, but xylose was detected only in hydrolysates of pigments 4 and 5. Furthermore, upon alkaline hydrolysis of pigment 3 resulted in ferulic acid and one deacylanthocyanin, which was identical with pigment 1 by comparison of TLC and HPLC.
From these results, the structures of pigments 1 – 6 were presumed to be cyanidin 3-sambubioside-7-glucoside (pigment 1), cyanidin 3-sambubioside (pigment 2), feruloylcyanidin 3-sambubioside-7-glucoside (pigment 3), quercetin 3-sambubioside (pigment 4), kaempferol 3-sambubioside (pigment 5), and kaempferol 3-glucoside (pigment 6), respectively.
The structure of pigment 2 was confirmed to be cyanidin 3-sambubioside by analysis of UV-Vis, TLC and HPLC in direct comparison with the authentic specimen obtained from Corydalis ambigua,5 and pigment 6 was confirmed to be kaempferol 3-glucoside in comparison with the authentic standard purchased from Extrasynthese (France). The elemental components of both pigments were also confirmed by measuring their high-resolution FAB MS spectra (see Table 1 and EXPERIMENTAL).
The other four pigments 1, 3, 4, and 5 were elucidated based on the analysis of their 1H and/or 13C NMR spectra [400 MHz for 1H and 100 MHz for 13C NMR spectra, including 2D COSY, NOESY, C-H COSY and COLOC] (See Table 2 and Experimental Section).
Pigment 1 (= deacyl pigment 3)
The FAB mass spectra of 1 and deacyl pigment 3 gave a same molecular ion [M]+ at 743 m/z (calc. for C32H39O20) indicating that pigment 1 and deacyl pigment 3 are composed of cyanidin with two molecules of glucose and one molecule of xylose. The elemental components of pigment 1 were confirmed by measuring its high-resolution FAB MS (calc. C32H39O20: 743.2035, found 743.2017). The 1H NMR spectrum of pigment 1 exhibited six aromatic protons being identified with those of cyanidin moiety5 (Table 2). The anomeric protons of sugar moieties were assigned at δ 5.64 (d, J = 7.6 Hz, Glu A-1), δ 5.20 (d, J = 7.4 Hz, Glu B-1) and δ 4.65 (d, J = 7.8 Hz, xylose-1), and these sugars were determined to be in β-pyranose forms from their observed J values. By application of NOESY experiments, the long range NOEs between H-1 of Glu A and H-4 (δ 8.84) of cyanidin, between H-1 of Glu B and H-8 (δ 7.33) and H-6 (δ 6.80) of cyanidin, and also between H-1 of xylose and H-2 (δ 3.89) of Glu A were observed (Fig. 1), suggesting that OH-3 and OH-7 of cyanidin are glycosylated with Glu A and Glu B, respectively, and OH-2 of Glu A is glycosylated with xylose. Therefore, pigment 1 (= deacyl pigment 3) was determined to be cyanidin 3-O-[2-O-(β-xylopyranosyl)-β-glucopyranoside]-7-O-β-glucopyranoside. This structure was further confirmed by the measurement of 13C NMR spectra as shown in Table 2.
Pigment 3
The FAB mass spectrum of pigment 3 gave a molecular ion [M]+ at 919 m/z (calc. for C42H47O23) indicating that pigment 3 is composed of cyanidin with two molecules of glucose and one molecule each of xylose and ferulic acid. The elemental components were confirmed by measuring its high-resolution FAB MS (calc. C42H47O23: 919.2508, found 919.2502).
The 1H NMR spectrum of pigment 3 was superimposed on that of pigment 1 except for the signals of ferulic acid and Glu B moieties. The proton chemical shifts of the methylene (-CH2-) group of Glu B were shifted to a lower magnetic field at δ 4.23 and 4.56 indicating that Glu B was acylated with ferulic acid at OH-6 of Glu B. Three aromatic proton signals of ferulic acid were observed at δ 7.11, δ 6.66 and δ 6.81. Two olefinic protons were exhibited at δ 7.46 and 6.24 with large coupling constants (J = 15.9 and 15.9 Hz) (Table 2) suggesting that this ferulic acid was in the trans configulation. Therefore、the structure of pigment 3 was determined to be cyanidin 3-O-[2-O-(β-xylopyranosyl)-β-glucopyranoside]-7-O- [6-O-(trans-feruloyl)-β-glucopyranoside], which is a new anthocyanin in plants.1-4
Pigments 4 and 5
The FAB mass spectra of pigments 4 and 5 gave their molecular ions [M+H]+ at 597 m/z (calc. C26H29O16) and at 581 m/z (calc. C26H29O15) indicating that pigment 4 is composed of quercetin with one molecule each of glucose and xylose, and pigment 5 is composed of kaempferol with one molecule each of glucose and xylose. Their elemental components were confirmed by measuring their high-resolution FAB MS (see EXPERIMENTAL).
The 1H NMR spectrum of pigment 4 was similar to that of pigment 5 except for the signals of their aglycone moieties (see Experimental Section), in which the aromatic proton signals of pigment 4 were observed at δ 6.20 (H-6), δ 6.41 (H-8), δ 7.57 (H-2’), δ 6.86 (H-5’), and δ 7.68 (H-6’), and those of pigment 5 were exhibited at δ 6.21 (H-6), δ 6.45 (H-8), δ 8.11 (H-2’ and 6’), and δ 6.91 (H-3’ and 5’) (see Experimental Section).
The proton chemical shifts of sugar moieties of both pigments were observed in the regions of δ 5.73 – 3.08 for pigment 4 and δ 5.73 – 3.04 for pigment 5, where two anomeric protons were observed at δ 5.73 (d, J = 7.4 Hz, Glu C) and δ 4.59 (d, J = 7.1 Hz, xylose) for pigment 4, and at δ 5.73 (d, J = 7.4 Hz, Glu C) and δ 4.61 (d, J = 7.3 Hz, xylose) for pigment 5. Based on the observed coupling constants (see Experimental Section), these glucose and xylose were determined to be β-pyranose forms. In the NOESY spectra of pigments 4 and 5, the correlations between H-2 of Glu C and H-1 of xylose were observed in both pigments indicating that OH-2 of Glu C was bonded with OH-1 of xylose, respectively. Since the hypsochromic effects at main absorption maxima of both pigments 4 and 5 were observed in UV spectroscopic experiments, the glycosylations at OH-3 of both aglycones were ascertained.8
Therefore, the structures of both pigments were determined to be quercetin 3-O-[2-O-(β-xylopyranosyl)-β-glucopyranoside] for pigment 4, and kaempferol 3-O-[2-O-(β-xylopyranosyl)-β-glucopyranoside] for pigment 5. Both structures were confirmed by measuring 13C NMR spectra (see Experimental Section).
Flower color and anthocyanins of Oxypetalum caeruleum.
Based on the above results, the presence of cyanidin 3-sambubioside-7-glucoside (pigment 1), cyanidin 3-sambubioside (pigment 2), cyanidin 3-sambubioside-7-feruloylglucoside (pigment 3), quercetin 3-sambubioside (pigment 4), kaempferol 3-sambubioside (pigment 5), and kaempferol 3-glucoside (pigment 6) were found in the sky-blue flowers (Blue 107C by R.H.S. Colour Chart) of Oxypetalum caeruleum ‘Caerulea’. Among them, cyanidin 3-sambubioside-7-glucoside and kaempferol 3-sambubioside were recognized as the main floral pigments, and cyanidin 3-sambubioside-7-feruloylglucoside was realized to be a new anthocyanin in plants.1-4 Thus far, cyanidin 3-(6G-rhamnosylsambubioside)-7-glucoside was reported to be involved in the blue flowers (Blue 106A) of Corydalis flexuosa cultivars as a main anthocyanin5 concerned with the floral anthocyanins in the similar blue flowers, and also cyanidin 3-malonylsambubioside-7-glucoside was found in the blue flowers (Blue 106A) of Meconopsis grandis together with several flavonol glycosides.6,7 By measurement of absorption spectra in the visible region 360 – 700 nm, the fresh petal-tissues of these three plants exhibited two similar strong absorption maxima at 644 – 656 nm and at 600 – 605 nm with a weak shoulder at near 559 nm (see Experimental Section). Furthermore, the restoration study of the blue flower color of M. grandis was recently performed by mixing cyanidin 3-malonylsambubioside-7-glucoside with kaempferol glycosides and some metal ions.7 Based on the consideration of these results, it might be considered that cyanidin 3-sambubioside-7-glucoside takes part in producing the sky-blue flower color of O. caeruleum ‘Caerulea’ as an important component as those of C. flexuosa and M. grandis, and also kaempferol 3-glucoside plays an important role as a co-pigment in producing its blue color in O. caeruleum. The detail restoration study of this sky-blue flower color with cyanidin 3-sambubioside-7-glucoside will be reported elsewhere.
EXPERIMENTAL
General procedures
TLC was carried out on plastic sheets or glass plate coated cellulose (Merck) using ten mobile phases: BAW (n-BuOH-HOAc-H2O, 4:1:5), BuHCl (n-BuOH-2N HCl, 1:1), HOAc-HCl (HOAc-HCl-H2O, 15:3:82), 1% HCl for flavonoids, and BAW, H2O, and 6% HOAc for hydroxycinnamic acids with UV light, IPW (i-PrOH-H2O, 4:1), IPB (i-PrOH-n-BuOH-H2O, 7:1:2), and PhOH (PhOH-H2O, 4:1) for sugars with aniline hydrogen phthalate spray reagent, and BAW, PhOH, and Forestal (HOAc-HCl-H2O, 30:3:10) for aglycone. The identification of flavonoids, sugars, and acid were carried out by standard procedures.8,9
Analytical HPLC was performed on Shimadzu LC 10A system, using a Waters C18 (4.6 x 250 mm) column at 40 °C with a flow rate of 1 mL/min and monitoring at 530 nm for anthocyanin and 350 nm for flavonol. The eluant was applied as a linear gradient elution for 40 min from 20% to 85% solvent B (1.5% H3PO4, 20% HOAc, 25% MeCN in H2O) in solvent A (1.5% H3PO4 in H2O).
UV-Vis spectra were recorded on UV-Vis spectrophotometer (MPS-2450, Shimadzu) in 0.1% HCl- MeOH for anthocyanin and in MeOH for flavonols. The spectral absorption of flowers was directly measured on the intact tissue using a recording spectrophotometer operating as a double-beam instrument (Type: MPS-2450).10 FAB mass spectra (JEOL JMS-700) were obtained in the positive ion mode using 1:1 mixture of dithiothreitol and 3-nitrobenzyl alcohol as a matrix.
NMR spectra (JEOL AL-400) were acquired at 400 MHz for 1H spectra and 100 MHz for 13C spectra in DMSO-d6 for flavonols and DMSO-d6-CF3CO2D (9:1) for anthocyanins. Chemical shifts are reported relative to a TMS internal standard (δ), and coupling constants are in Hz.
Plant materials
Seeds of O. caeruleum ‘Caerulea’ were purchased from Sakata Seed Co., Ltd, Yokohama. The plants were grown in the green house of Minami-Kyushu University. The fresh flower sepals were collected in spring – autumn, and dried at 45 °C, and stored in desiccators until needs. The flower colors of these plants were recorded by comparing them directly with the Royal Horticultural Society (R.H.S.) Colour Chart. The chromaticity value of the fresh sky-blue flowers of this cultivar, b/a = -11.7/2.3 = -5.1 by ND101-DP Spectro Color Meter (Nippon Denshoku Industries Co., Ltd.). The absorption spectrum of the fresh petal of O. caeruleum ‘Caerulea’ (Blue 107C by R.H.S. Colour Chart) exhibited characteristic absorption maxima at 656, 600, 559sh nm in the visible region 360 – 700 nm, which were comparable to those of Corydalis flexuosa ‘Blue Panda’ (Blue 106A); 644, 604, 559sh nm, and Meconopsis grandis (Blue 106A); 649, 605, 559sh nm.
Isolation of flavonols and anthocyanin
The dried flowers (100 g) were extracted with 5% HOAc (10 L). Flavonols and anthocyanins in the extract were absorbed on a Diaion HP-20 column, and after re-extracted with 5% HOAc-MeOH, the eluant was concentrated into a small volume. The concentrated extract was fractionated with Sephadex LH-20 CC using MeOH-HOAc-H2O (6:1:12). The fractions were further purified with PC (n-BuOH-HOAc-H2O, 4:1:2 and 15% HOAc) and prep. HPLC. Prep. HPLC was performed on a Shimadzu LC 10A system using a Waters C18 column (19 x 150 mm) column at 40 °C with a flow rate of 4 ml/min-1. Three pigments, 1 (35 mg), 2 (5 mg), and 3 (2 mg) as dark red powders, and 4 (600 mg), 5 (60 mg), and 6 (10 mg) as a pale yellow powders, were obtained.
Cyanidin 3-sambubioside-7-glucoside (1)
Dark red powder; for UV-Vis, TLC and HPLC see Table 1, and for 1H and 13C NMR spectroscopic assignments see Table 2. HR-FAB MS calc. for C32H39O20 [M]+ 743.2035, Found 743.2017.
Cyanidin 3-sambubioside (2)
Dark red powder, for UV-Vis, TLC and HPLC see Table 1, and for HR-FAB MS calc. for C26H29O15 [M]+ 581.1506, Found 581.1511.
Cyanidin 3-sambubioside-7-feruloyl-glucoside (3)
Dark red powder; for UV-Vis, TLC and HPLC see Table 1, and for 1H and 13C NMR spectroscopic assignments see Table 2. HR-FAB MS calc. for C42H47O23 [M]+ 919.2508, Found 919.2502.
Quercetin 3-sambubioside (4)
Pale yellow powder, TLC (Rf values x 100); BAW 67, BuHCl 66, 1% HCl 29, HOAc-HCl 87, UV brown, UV/NH3 yellow, UV (λmax nm); MeOH 356, 304sh, 265sh, 256, +NaOMe 374, 323, 273, +AlCl3 432, 331, 301sh, 274, +AlCl3/HCl 399, 359, 298, 269, +NaOAc 363, 326sh, 268, 259sh, +NaOAc/H3BO3 376, 295, 261, HPLC (Rt min) 20.6, HR-FAB MS calc. for C26H29O16 [M+H]+ 597.1456, Found 597.1443.
1H NMR (400 MHz, DMSO-d6, an internal standard of TMS); δ Quercetin: 7.68 (dd, J = 2.2, 8.5 Hz, H-6’), 7.57 (d, J = 2.2 Hz, H-2’), 6.86 (d, J = 8.5 Hz, H-5’), 6.41 (d, J = 2.0 Hz, H-8), 6.20 (d, J = 2.0 Hz, H-6), Glu C: 5.73 (d, J = 7.4 Hz, H-1), 3.48 (t, J = 8.8 Hz, H-2), 3.14 (t, J = 8.8 Hz, H-3), 3.12 (m, H-4), 3.48 (m, H-5), 3.33 (m, H-6a), 3.55 (brd, J = 11.2 Hz, H-6b), Xylose: 4.59 (d, J = 7.1 Hz, H-1), 3.04 (t, J = 7.7 Hz, H-2), 3.12 (m, H-3), 3.27 (m, H-4), 3.05 (t, J = 11.2 Hz, H-5a), 3.69 (dd, J = 5.1, 11.2 Hz, H-5b). 13C NMR (100 MHz, DMSO-d6, an internal standard of TMS); δ Quercetin: 155.4 (C-2), 133.0 (C-3), 177.4 (C-4), 161.3 (C-5), 98.6 (C-6), 164.1 (C-7), 93.4 (C-8), 156.3 (C-9), 104.0 (C-10), 121.2 (C-1’), 116.0 (C-2’), 145.0 (C-3’), 148.6 (C-4’), 115.3 (C-5’), 122.0 (C-6’), Glu C: 97.9 (C-1), 81.9 (C-2), 76.2 (C-3), 69.6 (C-4), 76.9 (C-5), 60.7 (C-6), Xylose: 104.6 (C-1), 74.0 (C-2), 77.7 (C-3), 69.4 (C-4), 65.7 (C-5).
Kaempferol 3-sambubioside (5)
Pale yellow powder, TLC (Rf values x 100); BAW 81, BuHCl 82, 1% HCl 39, HOAc-HCl 90, UV brown, UV/NH3 dark yellow, UV (λmax nm); MeOH 349, 325sh, 301sh, 266, +NaOMe 371, 302, 273, +AlCl3 391, 351, 303, 273, +AlCl3/HCl 392, 346, 301, 274, +NaOAc 352, 316sh, 304sh, 267, +NaOAc/H3BO3 349, 325sh, 302sh, 266, HPLC (Rt min) 24.1, HR-FAB MS calc. for C26H29O15 [M+H]+ 581.1506, Found 581.1520.
1H NMR (400 MHz, DMSO-d6, an internal standard of TMS); δ Kaempferol: 8.11 (d, J = 9.0 Hz, H-2’,6’), 6.91 (d, J = 9.0 Hz, H-3’,5’), 6.45 (d, J = 2.0 Hz, H-8), 6.21 (d, J = 2.0 Hz, H-6), Glu C: 5.73 (d, J = 7.4 Hz, H-1), 3.43 (t, J = 8.2 Hz, H-2), 3.16 (t, J = 8.8 Hz, H-3), 3.11 (t, J = 8.3 Hz, H-4), 3.48 (m, H-5), 3.31 (m, H-6a), 3.54 (brd, J = 11.5 Hz, H-6b), Xylose: 4.61 (d, J = 7.3 Hz, H-1), 3.08 (t, J = 8.1 Hz, H-2), 3.12 (t, J = 7.6 Hz, H-3), 3.29 (m, H-4), 3.08 (t, J = 11.2 Hz, H-5a), 3.73 (dd, J = 5.1, 11.2 Hz, H-5b). 13C NMR (100 MHz, DMSO-d6, an internal standard of TMS); δ Kaempferol: 155.3 (C-2), 132.9 (C-3), 177.5 (C-4), 161.3 (C-5), 98.7 (C-6), 164.1 (C-7), 93.6 (C-8), 156.3 (C-9), 104.0 (C-10), 120.9 (C-1’), 131.0 (C-2’,5’), 115.2 (C-3’,5’), 160.0 (C-4’), Glu C: 97.9 (C-1), 81.9 (C-2), 76.3 (C-3), 69.6 (C-4), 76.9 (C-5), 60.5 (C-6), Xylose: 104.6 (C-1), 74.0 (C-2), 77.6 (C-3), 69.5 (C-4), 65.8 (C-5).
Kaempferol 3-glucoside (6)
Pale yellow powder, TLC (Rf values x 100); BAW 89, BuHCl 90, 1% HCl 10, HOAc-HCl 79, UV brown, UV/NH3 dark yellow, UV (λmax nm); MeOH 348, 324, 300, 266, +NaOMe 377, 305, 274, +AlCl3 393, 350, 304, 273, +AlCl3/HCl 392, 346, 302, 274, +NaOAc 351, 319sh, 304sh, 267, +NaOAc/H3BO3 349, 324sh, 301sh, 266, HPLC (Rt min) 27.2, HR-FAB MS calc. for C21H21O11 [M+H]+ 449.1084, Found 449.1081.
Aglycones, glucose, xylose, and ferulic acid
Acid hydrolysis of 1 – 6 (ca. 0.5 mg each) was achieved by 2N HCl (1 mL) at 90 °C for 2 h. Moreover, Alkaline hydrolysis of 1 – 6 (ca. 0.5 mg each) was achieved by 2N NaOH (1 mL) using a degassed syringe to stir for 15 min. The solution was then acidified with 2N HCl (1.1 mL). These solutions were used for TLC and HPLC.9 Products in these hydrolysates were identified in comparison with the authentic standards of glucose, xylose, ferulic acid, kaempferol, and quercetin, which were of commercial origins. Cyanidin was obtained from the flowers of Matthiola incana.9
ACKNOWLEDGEMENTS
This work was supported in part by a Grant-in-Aid for Science Research (C) from the Japan Society for the Promotion of Science (JSPS).
References
1. J. B. Harborne and H. Baxter, “The Handbook of Natural Flavonoids,” John Wiley & Sons. Chichester, 1999.
2. Ø. M. Andersen and M. Jordheim, “Flavonoids: Chemistry, Biochemistry and Aplications,” CRC Press, Boca Raton, 2006. p. 471.
3. N. C. Veitch and R. J. Grayer, Flavonoids and their glycosides, including anthocyanins, Nat. Prod. Rep., 2008, 25, 555. CrossRef
4. N. C. Veitch and R. J. Grayer, Flavonoids and their glycosides, including anthocyanins, Nat. Prod. Rep., 2011, 28, 1625. CrossRef
5. F. Tatsuzawa, Y. Mikanagi, N. Saito, K. Shinoda, A. Shigihara, and T. Honda, Biochemical Systematics and Ecology, 2005, 33, 789. CrossRef
6. M. Tanaka, T. Fujimori, I. Uchida, S. Yamaguchi, and K. Takeda, Phytochemistry, 2001, 56, 373. CrossRef
7. K. Yoshida, S. Kitahara, D. Ito, and T. Kondo, Phytochemistry, 2006, 67, 992. CrossRef
8. J. B. Harborne, “Phytochemical Methods”, Second ed., Chapman and Hall, London, 1984. CrossRef
9. F. Tatsuzawa, N. Saito, K. Toki, K. Shinoda, and T. Honda, J. Japan. Soc. Hort. Sci., 2012, 81, 91. CrossRef
10. M. Yokoi and N. Saito, Phytochemistry, 1973, 12, 1783. CrossRef