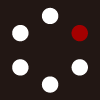
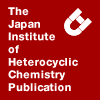
HETEROCYCLES
An International Journal for Reviews and Communications in Heterocyclic ChemistryWeb Edition ISSN: 1881-0942
Published online by The Japan Institute of Heterocyclic Chemistry
e-Journal
Full Text HTML
Received, 22nd March, 2012, Accepted, 6th July, 2012, Published online, 17th July, 2012.
DOI: 10.3987/COM-12-12472
■ Microwave-Assisted Synthesis of 3,1-Benzoxazin-2-ones from 3-Hydroxyoxindoles
Oscar R. Suárez-Castillo,* Claudia I. Bautista-Hernández, Maricruz Sánchez-Zavala, Myriam Meléndez-Rodríguez, Araceli Sierra-Zenteno, Martha S. Morales-Ríos, and Pedro Joseph-Nathan
Área Académica de Química, Universidad Autónoma del Estado de Hidalgo, Mineral de la Reforma, Hidalgo, 42184, Mexico
Abstract
A general protocol for the synthesis of 3,1-benzoxazin-2-ones 18 from 3-hydroxyoxindoles 16 in a two steps sequence through phenylsuccinates or phenylpropionates 17 is described. Best reaction conditions for ring opening of 16 to succinates or propionates 17 were achieved using alcohol/silica gel, while cyclization of 17 to benzoxazinones 18 was easily done with HCl/alcohol. It was also found that 17 and 18 can be transesterified using HCl/alcohol. Most transformations were carried out by traditional heating and by microwave (MW) irradiation to accelerate reaction rates.INTRODUCTION
3,1-Benzoxazin-2-one derivatives have received considerable attention due to their strong biological activity.1 For example, Sustive® (Efavirenz, 1, Figure 1) is one of the most used non-nucleoside reverse transcriptase drugs for combating HIV-1 replication.1a
Other 3,1-benzoxazin-2-one derivatives such as 2a-d have shown to be non-steroidal potent and selective progesterone receptor antagonists.1b,c As clinically successful anti HIV and progesterone receptor antagonists, including 3,1-benzoxazin-2-one derivatives, are scarce and their therapeutic potential has not yet been fully realized, some reports on the synthesis of these heterocycles have appeared. At this respect, it is important to continue to develop efficient methods for the synthesis of this class of heterocycles, especially routes based upon readily available starting materials. A few general approaches for synthesizing the benzoxazinone skeleton 3 have been reported so far, for which representative examples are shown in Scheme 1. They include reaction of the corresponding o-aminobenzyl alcohol derivatives 4 with phosgene or its equivalents,2 selenium-catalyzed cabonylation;2 cyclization of o-vinyl-phenyl carbamates 5 reacting with I2, Br2, HI or H2O2;3 and aminolysis of the lactone ring in 3-substituted phthalides 6 followed by Hofmann rearrangement and ring closure.4 A recently developed methodology implies double-lithiation of a transient urea derived from phenylisocyanate 7 and subsequent reaction with aldehydes to give intermediate benzhydrols which cyclize to benzoxazinones.3,5
In continuation with our studies aimed at developing new methods to synthesize heterocyclic compounds with potential biological activity, we describe herein an alternative procedure for the synthesis of the 3,1-benzoxazin-2-one core starting from 3-hydroxyoxindole derivatives. We also present the beneficial effect of microwaves on the transformations carried out in this work.
RESULTS AND DISCUSSION
It is known that methyl 2-(2-oxo-3-indolyl)acetate (8, Scheme 2) is prone to undergo acid-catalyzed ring opening alkoxylation to give dimethylsuccinate 9, which after recyclization leads to 2-quinolone 10.6 An equilibrium favoring 10 was identified6 and succinate 9 could not be isolated. It is also known that N-carbomethoxy isatylidenes, like 11, are smoothly opened to the corresponding benzylidene 12 on treatment with MeOH under reflux to give quinolinone 13 after ring reclosure.7 In addition, we recently reported the preparation of 3-hydroxyoxindole derivatives like 16a (Scheme 3) and their use for the total synthesis of natural products.8 Thus, we envisioned that acid-catalyzed ring opening of these oxindoles could afford phenylsuccinates 17, analogs of amino alcohols 4, which in turn could be used to build the 3,1-benzoxazin-2-one scaffold 18.
The general synthesis of benzoxazinones is described in Scheme 3, showing 3-hydroxyoxindoles 16 as the key intermediates for the goal which were prepared from the corresponding indoles 14. Compounds 14b and 15a have been synthesized under traditional heating,8a and in this work we explore for milder and practical reaction conditions to accelerate the rate of formation of compounds 14b8b and 15a-c by using microwave (MW) irradiation. The results are shown in Table 1, where comparisons of reaction times clearly exemplifies the advantages of heating by MW irradiation to afford the products.9 Thus, esterification of 14a to 14b and N-H protections of 14a-c to 15a-c always proceeded faster with MW-heating. In addition, oxidation of compounds 15a-c to the corresponding 3-hydoxyoxindoles 16a-c was achieved using dimethyl dioxirane (DMD).10 The X-ray structure of 16b is shown in Figure 2).
With 3-hydroxyoxindoles 16a-c in hand we focus our attention to the ring opening of compounds 16 with alcohols under reflux, giving rise to phenylsuccinates 17. The reaction of 16a with MeOH under reflux for 50 h afforded 17a in 70% yield, together with unreacted starting material. When hydrogen chloride catalyzed methoxylation of 16a was performed at rt, 17a was obtained in 86% yield after a prolonged reaction time (23 h). When the acid-catalyzed reaction was carried out under reflux for 5 h, the desired target 3,1-benzoxazin-2-one 18a (Figure 4) and phenylsuccinate 17a were obtained as major products in moderate 49% and 24% yields, respectively, together with minor amounts of quinolinone 20a in 12% yield. In contrast, MW irradiation of 16a for 1.5 h gave 17a (41%), 18a (20%) and 20a (11%). Longer reaction times to increase selective formation of 18a resulted in decomposition. These results clearly showed that 3-hydroxyoxindole 16a could be a useful starting material to produce the 3,1-benzoxazin-2-one 18a directly or in a two steps sequence through phenylsuccinate 17a.
It is worth noting that most 1H (Figure 5) and 13C NMR signals of 3-hydroxyoxindole 16a and 3,1-benzoxazin-2-one 18a show quite similar signal patterns (see experimental) and could ambiguously be assigned to either compound, although the great differences in proton chemical shifts due to the OH (in 16a), NH (in 18a), H7 (in 16a)11 and H8 (in 18a) signals as well as the specific AB pattern of the methylene protons for each isomer allow recognition of the spectrum for each isomer. The X-ray structures shown in Figures 2-4 evidence that H7, H3’ and H5 in oxindoles 16, succinates 17 and 3,1-benzoxazin-2-one 18, respectively, could be affected by the anisotropic effect of the corresponding carbonyl groups in crystals, which is in exact coincidence with those results obtained in solution11 (See experimental). Figure 3 shows succinate 17b with a disordered ethyl group.
It is important to note that hydrogen chloride acid-catalyzed transformation of 16a to 17a or 18a requires the use of freshly prepared concentrated HCl/MeOH (3.8 M) in order to avoid prolonged reaction times. Treatment of 16a with silica gel12 in refluxing MeOH, accomplished an efficient conversion (80%) in a short time (1 h) to give phenylsuccinate 17a. The MW irradiation was also efficiently achieved for this conversion since 17a was obtained in only 8 min with a similar yield (81%) (Table 2, entries 1 and 2). On ring opening of 3-hydroxyoxindole 16a to phenylsuccinate 17a either with MeOH, HCl/MeOH or silica gel/MeOH, consumption of the starting material (16a) is close to completion and 16a was always recovered. This suggested an equilibrium between 16a and 17a. In fact, when 17a was treated with silica gel/MeOH under reflux for 1.5 h, TLC monitoring (by UV) of the reaction process always showed existence of two components corresponding to the starting material 17a and 3-hydroxyoxindole 16a in a constant ratio of 8:1 (by 1H NMR).
In order to study the steric hindrance influence of the alcohol used in ring opening of 16a, this compound was treated with EtOH, i-PrOH and t-BuOH using silica gel to catalyze the reaction under reflux and under MW irradiation. As is shown in Table 2 (Entries 3-6), ring opening of 16a is greatly influenced by the steric bulkiness of the alcohol used. As the steric hindrance of the alcohol increases, conversion of 16a to 17a-c becomes considerably slower with starting material recovery and poor chemical yields. Even more, when 16a was treated with t-BuOH, the desired product 18 was not detected, instead a trace amount of oxindole 19a was obtained8a (Scheme 3) after a prolonged reaction time. Similarly, 3-hydroxyoxindoles 16b and 16c were converted to the corresponding phenylpropionates 17d and 17e in 87% and 76% yields, respectively, with MW irradiation (Table 2, entries 7-10). The synthesized phenylsuccinates 17a-d were efficiently crystallized and their crystal structures are shown in Figure 3. With these results we can conclude that ring opening of 3-hydroxyoxindoles 16a-c is steric hindrance dependent both on the alcohol and carbamate substituents. It is worth noting that the transformation of 16a-c into 17a-e is always accompanied by starting material recovery.
Once phenylsuccinates and propionates 17 were obtained, the next step was to find the best reaction conditions for benzoxazinone ring formation. Thus, when phenylsuccinate 17a was treated with methanolic hydrogen chloride at rt, 3,1-benzoxazin-2-one 18a was obtained in 70% yield but only after a prolonged reaction time (192 h) (Table 3, entry 1). The long reaction time for this transformation was drastically reduced by use of MW irradiation for 1 h, giving 18a in 54% yield together with oxindole 16a in 9% yield (Table 3, entry 2). In addition, when 17b was treated with HCl/MeOH at rt for 288 h, the expected 3,1-benzoxazin-2-one 18b was not obtained, but rather a mixture of transesterified phenylsuccinate 17a and 3,1-benzoxazin-2-one 18a was obtained in 33% and 64% yields, respectively (Table 3, entry 3). No traces of phenylsuccinate 17b nor 3,1-benzoxazin-2-one 18b were detected. This suggests that reactions from 17b to 17a or from 18b to 18a are faster than the ring closure of 17b to 3,1-benzoxazin-2-one 18b. To demonstrate this hypothesis, phenylsuccinate 17b was treated with ethanolic hydrogen chloride at rt (for 288 h) to yield a mixture of phenylsuccinate 17f (Figure 3) and 3,1-benzoxazin-2-one 18c (Figure 4) in 32% and 60% yields (Table 3, entry 4), respectively, no traces of 3,1-benzoxazin-2-one 18b or phenylsuccinate 17b being detected. These results also demonstrated that steric hindrance for ring closure of 17a or 17f to the corresponding benzoxazinones does not influence significantly the reaction rate. When 17b was reacted under the same reaction conditions using MW irradiation for 1 h, considerable enhancement was observed, giving rise to 3,1-benzoxazin-2-one 18c in 43% yield, together with oxindole 19b (19%), succinate 17f (26%), and quinolinone 20b (10%) (Table 3, entry 5).
Less hindered phenylpropionate 17d was also reacted with HCl/MeOH giving rise to 3,1-benzoxazin-2-one 18d at rt in 76% after 192 h, a similar result than that obtained when 17a was reacted under the same conditions. However, MW irradiation of 17d during 1 h gave 18d and oxindole 16b in 81% and 8% yields, respectively (Table 3, entries 8 and 9).
The steric effect influence of ring closure from succinates to the benzoxazinone scaffold was evidenced when 17c was treated with HCl/i-PrOH at rt since 3,1-benzoxazin-2-one 18e (Figure 4) was obtained in only 16% yield after 432 h, together with succinate 17g in 39% yield and oxindole 19c in 18% yield (Table 3, entry 6), evidencing a steric effect dependence around C2 in the closure of phenylsuccinate 17a-c and phenylpropionate 17d to either 3,1-benzoxazin-2-one 18 or oxindole 19. Attempting to reduce the long conversion time of 17c to 18e using MW afforded 3,1-benzoxazin-2-one 18e in 42% yield, together with phenylsuccinate 17g (17%), oxindole 19c (16%) and quinolinone 20c in 12% yield after 1 h (Table 3, entry 7). With less sterically hindered phenylsuccinates 17a-17b and phenylpropionate 17d nucleophilic attack of a OH group at C2 to form a carbonyl carbamate group is favored, giving rise to the corresponding 3,1-benzoxazin-2-ones 18a, 18b and 18d, while with increased steric effect at this position nucleophilic attack of the NH group to the –CO2R3 group is favored affording oxindoles 19b and 19c. Reaction of N-Boc sterically hindered succinate 17e with HCl/MeOH and MW irradiation conducted exclusively to oxindole 19d (Figure 2, Table 5) in 99% after 1 h (Table 3, entry 10), probably due to acid labile hydrolysis of the NHBoc group.13
Although 3,1-benzoxazin-2-one 18c and 18e could easily be obtained from their corresponding succinates 17f and 17g using MW irradiation, the yields are low due to their transformation into unidentified products. Thus we decided to transesterify 3,1-benzoxazin-2-one 18a with HCl/EtOH and HCl/i-PrOH using MW irradiation whereby 3,1-benzoxazin-2-ones 18c and 18e were obtained selectively in 95% and 91% yields, respectively (Table 3, entries 11 and 12).
In conclusion, we have demonstrated that 3-hydroxyoxindoles 16 are suitable starting materials to synthesize 3,1-benzoxazin-2-ones 18 in a two steps sequence through phenylsuccinates or phenylpropionates 17. A variety of 3,1-benzoxazin-2-ones could be obtained by transesterification of 17 or 18 with several alcohols. MW irradiation provides products in good yields and short reaction times as compared to conventional heating. In view of the ready availability of the starting materials, as well as the ease of operation, the present procedure offers a convenient synthetic methodology for this heterocycles.
EXPERIMENTAL
General experimental procedures
Melting points were determined on a Büchi B-540 apparatus. IR spectra were recorded on a Perkin-Elmer GX FT-IR spectrophotometer. The 400 and 100 MHz 1H and 13C NMR spectra were obtained on JEOL Eclipse + 400 and Varian VNMRS 400 spectrometers, while the 300 and 75 MHz 1H and 13C NMR spectra were obtained on a Varian Mercury-300 spectrometer using CDCl3 or DMSO-d6, as solvents. The chemical shifts of CHCl3 and DMSO present in the deuterated solvents were used as reference.14 For complete assignments 2D NMR, gHSQC, and gHMBC spectra were used. Data are reported as follows: chemical shift in ppm, integration, multiplicity (s = singlet, d = doublet, t = triplet, q = quartet, sp = septet, m = multiplet, br = broad, AB = AB system), coupling constant (Hz), and assignment. GC/MS analyses were conducted on a Varian CP 3800 GC equipped with a Varian Saturn 2000 selective mass detector and a 30 m, 0.25 mm i.d., 0.25 mm CP-SIL capillary column, using helium as carrier gas (1 mL/min), programmed from 70 °C to 250 °C at a rate of 30 °C/min, with the injector temperature at 200 °C. MS analyses were obtained in the electron impact (EI) mode at an ionizing voltage of 70 eV on a Hewlett Packard 5989-A spectrometer. High-resolution (HR) mass spectra were measured on a JEOL JMS-SX 102A mass spectrometer at Instituto de Química, UNAM-México. Microanalytical determinations were performed on a Perkin-Elmer 2400 series PCII apparatus. Analytical thin-layer chromatography (TLC) was done on silica gel F254 coated aluminum sheets (0.25 mm thickness) with a fluorescent indicator. Visualization was accomplished with UV light (254 nm). Flash chromatography was done using Silica Gel 60 (230-400 mesh) from Aldrich. Microwave irradiation reactions were carried out on a CEM Mars 5™ microwave reactor working at 300 watts ±15% and equipped with an ESP-1500 Plus Pressure Control System and a RTP-300 Plus Temperature Control System. The reaction mixtures were deposited in HP-500 vessels and the instrumental temperature settings were 60 °C for MeOH, 75 °C for EtOH, 85 °C for i-PrOH, 80 °C for CH3CN, and 120 °C for Me2CO3.
Preparation of methyl 2-(1H-indol-3-yl)acetate 14b.
To a solution of indolylacetic acid (14a, 6.00 g, 34.25 mmol) in MeOH (50 mL) was added p-toluenesulfonic acid (100 mg) and the solution heated under microwave irradiation for 20 min. After cooling to rt, the MeOH was evaporated under reduced pressure and the residue was dissolved in EtOAc (100 mL). The organic phase was washed with a saturated aqueous solution of NaHCO3 (2 x 30 mL) and brine (2 x 30 mL), dried over Na2SO4, filtered and evaporated to dryness in vacuum. The resultant crude product was purified by flash column chromatography on silica gel eluting with EtOAc/hexanes 1:4 to give 14b as a yellow solid (6.450 g, 99%). Spectra were consistent with those reported.8c
General procedure for the preparation of indole carbamates 15b,c.8
A solution of 3-methylindole (14c, 1.0 g, 7.62 mmol) and 1,8-diazabicyclo[5.4.0]undec-7-ene (DBU) 0.1 equiv (114 µL) in Me2CO3 (30 mL) was stirred under reflux (i) for 26 h or (ii) during 6 h under microwave irradiation. Similarly, to a solution of 14c (1.0 g, 7.62 mmol) in MeCN (25 mL) was added Boc2O (2.0 g, 1.2 equiv) and DMAP (0.093 g, 0.1 equiv) and the mixture was stirred at rt (iii) for 3 h or (iv) during 10 min under microwave irradiation. After cooling to rt, the volatiles were evaporated and EtOAc (50 mL) was added to the reaction crudes. The organic solution was washed with a saturated aqueous solution of NaHCO3 (2 × 25 mL) and brine (2 × 25 mL), dried over Na2SO4, filtered and concentrated in vacuo. The resultant crude products 15b,c were purified by flash chromatography on silica gel using EtOAc/hexanes 1:7.
Methyl 3-methyl-1H-indolecarboxylate (15b). Prepared from 14c as colorless oil:8 (i) 1.330 g, 92%; (ii) 1.320 g, 91%. Although 15b is known,15 it is spectroscopically not yet fully characterized. Thus, NMR data follow: 1H NMR (CDCl3, 400 MHz) δ 8.19 (1H, brs, H7), 7.52 (1H, dd, J = 7.1, 0.8 Hz, H4), 7.37 (1H, td, J = 7.1, 1.2 Hz, H6) and 7.37 (1H, brs, H2), 7.29 (1H, td, J = 7.4, 0.8 Hz, H5), 4.02 (3H, s, CO2Me), 2.29 (3H, d, J = 1.2 Hz, CH3); 13C NMR (CDCl3, 100 MHz) δ 151.7 (CO2Me), 135.7 (C7a), 131.7 (C3a), 124.7 (C6), 122.9 (C5), 122.6 (C2), 119.2 (C4), 117.5 (C3), 115.3 (C7), 53.8 (CO2Me), 9.9 (CH3); IR (KBr) νmax 3051, 2955, 1734, 1611 cm-1; EIMS m/z 189 [M +] (100), 144 (43), 130 (19), 103 (9), 77 (9); FABHRMS m/z 189.0790 (calcd for C11H11NO2,189.0790).
tert-Butyl 3-methyl-1H-indole-1-carboxylate (15c). Prepared from 14c as colorless oil: (iii) 1.750 g, 99%; (iv) 1.700 g, 97%. Although 15c is known,16 it is spectroscopically not yet fully characterized. Thus, NMR data follow: 1H NMR (CDCl3, 400 MHz) δ 8.11 (1H, brs, H7), 7.48 (1H, d, J = 7.8 Hz, H4), 7.34 (1H, brs, H2), 7.30 (1H, td, J = 7.3, 1.3 Hz, H6), 7.23 (1H, td, J = 7.4, 1.3 Hz, H5), 2.25 (3H, s, CH3), 1.65 (9H, s, CO2tBu); 13C NMR (CDCl3, 100 MHz) δ 148.9 (CO2tBu), 134.7 (C7a), 130.8 (C3a), 123.6 (C6), 122.2 (C2), 121.8 (C5), 118.4 (C4), 115.9 (C3), 114.7 (C7), 83.3 (CO2tBu), 29.2 (CO2tBu), 10.9 (CH3); IR (KBr) νmax 2978, 2934, 1732, 1609, 1389 cm-1; EIMS m/z 175 [M + − tBu] (100), 130 (91), 77 (14), 57 (30); FABHRMS m/z 231.1252 (calcd for C14H17NO2, 231.1259).
General procedure for the preparation of 3-hydroxyoxindoles 16b,c. To a solution of the appropriate indole 15b (0.50 g, 2.64 mmol) or 15c (0.50 g, 2.16 mmol) in acetone (15 mL) was added NaHCO3 (3.5 equiv) dissolved in water (5-7 mL). The resulting thick mixture was treated dropwise, at rt over 10 min, with a solution of oxone monopersulfate complex (2.5 equiv of KHSO5) and disodium EDTA (5 mg) in water (4-6 mL). After complete addition, the mixture was stirred at rt for additional 2 h for 15b, and 4 h for 15c. The solids were filtrated off and the acetone was evaporated under reduced pressure, and the residue was dissolved in EtOAc (30 mL). The organic solution was washed with brine (2 × 20 mL), dried over Na2SO4, filtered and concentrated in vacuo. The resultant crude products were purified by flash column chromatography with EtOAc/hexanes 1:4.
Methyl 3-hydroxy-3-methyl-2-oxoindoline-1-carboxylate (16b). Prepared from 15b as colorless crystals (0.350 g, 59%); mp 90−92 ºC (EtOAc/hexanes); 1H NMR (DMSO-d6, 400 MHz) δ 7.85 (1H, d, J = 8.3 Hz, H7), 7.49 (1H, dd, J = 7.3, 1.0 Hz, H4), 7.42 (1H, td, J = 7.8, 1.4 Hz, H6), 7.28 (1H, td, J = 7.3, 1.0 Hz, H5), 6.32 (1H, s, OH), 3.95 (3H, s, CO2Me), 1.49 (3H, s, CH3); 13C NMR (DMSO-d6, 100 MHz) δ 174.8 (C2), 150.0 (CO2Me), 137.1 (C7a), 131.6 (C3a), 128.6 (C6), 124.3 (C5), 123.1 (C4), 114.3 (C7), 72.7 (C3), 54.5 (CO2Me), 26.2 (CH3); IR (KBr) νmax 3405, 3004, 2953, 1761, 1746, 1614 cm-1; EIMS m/z 221 [M +] (21), 204 (31), 193 (58), 178 (32), 146 (100); Anal. Calcd for C11H11NO4: C 59.73; H 5.01; N 6.33. Found: C 59.73; H 4.92; N 5.98.
tert-Butyl 3-hydroxy-3-methyl-2-oxoindoline-1-carboxylate (16c). Prepared from 15c as colorless oil (0.350 g, 62%); 1H NMR (DMSO-d6, 400 MHz) δ 7.79 (1H, brd, J = 8.2 Hz, H7), 7.47 (1H, dd, J = 7.4, 1.2 Hz, H4), 7.40 (1H, td, J = 8.2, 1.2 Hz, H6), 7.25 (1H, td, J = 7.5, 1.1 Hz, H5), 6.25 (1H, s, OH), 1.61 (9H, s, CO2tBu), 1.47 (3H, s, CH3); 13C NMR (DMSO-d6, 100 MHz) δ 177.3 (C2), 149.7 (CO2tBu), 139.1 (C7a), 133.2 (C3a), 130.1 (C6), 125.6 (C5), 124.5 (C4), 115.5 (C7), 84.6 (CO2tBu), 73.1 (C3), 28.6 (CO2tBu), 25.8 (CH3); IR (film) νmax 3452, 2980, 2934, 1781, 1732, 1611, 1288, 1146 cm-1; EIMS m/z 163 (calcd for C14H17NO4, 263.1158).
Procedures for ring opening of 3-hydroxyoxindoles 16a-c.
Method A. A solution of dioxyindole 16a (0.50 g, 1.791 mmol) in MeOH (25 mL) was heated under reflux for 50 h. After cooling to rt, the solvent was evaporated under reduced pressure and the resultant crude product was purified by flash column chromatography.
Method B. A solution of dioxyindole 16a (0.10 g, 0.358 mmol) in HCl/MeOH (3.8 M, 2.5 mL) was stirred at rt for 23 h. The volatiles were evaporated under reduced pressure and the residue was diluted with EtOAc (25 mL), washed with an aqueous saturated aqueous solution of NaHCO3 (2 × 20 mL) and brine (2 × 20 mL), dried over Na2SO4, filtered and concentrated in vacuo. The resultant crude product was purified by flash column chromatography.
Method C. A solution of dioxyindole 16a (0.50 g, 1.791 mmol) in HCl/MeOH (3.8 M, 25 mL) was stirred under reflux (i) for 5 h or (ii) under microwave irradiation during 1.5 h. The volatiles were evaporated under reduced pressure and the residue was diluted with EtOAc (50 mL), washed with an aqueous saturated aqueous solution of NaHCO3 (3 × 25 mL) and brine (2 × 20 mL), dried over Na2SO4, filtered and concentrated in vacuo. The resultant crude product was purified by flash column chromatography.
Method D. To a solution of the appropriate dioxyindole 16a (0.50 g, 1.791 mmol), 16b (0.50 g, 2.260 mmol) or 16c (0.50 g, 1.899 mmol) in 25 mL of the appropriate alcohol (MeOH for 16a-c, EtOH for 16a, or i-PrOH for 16a) was added silica gel (1.0 g) and the mixture heated (i) at reflux or (ii) under microwave irradiation for a specified period of time as follows: (i) 16a and 16b when reacting with MeOH for 1 h, 16c for 3 h; 16a when reacting with EtOH for 14 h; 16a when reacting with i-PrOH for 87 h; (ii) 16a when reacting with MeOH for 8 min, 16b for 10 min and 16c for 45 min; 16a when reacting with EtOH for 7 h; 16a when reacting with i-PrOH for 21 h. After cooling to rt, the solids were filtrated, the alcohol was evaporated under reduced pressure and the residue was purified by column chromatography.
Dimethyl 2-hydroxy-2-(2-(methoxycarbonylamino)phenyl)succinate (17a). Prepared from 16a and purified with EtOAc/hexanes 2:3 to afford colorless crystals. Method A: 0.389 g, 70% and 0.138 g of recovered 16a; Method B: 0.096 g, 86% and 0.013 g of recovered 16a; Method C: (i) 0.133 g, 24% and 0.045 g of recovered 16a; (ii) 0.226 g, 41% and 0.044 g of recovered 16a; Method D: (i) 0.446 g, 80% and 0.082 g of recovered 16a; (ii) 0.449 g, 81% and 0.083 g of recovered 16a; mp 145-147 ºC (EtOAc/hexanes); 1H NMR (DMSO-d6, 400 MHz) δ 9.08 (1H, brs, NH), 7.90 (1H, brd, J = 8.0 Hz, H3’), 7.39 (1H, dd, J = 7.6, 1.1 Hz, H6’), 7.35 (1H, td, J = 7.6, 1.4 Hz, H4’), 7.11 (1H, td, J = 7.6, 1.1 Hz, H5’), 7.02 (1H, s, OH), 3.70 (3H, s, NCO2Me), 3.65 (3H, s, CO2Me), 3.64 (3H, s, CH2CO2Me), 3.56, 3.08 (2H, AB system, J = 15.0 Hz, H3); 13C NMR (DMSO-d6, 100 MHz) δ 172.9 (CO2Me), 170.6 (CH2CO2Me), 154.6 (NCO2Me), 137.9 (C2’), 130.8 (C1’), 129.7 (C4’), 127.4 (C6’), 124.1 (C5’), 122.5 (C3’), 77.4 (C2), 53.3 (CO2Me), 52.9 (CH2CO2Me), 52.5 (NCO2Me), 44.2 (C3); IR (KBr) νmax 3451, 3352, 2957, 2850, 1731, 1590, 1455 cm-1; EIMS m/z 311 [M] + (6), 279 (5), 252 (15), 220 (42), 178 (23), 146 (100), 120 (10), 90 (18), 59 (26). Anal. Calcd for C14H17NO7: C 54.02; H 5.50; N 4.50. Found: C 54.31; H 5.57; N 4.10.
Methyl 4-(2-methoxy-2-oxoethyl)-2-oxo-2,4-dihydro-1H-benzo[d][1,3]oxazine-4-carboxylate (18a). Prepared from 16a and purified with EtOAc/hexanes 2:3 to afford 18a as colorless crystals. Method C: (i) 0.243 g, 49%; (ii) 0.102 g, 20%; mp 181-183 ºC (EtOAc/hexanes); 1H NMR (DMSO-d6, 300 MHz) δ 10.43 (1H, s, NH), 7.47 (1H, dd, J = 7.7, 1.1 Hz, H5), 7.34 (1H, td, J = 7.7, 1.3 Hz, H7), 7.08 (1H, td, J = 7.7, 1.1 Hz, H6), 6.91 (1H, dd, J = 8.0, 1.1 Hz, H8), 3.92, 3.23 (2H, AB system, J = 16.8 Hz, H9), 3.68 (3H, s, CO2Me), 3.67 (3H, s, CH2CO2Me); 13C NMR (DMSO-d6, 75.4 MHz) δ 170.2 (CO2Me), 169.9 (CH2CO2Me), 149.8 (NCO2), 136.5 (C8a), 131.3 (C7), 126.2 (C5), 123.9 (C6), 118.7 (C4a), 115.3 (C8), 83.1 (C4), 54.2 (CH2CO2Me), 52.9 (CO2Me), 41.7 (C9); IR (KBr) νmax 3431, 3131, 2960, 1723, 1599, 1490 cm-1; EIMS m/z 279 [M] + (2), 220 (23), 188 (11), 146 (100); Anal. Calcd for C13H13NO6: C 55.92; H 4.69; N 5.02. Found: C 55.96; H 4.64; N 4.74.
Methyl 2-oxo-1,2-dihydroquinoline-4-carboxylate (20a). The reaction of 16a with method C also afforded quinolinone 20a which was purified with EtOAc/hexanes 2:3 to afford 20a as a white solid: (i) 0.042 g, 12%; (ii) 0.041 g, 11%; mp 246-248 ºC; 1H NMR (DMSO-d6, 400 MHz) δ 12.20 (1H, brs, NH), 8.09 (1H, dd, J = 8.2, 1.2 Hz, H5), 7.61 (1H, td, J = 8.2, 1.2 Hz, H7), 7.42 (1H, dd, J = 8.2, 0.8 Hz, H8), 7.28 (1H, td, J = 7.5, 1.2 Hz, H6), 6.94 (1H, s, H3), 3.96 (3H, s, CH3); 13C NMR (DMSO-d6, 100 MHz) δ 166.6 (CO2Me), 161.9 (C2), 141.0 (C4), 140.4 (C8a), 132.2 (C7), 126.9 (C5), 125.0 (C3), 123.5 (C6), 116.9 (C8), 116.5 (C4a), 54.0 (CH3); IR (KBr) νmax 3453, 2919, 2850, 1727, 1671 cm-1; EIMS m/z 203 [M] + (100), 173 (19), 144 (22), 117 (26).
1-Ethyl 4-methyl-2-hydroxy-2-(2-(methoxycarbonylamino)phenyl)succinate (17b). Prepared from 16a and purified with EtOAc/hexanes 2:3 to afford colorless crystals. Method D: (i) 0.405 g, 70% and 0.135 g of recovered 16a; (ii) 0.292 g, 50% and 0.222 g of recovered 16a; mp 90-92 ºC (EtOAc/hexane); 1H NMR (DMSO-d6, 400 MHz) δ 9.12 (1H, brs, NH), 7.92 (1H, brd, J = 8.0 Hz, H3’), 7.38 (1H, d, J = 7.7 Hz, H6’), 7.36 (1H, t, J = 7.3 Hz, H4’), 7.10 (1H, td, J = 7.3, 1.1 Hz, H5’), 6.99 (1H, s, OH), 4.13 (2H, dc, J = 15.0, 7.3 Hz, CO2CH2), 3.70 (3H, s, NCO2Me), 3.64 (3H, s, CO2Me), 3.57, 3.08 (2H, AB system, J = 15.0 Hz, H3), 1.15 (3H, t, J = 7.3 Hz, CH3); 13C NMR (DMSO-d6, 100 MHz) δ 172.4 (CO2CH2), 170.6 (CO2Me), 154.5 (NCO2Me), 138.0 (C2’), 130.7 (C1’), 129.7 (C4’), 127.4 (C6’), 123.9 (C5’), 122.1 (C3’), 77.4 (C2), 62.2 (CO2CH2), 52.9 (NCO2Me), 52.5 (CO2Me), 44.2 (C3), 14.7 (Et); IR (KBr) νmax 3460, 3366, 2957, 2849, 1732, 1589, 1452 cm-1; EIMS m/z 325 [M] + (4), 279 (4), 252 (16), 220 (46), 178 (28), 146 (100), 90 (16), 59 (19). Anal. Calcd for C15H19NO7: C 55.38; H 5.89; N 4.31. Found: C 55.51; H 5.88; N 3.91.
1-Isopropyl 4-methyl 2-hydroxy-2-(2-(methoxycarbonylamino)phenyl)succinate (17c). Prepared from 16a and purified with EtOAc/hexanes 2:3 to afford colorless crystals. Method D: (i) 0.085 g, 14% and 0.390 g of recovered 16a; (ii) 0.061 g, 10% and 0.414 g of recovered 16a; mp 113-115 ºC (EtOAc/hexanes); 1H NMR (DMSO-d6, 400 MHz) δ 9.17 (1H, brs, NH), 7.95 (1H, brd, J = 8.0 Hz, H3’), 7.36 (1H, d, J = 7.7 Hz, H6’), 7.35 (1H, t, J = 7.3 Hz, H4’), 7.09 (1H, td, J = 7.7, 1.1 Hz, H5’), 6.96 (1H, s, OH), 4.94 (1H, sp, J = 6.3 Hz, CH), 3.70 (3H, s, NCO2Me), 3.64 (3H, s, CO2Me), 3.57, 3.07 (2H, AB system, J = 15.0 Hz, H3), 1.18, 1.14 (6H, 2d, J = 6.3, 6.6 Hz, 2CH3); 13C NMR (DMSO-d6, 100 MHz) δ 171.8 (CO2CH), 170.6 (CO2Me), 154.4 (NCO2Me), 138.1 (C2’), 130.4 (C1’), 129.7 (C4’), 127.4 (C6’), 123.7 (C5’), 121.7 (C3’), 77.5 (C2), 69.9 (CH), 52.8 (CO2Me), 52.5 (NCO2Me), 44.2 (C3), 22.1, 22.0 (2CH3); IR (KBr) νmax 3446, 3341, 2982, 2847, 1712, 1590 cm-1; EIMS m/z 339 [M] + (4), 252 (21), 220 (44), 178 (31), 146 (100), 90 (22), 59 (20); Anal. Calcd for C16H21NO7: C 56.63; H 6.24; N 4.13. Found: C 56.90; H 6.37; N 3.94.
Methyl 2-hydroxy-2-(2-(methoxycarbonylamino)phenyl)propionate (17d). Prepared from 16b and purified with EtOAc/hexanes 1:7 to afford colorless crystals. Method D: (i) 0.484 g, 85% and 0.063 g of recovered 16b; (ii) 0.499 g, 87% and 0.051 g of recovered 16b; mp 108-110 ºC (EtOAc/hexanes); 1H NMR (DMSO-d6, 400 MHz) δ 8.86 (1H, brs, NH), 7.77 (1H, brd, J = 7.8 Hz, H3’), 7.42 (1H, dd, J = 7.8, 1.4 Hz, H6’), 7.34 (1H, td, J = 7.3, 1.4 Hz, H4’), 7.13 (1H, td, J = 7.3, 1.1 Hz, H5’), 6.84 (1H, brs, OH), 3.68 (3H, s, NCO2Me), 3.59 (3H, s, CO2Me), 1.76 (3H, s, CH3); 13C NMR (DMSO-d6, 100 MHz) δ 173.5 (CO2Me), 153.0 (NCO2Me), 136.2 (C2’), 131.9 (C1’), 128.1 (C4’), 125.8 (C6’), 123.1 (C5’), 121.4 (C3’), 75.7 (C2), 53.2 (CO2Me), 52.8 (NCO2Me), 26.9 (CH3); IR (KBr) νmax 3363, 3293, 3012, 2957, 1746, 1701, 1609 cm-1; EIMS m/z [M + − H2O] (0.1), 221 (37), 204 (100), 193 (51), 178 (27), 146 (83); Anal. Calcd for C12H15NO5: C 56.91; H 5.97; N 5.53. Found: C 56.94; H 5.89; N 5.26.
Methyl 2-(2-(tert-butoxycarbonylamino)phenyl)-2-hydroxypropionate (17e). Prepared from 16c and purified with CH2Cl2 to afford 17e as colorless crystals. Method D: (i) 0.395 g, 70% and 0.120 g of recovered 16c; (ii) 0.425 g, 76% and 0.079 g of recovered 16c; mp 128-130 ºC (EtOAc/hexanes); 1H NMR (DMSO-d6, 400 MHz) δ 8.58 (1H, brs, NH), 7.74 (1H, brd, J = 7.9 Hz, H3’), 7.40 (1H, dd, J = 7.6, 1.5 Hz, H6’), 7.32 (1H, td, J = 7.0, 1.5 Hz, H4’), 7.10 (1H, td, J = 7.6, 1.1 Hz, H5’), 6.80 (1H, brs, OH), 3.62 (3H, s, CO2Me), 1.77 (3H, s, CH3), 1.49 (9H, s, CO2tBu); 13C NMR (DMSO-d6, 100 MHz) δ 175.8 (CO2Me), 153.4 (CO2tBu), 138.0 (C2’), 133.1 (C1’), 129.2 (C4’), 127.0 (C6’), 123.9 (C5’), 122.8 (C3’), 80.2 (CO2tBu), 76.1 (C2), 53.3 (CO2Me), 29.0 (CO2tBu), 26.5 (CH3); IR (KBr) νmax 3344, 3314, 2992, 2951, 1737, 1686, 1608 cm-1; EIMS m/z 190 [M + − O-tBu + MeOH)] (0.1), 163 (30), 135 (93), 120 (100), 92 (19); FABHRMS m/z 295.1428 (calcd for C15H21NO5, 295.1420).
Cyclization procedure for succinates and propionates 17a-e.
A solution of the appropriate succinate 17a (0.250 g, 0.803 mmol), 17b (0.250 g, 0.768 mmol), 17c (0.250 g, 0.737 mmol) or propionate 17d (0.250 g, 0.987 mmol), 17e (0.250 g, 0.846 mmol) in the appropriate HCl/alcohol mixture (50 mL) was stirred at (i) rt or (ii) under microwave irradiation for a specified period of time as follows: (i) 17a and 17d when reacting with HCl/MeOH for 192 h, 17b for 288 h, 17e for 1 h; 17b when reacting with HCl/EtOH for 288 h; 17c when reacting with HCl/i-PrOH for 432 h; (ii) 17a and 17d when reacting with HCl/MeOH for 1 h, 17b when reacting with HCl/EtOH for 1 h; 17c when reacting with HCl/i-PrOH for 1 h. The volatiles were evaporated under reduce pressure and the residue was diluted with EtOAc (30-50 mL), washed with aqueous saturated aqueous solution of NaHCO3 (3 × 25 mL) and brine (2 × 20 mL), dried over Na2SO4, filtered and concentrated in vacuo. The resultant crude product was purified by flash column chromatography. Cyclization of 17a afforded 16a and 18a, cyclization of 17b afforded 18c, 17f, 19b and 20b, cyclization of 17c afforded 18e, 17g, 19c and 20c, cyclization of 17d afforded 16b and 18d, while cyclization of 17e afforded 19d.
Methyl 3-hydroxy-3-(2-methoxy-2-oxoethyl)-2-oxoindoline-1-carboxylate 16a. Prepared from 17a and purified with EtOAc/hexanes 2:3 to afford colorless crystals (ii) 0.020 g, 9%; mp 132-133 ºC (EtOAc/hexanes), Lit.,8 132-133 °C.
Methyl 4-(2-methoxy-2-oxoethyl)-2-oxo-2,4-dihydro-1H-benzo[d][1,3]oxazine-4-carboxylate (18a). Prepared from 17a and purified with EtOAc/hexanes 2:3 to afford colorless crystals (i) 0.156 g, 70% and 0.072 g of recovered 17a; (ii) 0.120 g, 54% and 0.090 g of recovered 17a.
Ethyl 4-(2-ethoxy-2-oxoethyl)-2-oxo-2,4-dihydro-1H-benzo[d][1,3]oxazine-4-carboxylate (18c). Prepared from 17b and purified with EtOAc/hexanes 2:3 to afford colorless crystals (i) 0.141 g, 60%; (ii) 0.102 g, 43%; mp 132-134 ºC (EtOAc/hexanes); 1H NMR (DMSO-d6, 400 MHz) δ 10.43 (1H, brs, NH), 7.45 (1H, d, J = 6.9 Hz, H5), 7.33 (1H, td, J = 7.8, 1.4 Hz, H7), 7.07 (1H, td, J = 7.8, 1.4 Hz, H6), 6.90 (1H, dd, J = 7.8, 0.9 Hz, H8), 4.20-4.04 (4H, m, 2OCH2), 3.88, 3.17 (2H, AB system, J = 16.6 Hz, H9), 1.20, 1.13 (6H, 2t, J = 7.4, 7.3 Hz, 2CH3); 13C NMR (DMSO-d6, 100 MHz) δ 167.3, 167.0 (CO2CH2, CH2CO2CH2), 148.0 (C2); 134.7 (C8a), 129.7 (C7), 124.6 (C5), 122.4 (C6), 117.4 (C4a), 113.9 (C8), 82.4 (C4), 62.6, 61.2 (2OCH2), 42.0 (C9), 15.4, 15.2 (2CH3); IR (KBr) νmax 3235, 3104, 2982, 1749, 1560 cm-1; EIMS m/z 307 [M] + (0.4), 234 (88), 146 (100). Anal. Calcd for C15H17NO6: C 58.63; H 5.58; N 4.56. Found: C 58.63; H 5.54; N 4.27.
Diethyl 2-hydroxy-2-(2-(methoxycarbonylamino)phenyl)succinate (17f). Prepared from 17b and purified with EtOAc/hexanes 2:3 to afford colorless crystals: (i) 0.084 g, 32%; (ii) 0.068 g, 26%; mp 73-75 ºC (EtOAc/hexanes); 1H NMR (DMSO-d6, 400 MHz) δ 9.12 (1H, brs, NH), 7.92 (1H, brd, J = 7.8 Hz, H3’), 7.38 (1H, dd, J = 7.8, 1.5 Hz, H6’), 7.35 (1H, td, J = 8.3, 1.5 Hz, H4’), 7.10 (1H, td, J = 7.8, 1.5 Hz, H5’), 6.99 (1H, brs, OH), 4.19-4.04 (4H, m, 2OCH2), 3.70 (3H, s, NCO2Me), 3.56, 3.04 (2H, AB system, J = 14.9 Hz, H9), 1.21, 1.15 (6H, 2t, J = 7.3 Hz, 2CH3); 13C NMR (DMSO-d6, 100 MHz) δ 172.4 (CO2CH2), 170.2 (CH2CO2CH2), 154.6 (NCO2Me), 138.1 (C2’), 130.7 (C1’), 129.8 (C4’), 127.5 (C6’), 124.0 (C5’), 122.1 (C3’), 77.5 (C2), 62.2, 61.3 (2OCH2), 52.9 (NCO2Me), 44.5 (C3), 14.9, 14.7 (2CH3); IR (KBr) νmax 3480, 3373, 3099, 2985, 1736, 1590, 1456 cm-1; EIMS m/z 293 [M] + − EtOH] (15), 265 (16), 219 (70), 146 (100). Anal. Calcd for C16H21NO7: C 56.63; H 6.24; N 4.13. Found: C 56.76; H 6.19; N 3.82.
Methyl 3-(2-ethoxy-2-oxoethyl)-3-hydroxy-2-oxoindoline-1-carboxylate (19b). Prepared from 17b and purified with EtOAc/hexanes 2:3 to afford a white solid: (ii) 0.043 g, 19%; mp 66-68 ºC; 1H NMR (DMSO-d6, 400 MHz) δ 7.85 (1H, brd, J = 7.9 Hz, H7), 7.53 (1H, dd, J = 7.5, 0.9 Hz, H4), 7.44 (1H, td, J = 7.5, 1.4 Hz, H6), 7.26 (1H, td, J = 7.5, 0.9 Hz, H5), 6.68 (1H, s, OH), 3.96 (3H, s, NCO2Me), 3.85, 3.80 (2H, 2dc, J = 10.6, 7.1 Hz, CO2CH2CH3), 3.19 (2H, s, H8), 0.93 (3H, t, J = 7.1 Hz, CO2CH2CH3); 13C NMR (DMSO-d6, 100 MHz) δ 175.9 (C2), 169.7 (CO2CH2CH3), 152.1 (NCO2Me), 140.5 (C7a), 131.0 (C6), 130.7 (C3a), 125.9 (C5), 125.1 (C4), 115.6 (C7), 73.3 (C3), 61.4 (CO2CH2CH3), 54.9 (NCO2Me), 43.3 (C8), 14.6 (CO2CH2CH3); IR (KBr) νmax 3433, 2995, 2962, 1737, 1713, 1609 cm-1; EIMS m/z 293 [M] + (15), 265 (16), 219 (75), 146 (100). FABHRMS m/z 293.0902 (calcd for C14H15NO6, 293.0899).
Ethyl 2-oxo-1,2-dihydroquinoline-4-carboxylate (20b). Prepared from 17b and purified with EtOAc/hexanes 2:3 to afford a white solid: (ii) 0.017 g, 10%; mp 208-210 ºC; 1H NMR (DMSO-d6, 400 MHz) δ 12.18 (1H, brs, NH), 8.09 (1H, dd, J = 8.2, 1.2 Hz, H5), 7.61 (1H, td, J = 7.1, 1.2 Hz, H7), 7.42 (1H, dd, J = 7.4, 0.8 Hz, H8), 7.28 (1H, td, J = 7.0, 1.2 Hz, H6), 6.94 (1H, s, H3), 4.43 (2H, q, J = 7.0 Hz, CH2), 1.39 (3H, t, J = 7.0 Hz, CH3); 13C NMR (DMSO-d6, 100 MHz) δ 166.0 (CO2Et), 161.8 (C2), 141.2 (C4), 140.4 (C8a), 132.1 (C7), 126.8 (C5), 124.8 (C3), 123.4 (C6), 116.8 (C8), 116.4 (C4a), 62.9 (CH2), 14.9 (CH3); IR (KBr) νmax 3454, 2923, 2851, 1724, 1655 cm-1; EIMS m/z 217 [M] + (100), 189 (45), 172 (20), 161 (14), 144 (31), 116 (48); FABHRMS m/z 217.0737 (calcd for C12H11NO3, 217.0739).
Isopropyl 4-(2-isopropoxy-2-oxoethyl)-2-oxo-2,4-dihydro-1H-benzo[d][1,3]oxazine-4-carboxylate (18e). Prepared from 17c and purified with EtOAc/hexanes 2:3 to afford colorless crystals (i) 0.040 g, 16% and 0.060 g of recovered 17c; (ii) 0.105 g, 42% and 0.020 g of recovered 17c; mp 131-133 ºC (EtOAc/hexanes); 1H NMR (DMSO-d6, 400 MHz) δ 10.40 (1H, brs, NH), 7.43 (1H, brd, J = 6.7 Hz, H5), 7.33 (1H, td, J = 7.8, 1.2 Hz, H7), 7.07 (1H, td, J = 7.9, 1.2 Hz, H6), 6.89 (1H, dd, J = 7.8, 1.2 Hz, H8), 4.95, 4.88 (2H, 2 sp, J = 6.2, 6.3 Hz, 2CH), 3.81, 3.12 (2H, AB system, J = 16.5 Hz, H9), 1.21, 1.19, 1.18, 1.08 (12H, 4d, J = 6.3 Hz, 4CH3); 13C NMR (DMSO-d6, 100 MHz) δ 169.0 (CO2CH), 168.7 (CH2CO2CH), 149.9 (C2), 136.4 (C8a), 131.1 (C7), 126.1 (C5), 123.7 (C6), 118.9 (C4a), 115.1 (C8), 83.2 (C4), 70.7, 69.0 (2CH), 42.2 (C9), 22.5, 22.4, 22.1, 22.0 (4CH3); IR (KBr) νmax 3168, 2986, 2941, 1741, 1603 cm-1; EIMS m/z 292 [M +− iPr] (2), 248 (23), 234 (15), 206 (59), 146 (100).
Diisopropyl 2-hydroxy-2-(2-(methoxycarbonylamino)phenyl)succinate (17g). Prepared from 17c and purified with EtOAc/hexanes 2:3 to afford a colorless oil: (i) 0.106 g, 39%; (ii) 0.045 g, 17%; 1H NMR (DMSO-d6, 400 MHz) δ 9.15 (1H, brs, NH), 7.94 (1H, brd, J = 7.8 Hz, H3’), 7.35 (1H, d, J = 7.8 Hz, H6’), 7.34 (1H, t, J = 6.8 Hz, H4’), 7.08 (1H, td, J = 7.8, 1.0 Hz, H5’), 6.87 (1H, brs, OH), 4.94 (1H, sp, J = 6.3 Hz, CH2CO2CH), 4.92 (1H, sp, J = 6.3 Hz, CO2CH), 3.70 (3H, s, NCO2Me), 3.50, 3.00 (2H, AB system, J = 14.9 Hz, H3), 1.22 (6H, d, J = 6.3 Hz, 2CH3), 1.17, 1.11 (6H, 2d, J = 6.3 Hz, 2CH3); 13C NMR (DMSO-d6, 100 MHz) δ 169.3 (CO2CH), 167.2 (CH2CO2CH), 152.3 (NCO2Me), 136.3 (C2’), 128.8 (C1’), 127.9 (C4’), 125.7 (C6’), 122.2 (C5’), 120.2 (C3’), 76.8 (C2), 69.2 (CO2CH), 68.1 (CH2CO2CH), 52.5 (NCO2Me), 44.4 (C3), 22.8, 22.7, 22.4, 22.3 (4CH3); IR (KBr) νmax 3363, 2982, 2939, 1736, 1591, 153, 1067 cm-1; EIMS m/z (relative intensity) 307 [M +− iPrOH] (13), 279 (23), 265 (27), 219 (81), 205 (66), 146 (100); FABHRMS m/z 367.1635 (calcd for C18H25NO7, 367.1631).
Methyl 3-hydroxy-3-(2-isopropoxy-2-oxoethyl)-2-oxoindoline-1-carboxylate (19c). Prepared from 17c and purified with EtOAc/hexanes 2:3 to afford a white solid: (i) 0.041 g, 18%; (ii) 0.036 g, 16%; mp 76-78 ºC; 1H NMR (DMSO-d6, 400 MHz) δ 7.86 (1H, brd, J = 8.2 Hz, H7), 7.53 (1H, dd, J = 7.5, 0.8 Hz, H4), 7.44 (1H, td, J = 7.9, 1.2 Hz, H6), 7.26 (1H, td, J = 7.4, 1.2 Hz, H5), 6.66 (1H, s, OH), 4.60 (1H, sp, J = 6.3 Hz, CH), 3.97 (3H, s, NCO2Me), 3.17, 3.11 (2H, AB system, J = 15.8, H8), 0.91, 0.85 (6H, 2d, J = 6.3 Hz, 2CH3); 13C NMR (DMSO-d6, 100 MHz) δ 175.8 (C2), 168.8 (CO2CH), 152.1 (NCO2Me), 140.5 (C7a), 130.9 (C6), 130.6 (C3a), 125.8 (C5), 125.0 (C4), 115.6 (C7), 73.3 (C3), 68.7 (CH), 54.8 (NCO2Me), 43.7 (C8), 22.1, 21.8 (2CH3); IR (KBr) νmax 3464, 2971, 2928, 1794, 1727, 1611cm-1; EIMS m/z 307 [M] + (17), 266 (31), 220 (66), 205 (85), 146 (100); FABHRMS m/z 307.1057 (calcd for C15H17NO6, 307.1056).
Isopropyl 2-oxo-1,2-dihydroquinoline-4-carboxylate (20c). Prepared from 17c and purified with EtOAc/hexanes 2:3 to afford a white solid: (ii) 0.021 g, 12%; mp 186-188 ºC; 1H NMR (DMSO-d6, 400 MHz) δ 12.17 (1H, brs, NH), 8.06 (1H, dd, J = 8.2, 1.1 Hz, H5), 7.59 (1H, td, J = 7.1, 1.1 Hz, H7), 7.41 (1H, dd, J = 8.2, 1.2 Hz, H8), 7.27 (1H, td, J = 8.2, 1.2 Hz, H6), 6.90 (1H, s, H3), 5.25 (1H, sp, J = 6.3 Hz, CH), 1.39 (6H, d, J = 6.2 Hz, 2CH3); 13C NMR (DMSO-d6, 100 MHz) δ 165.5 (CO2i-Pr), 161.8 (C2), 141.5 (C4), 140.4 (C8a), 132.0 (C7), 126.7 (C5), 124.6 (C3), 123.4 (C6), 116.9 (C8), 116.4 (C4a), 70.7 (CH), 22.4 (2CH3); IR (KBr) νmax 3418, 2923, 2850, 1712, 1662 cm-1; EIMS m/z 231 [M] + (57), 189 (100), 172 (14), 161 (20), 144 (21), 117 (36); FABHRMS m/z 231.0887 (calcd for C13H13NO3, 231.0895).
Methyl 3-hydroxy-3-methyl-2-oxoindoline-1-carboxylate (16b). Prepared from 17d and purified with EtOAc/hexanes 1:4 to afford colorless crystals (ii) 0.017 g (8%).
Methyl 4-methyl-2-oxo-2,4-dihydro-1H-benzo[d][1,3]oxazine-4-carboxylate (18d). Prepared from 17d and purified with EtOAc/hexanes 1:4 to afford colorless crystals: (i) 0.167 g, 76% and 0.046 g of recovered 17d; (ii) 0.176 g, 81% and 0.018 g of recovered 17d; mp 164-166 ºC (EtOAc/hexanes); 1H NMR (DMSO-d6, 400 MHz) δ 10.38 (1H, s, NH), 7.42 (1H, dd, J = 7.8, 1.0 Hz, H5), 7.34 (1H, td, J = 7.8, 1.4 Hz, H7), 7.10 (1H, td, J = 7.8, 1.0 Hz, H6), 6.92 (1H, dd, J = 7.8, 1.0 Hz, H8), 3.68 (3H, s, CO2Me), 1.93 (3H, s, CH3); 13C NMR (DMSO-d6, 100 MHz) δ 169.1 (CO2Me), 148.6 (C2), 134.7 (C8a), 129.3 (C7), 124.5 (C5), 122.4 (C6), 119.6 (C4a), 113.7 (C8), 82.1 (C4), 53.9 (CO2Me), 24.1 (CH3); IR (KBr) νmax 3419, 3166, 2925, 1742, 1601, 1497 cm-1; EIMS m/z 221 [M] + (3), 162 (100), 144 (23), 116 (27); Anal. Calcd for C11H11NO4: C 59.73; H 5.01; N 6.33. Found: C 59.76; H 4.88; N 5.94.
3-Hydroxy-3-methylindolin-2-one (19d). Prepared from 17e and purified with EtOAc/hexanes 2:3 to afford colorless crystals: (i) 0.137 g, 99%; mp 163-165 ºC (EtOAc/hexanes); 1H NMR (DMSO-d6, 400 MHz) δ 10.27 (1H, brs, NH), 7.32 (1H, dd, J = 6.7, 0.7 Hz, H4), 7.23 (1H, td, J = 7.4, 1.2 Hz, H6), 7.00 (1H, td, J = 7.5, 1.2 Hz, H5), 6.85 (1H, d, J = 7.4 Hz, H7), 5.91 (1H, s, OH), 1.40 (3H, s, CH3); 13C NMR (DMSO-d6, 100 MHz) δ 180.7 (C2), 142.1 (C7a), 134.6 (C3a), 129.8 (C6), 124.4 (C4), 122.7 (C5), 110.6 (C7), 73.6 (C3), 25.5 (CH3); IR (KBr) νmax 3369, 3181, 2975, 1709, 1626 cm-1; EMIE m/z 163 [M] + (18), 135 (73), 120 (100), 92 (21); FABHRMS m/z 163.0632 (calcd for C9H9NO2, 163.0633).
General transesterification procedure for benzoxazinone 18a to 18c,e.
A solution of benzoxazinone 18a (0.05 g, 0.179 mmol) in the appropriate mixture HCl/EtOH or HCl/i-PrOH (20 mL) was heated under microwave irradiation to afford 18c for 1 h and to afford 18e for 2.5 h. After cooling to rt, the volatiles were evaporated under reduced pressure and the residue was dissolved in EtOAc (20 mL). The organic solution was washed with a saturated aqueous solution of NaHCO3 (3 x 20 mL) and brine (2 x 20 mL), dried over Na2SO4, filtrated and evaporated to dryness in vacuum. The resultant crude products were purified by flash column chromatography on silica gel eluting with EtOAc/hexanes, 2:3 to afford, respectively, 18c and 18e in 95% (0.052 g) and 92% (0.055 g) yield.
Single crystal X-ray diffraction analyses
Data collection for 17a (Table 4) was carried out on a Bruker Smart 6000 CCD diffractometer using Mo/Kα radiation (λ = 0.7073 Å). A total of 1321 frames were collected at a scan width of 0.3° and an exposure time of 10 s/frame. These data were processed with the SAINT software package, provided by the diffractometer manufacturer, by using a narrow frame integration algorithm. An empirical absorption correction was applied. Data collections for 16b, 17b-17f, 18a, 18c and 18d (Tables 4 and 5) were done on an Enraf-Nonius CAD4 diffractometer using Cu Kα radiation (λ = 1.54184 Å) while those for 18e and 19d were done on an Agilent Technologies Gemini A CCD diffractometer using Mo/Kα radiation (λ = 0.7073 Å). The structures were solved by direct methods using the SHELXS-9717 program included in the WINGX v1.6 package.18 Structural refinements were carried out by full-matrix least squares on F2. The
non-hydrogen atoms were treated anisotropically, and the hydrogen atoms, included in the structure factor calculation, were refined isotropically. Atomic coordinates, bond lengths, bond angles and anisotropic thermal parameters are in deposit at the Cambridge Crystallographic Data Center. Tables 4 and 5 summarize the relevant data.
ACKNOWLEDGEMENTS
We are pleased to acknowledge financial support from CONACYT (Mexico) grants 83723 and 84453. CIBH thanks CONACYT for fellowships 237689.
References
1. (a) R. C. Rizzo, M. Udier-Blagović, D.-P. Wang, E. K. Watkins, M. B. Kroeger Smith, R. H. Smith Jr., J. Tirado-Rives, and W. L. Jorgensen, J. Med. Chem., 2002, 45, 2970; CrossRef (b) P. Zhang, E. A. Terefenko, A. Fensome, J. Wrobel, R. Winneker, S. Lundeen, K. B. Marschke, and Z. Zhang, J. Med. Chem., 2002, 45, 4379; CrossRef (c) P. Zhang, J. C. Kern, E. A. Terefenko, A. Fensome, R. Unwalla, Z. Zhang, J. Cohen, T. J. Berrodin, M. R. Yudt, R. C. Winneker, and J. Wrobel, Bioorg. Med. Chem., 2008, 16, 6589. CrossRef
2. (a) S. S. Nikam, P.-W. Yuen, B. E. Kornberg, B. Tobias, and M. F. Rafferty, J. Org. Chem., 1997, 62, 9331; CrossRef (b) R. Consonni, P. D. Croce, R. Ferraccioli, and C. La Rosa, J. Chem. Soc., Perkin Trans. 1, 1996, 1809; CrossRef (c) M. Patel, S. S. Ko, R. J. McHugh Jr., J. A. Markwalder, A. S. Srivastava, B. C. Cordova, R. M. Klabe, S. Erickson-Viitanen, G. L. Trainor, and S. P. Seitz, Bioorg. Med. Chem. Lett., 1999, 9, 2805; CrossRef (d) M. Patel, R. J. McHugh Jr., B. C. Cordova, R. M. Klabe, S. Erickson-Viitanen, G. L. Trainor, and S. S. Ko, Bioorg. Med. Chem. Lett., 1999, 9, 3221; CrossRef (e) J. C. Kern, E. A. Terefenko, A. Fensome, R. Unwalla, J. Wrobel, Y. Zhu, J. Cohen, R. Winneker, Z. Zhang, and P. Zhang, Bioorg. Med. Chem. Lett., 2007, 17, 189; CrossRef (f) M. Ohno, H. Sato, and S. Eguchi, Synlett, 1999, 207; CrossRef (g) T. Yoshida, N. Kambe, S. Murai, and N. Sonoda, Bull. Chem. Soc. Jpn., 1987, 60, 1793. CrossRef
3. (a) K. Kobayashi, S. Fukamachi, and H. Konishi, Heterocycles, 2008, 75, 2301; CrossRef (b) K. Kobayashi, S. Fukamachi, D. Nakamura, O. Morikawa, and H. Konishi, Heterocycles, 2008, 75, 95. CrossRef
4. E. Hernández, J. M. Vélez, and C. P. Vlaar, Tetrahedron Lett., 2007, 48, 8972. CrossRef
5. C. E. Houlden, G. C. Lloyd-Jones, and K. I. Booker-Milburn, Org. Lett., 2010, 12, 3090. CrossRef
6. E. Rivera-Becerril, N. Pérez-Hernández, P. Joseph-Nathan, and M. S. Morales-Ríos, Heterocycles, 2006, 68, 1459. CrossRef
7. M. S. Morales-Ríos, N. X. Martínez-Galero, M. Loeza-Coria, and P. Joseph-Nathan, J. Org. Chem., 1995, 60, 6194. CrossRef
8. (a) O. R. Suárez-Castillo, M. Sánchez-Zavala, M. Meléndez-Rodríguez, L. E. Castelán-Duarte, M. S. Morales-Ríos, and P. Joseph-Nathan, Tetrahedron, 2006, 62, 3040; CrossRef (b) Q. Yang, X.-j. Wang, Z.-y. Li, L. Sun, and Q.-d. You, Synth. Commun., 2008, 38, 4107; CrossRef (c) M. Selva, P. Tundo, D. Brunelli, and A. Perosa, Green Chem., 2007, 9, 463. CrossRef
9. (a) D. Obermayer, B. Gutmann, and C. O. Kappe, Angew. Chem. Int. Ed., 2009, 48, 8321; CrossRef (b) M. Burns, G. Lloyd-Jones, J. D. Moseley, and J. S. Renny, J. Org. Chem., 2010, 75, 6347; CrossRef (c) J. Robinson, S. Kingman, D. Irvine, P. Licence, A. Smith, G. Dimitrakis, D. Obermayer, and C. O. Kappe, Phys. Chem. Chem. Phys., 2010, 12, 4750. CrossRef
10. L. Shu and Y. Shi, J. Org. Chem., 2000, 65, 8807. CrossRef
11. O. R. Suárez-Castillo, Y. M. A. Contreras-Martínez, L. Beiza-Granados, M. Meléndez-Rodríguez, J. R. Villagómez-Ibarra, J. M. Torres-Valencia, M. S. Morales-Ríos, and P. Joseph-Nathan, Tetrahedron, 2005, 61, 8809. CrossRef
12. T. Apelqvist and D. Wensbo, Tetrahedron Lett., 1996, 37, 1471. CrossRef
13. T. W. Green and P. G. M. Wuts, ‘Protective Groups in Organic Synthesis,’ 3rd ed. by Wiley: New York, 1999.
14. (a) H. E. Gottlieb, V. Kotlyar, and A. Nudelman, J. Org. Chem., 1997, 62, 7512; CrossRef (b) G. R. Fulmer, A. J. M. Miller, N. H. Sherden, H. E. Gottlieb, A. Nudelman, B. M. Stoltz, J. E. Bercaw, and K. I. Goldber, Organometallics, 2010, 29, 2176. CrossRef
15. E. Wenkert, M. E. Alonso, H. E. Gottlieb, and E. L. Sanchez, J. Org. Chem., 1977, 42, 3945. CrossRef
16. (a) L. Shen and R. P. Hsung, Org. Lett., 2005, 7, 775; CrossRef (b) R. Kuwano and M. Kashiwabara, Org. Lett., 2006, 8, 2653. CrossRef
17. G. M. Sheldrick, ‘Programs for Crystal Structure Analysis.’ Institüt für Anorganische Chemie der Universität; University of Göttingen: Göttingen, Germany, 1988.
18. L. J. Farrugia, J. Appl. Crystallogr., 1999, 32, 837. CrossRef