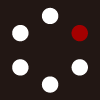
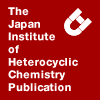
HETEROCYCLES
An International Journal for Reviews and Communications in Heterocyclic ChemistryWeb Edition ISSN: 1881-0942
Published online by The Japan Institute of Heterocyclic Chemistry
e-Journal
Full Text HTML
Received, 9th April, 2012, Accepted, 26th June, 2012, Published online, 4th July, 2012.
DOI: 10.3987/REV-12-741
■ Development of the Acenaphthenequinone Reactions
Ghodsi Mohammadi Ziarani,* Parvin Hajiabbasi, and Parisa Gholamzadeh
Department of Chemistry, Alzahra University, Vanak Square, Tehran, P.O. Box number 19938939973, Iran
Abstract
Acenaphthenequinone and its derivatives have a broad spectrum of applications as biologically active compounds, dyes, pharmaceuticals, drugs, therapeutic agents and pesticides. This brief review represents various reactions such as pericyclic, multicomponent, ring opening and substitutions of acenaphthenequinone reactions.CONTENTS
1 Introduction
2 Pericyclic reactions of acenaphthenequinone
2.1 [4+2] And [2+2] cycloaddition of acenaphthenequinone
2.2 1,3-Dipolar cycloaddition of acenaphthenequinone
2.2.1 1,3-Dipolar cycloaddition of acenaphthenequinone via azomethine yilides
3 Ring opening of acenaphthenequinone
4 Multicomponent reaction of acenaphthenequinone
4.1 Two-component reaction of acenaphthenequinone
4.2 Three-component reaction of acenaphthenequinone
4.3 Four-component reaction of acenaphthenequinone
5 Substitution reactions of acenaphthenequinone
6 Conclusion
7 Acknowledgements
8 References and notes
1. INTRODUCTION
In the last decade, great attention has been paid to acenaphthenequinone in various reactions such as pericyclic, multicomponent and substitution reactions to attain useful products. Pericyclic reaction of acenaphthenequinone 1 can construct spirolactones 2 which are novel spiroheterocycles.1 Consequently acenaphthenequinone 1 and its derivatives are used as biologically active compounds, dyes, pharmaceuticals, drugs, therapeutic agents and pesticides.2-4 So, it has a broad spectrum of applications5 which impelled us to review their new reactions.
2. PERICYCLIC REACTIONS OF ACENAPHTHENEQUINONE
2.1 [4+2] AND [2+2] CYCLOADDITION OF ACENAPHTHENEQUINONE
Lichtenthaler et al. achieved distinctly different regioselectivities of photocycloadditions of phenanthrenequinone 5 and acenaphthenequinone 1 to 3,4,6-tri-Ο-acetyl-D-glucal 3. The [2+2] cycloadduct (4, 86%) was attained by photocycloadditions of acenaphthenequinone 1 to 3,4,6-tri-Ο-acetyl-D-glucal 3 (Scheme 1). In addition, [4+2] cycloadduct (9, 50%) was accomplish by photocycloadditions of phenanthrenequinone 5 to the analogous of 3,4,6-tri-Ο-acetyl-D-glucal 3 such as 6, 7 and 8 (Scheme 2).6
Enantioselective [4+2] annulations of 2-alkynylbenzaldehydes 10 and acenaphthenequinone 1 was proceeded using cationic rhodium (I)/ (R,R)-Walphos complexes to compose spirocyclic benzopyranones 11-13 (Scheme 3). A number of cationic rhodium(I)/chiral bisphosphine complexes were screened due to their chemo- and enantioselectivity ability for cross [4+2] annulation. Ultimately, products were prepared with high yield and good enantioselectivity using (R,R)-Walphos as a chiral ligand.7
Photoreactions of 1,2-diketones 14 and silylketene acetals 15 are projected to follow three major competitive pathways leading from the excited states involving: (1) Direct [4+2]-cycloaddition to produce dioxenes 16, (2) Classical Paterno-Büchi [2+2]-cycloaddition to construct oxetanes 17, and (3) Desilylation to generate β-hydroxy-γ-ketoesters 18 or oxetanes 17 (Scheme 4). These competitive routes leading to these products are influenced by solvent polarity and the character of the silylketene acetal 15 and 1,2-diketone 14.8
2.2 1, 3-DIPOLAR CYCLOADDITION OF ACENAPHTHENEQUINONE
Carbonyl ylides underwent 1,3-dipolar cycloaddition reaction with acenaphthenequinone 1 to synthesize novel spirooxabicycles 20, 21 (Scheme 5). These reactions afforded a mixture of stereoisomers.9
Treatment of imidoyl chlorides 22 with triethylamine gave nitrile ylides 23 which undergo dipolar cycloaddition with acenaphthenequinone 1 to form novel spirooxazoline derivatives 24, 25 in high yield (Scheme 6).10
Acenaphthenequinone 1 reacted via 1,3-dipolar cycloaddition with diazofluorene 26 to give new oxadiazole derivatives 27 which reacted with triphenylphosphine and triethyl phosphite to afford new organophosphorus compounds (Scheme 7).11
1,3-Dipolar cycloaddition of zwitterionic intermediate generated in situ from dimethyl acetylenedicarboxylate (DMAD) 28 and cyclohexyl isocyanide 29 to acenaphthenequinone 1 gave iminolactone 30 which easily hydrolysed to spirolactones (Scheme 8).12
Pyridoquinoline and particularly oxazinoquinoline derivative 32 could be achieved via 1,4-dipolar cycloaddition reaction of acenaphthenequinone 1 and intermediate 33. Dipolarophile 33 are attained via quinoline 31 and DMAD 28 (Scheme 9).13 These novel functionalized heterocyclic compounds are the central core of biologically active molecules. For example pyridoquinoline derivatives are used as potential inhibitors of the fluoroquinolone efflux pump.14
2.2.1 1, 3-DIPOLAR CYCLOADDITION OF ACENAPHTHENEQUINONE VIA AZOMETHINE YLIDES
A facile 1,3-dipolar cycloaddition one-pot synthesis of novel steroidal dispiropyrrolidines 36 had performed by the reaction of steroidal dipolarophiles 34 and azomethine ylides 38 which derived from the reaction of acenaphthenequinone 1 and sarcosine 35, under reflux in methanol for 6 h (Scheme 10). However, this reaction was carried out in different solvents such as toluene, MeCN and MeOH. The results were confirmed that in polar solvents, the reaction rate and the cycloadducts yield were good (60–72%).15 The azomethine ylide 38 approaches to the prochiral carbon from the β-face that is the least hindered side of the steroidal dipolarophile to afford compound 36 with high selectivity (Scheme 11).
A chemo-, regio- and stereoselective synthesis of the novel spiropyridopyrrolizines and -pyrrolidines 40-42 was achieved via 1,3-dipolar cycloaddition of azomethine ylides 43-45 derived from acenaphthenequinone 1 and amino acids such as proline, phenylglycine, and sarcosine 35 to a series of 1-methyl-3,5-bis[(E)-arylmethylidene]tetrahydro-4(1H)-pyridinones 39 (Scheme 12). These novel compounds 40-42, achieved in methanol by refluxing for 30 min, were exhibited significant antimycobacterial activities.16
Another regioselective synthesis of the spiropyrrolidines and spiropyrrolizines (dispiroheterocyclic compounds) was achieved via 1,3-dipolar cycloaddition of azomethine ylides 46, derived from decarboxylated sarcosine 35 and acenaphthenequinone 1, into the (E)-3-arylidene-4-chromanones 47 as dipolarophiles to afford dispiroheterocycles 48 in good yields (Scheme 13).17
A facile one-pot synthesis of novel dispiroheterocycles, dispirooxindolopyrrolidines and -pyrrolizidines 52-53, was performed via [3+2] cycloaddition reaction of azomethine ylides 43 generated by acenaphthenequinone 1 and L-proline 51 or sarcosine 35 with (E)-2-oxoindolino-3-ylidene-acetophenones 50 in good yields through three process: (1) Ground in a pestle with Ball clay and subjected to microwave irradiation (2) Reaction mixture and ZrOCl2•8H2O in methanol was refluxed (3) Reaction mixture and ZrOCl2•8H2O was ground in a pestle and subjected to microwave irradiation (Scheme 14).18 Also, novel bioactive dispiroheterocycles 56 have been achieved in another regio- and stereoselective reaction by solid-supported catalyst in good yields (80-95%).19 Azomethine ylide 55 derived from the decarboxylative route of tetrahydroisoquinoline-3-carboxylic acid 54 and acenaphthenequinone 1 to various unusual dipolarophiles such as (E)-2-oxoindolino-3-ylidene-acetophenones 50 have been accomplished under various conditions: (1) silica/MW; (2) BiCl3–silica/MW; (3) TiO2–silica/MW (Scheme 14).
An efficient and regioselective three-component 1,3-dipolar cycloaddition reaction for the synthesis of spiropyrrolidines 58 and spirothiapyrrolizidines 59 in refluxing ethanol was reported by Liu and co-workers (Scheme 15).20
As demonstrated in Scheme 16, a high regio and stereoselective synthesis of novel spiropyrrolidines and polycyclic heterocycles 61 was performed via [3+2] cycloaddition reaction of azomethine ylides generated by the decarboxylative condensation of diketone 1 and sarcosine 35 with Baylis–Hillman adducts 60 as dipolarophiles. Various solvents were utilized such as DMF, xylene and methanol. When the reaction was carried out in methanol, there was a remarkable enhancing in the yield and reducing in the reaction time.21
3. RING OPENING OF ACENAPHTHENEQUINONE
A facile rearrangement of 2-hydroxyacenaphthenone derivatives 62 occurred by the attack of nonnucleophilic bases such as the bicyclic tertiary amidine, DBU, as catalyst to yield the anlargement of ring 64. The plausible mechanism was demonstrated in Scheme 17.22
A novel methodology was achieved by C-insertion of triphenylbismuthonium 2-oxoalkylides 65 between two carbonyl functions of acenaphthenequinone 1 to form 2-acyl-3-hydroxyphenalenones 67 in good yield (Scheme 18). In this protocol t-BuOK was added to a stirred suspension salt of 65 in THF at -78 ºC. Then, acenaphthenequinone 1 was added after 10 min and the resulting mixture was allowed slowly to warm to room temperature. The earlier route involved the ozonolytic cleavage of the olefinic bond in 1,2-disubstituted acenaphthene, followed by intramolecular Claisen condensation of the consequential carbonyl compound.23
Acenaphthenequinone 1 reacted with an excess of pyrrole 68 to produce the β-unsubstituted dipyrromethane moiety in compound 69 (Scheme 19). Ultimately, the compound 70 was obtained by ring opening and oxidation of compound 69.24
Some years later, Harmjanz et al. afforded a new methodology for the preparation of porphodimethenes 72, 73 (Scheme 20). 5-Substituted, β-unsubstituted dipyrromethanes 71 reacted with acenaphthenequinone 1 in the presence of catalytic amounts of either BF3•OEt2 or TFA to form porphodimethenes 72, 73.25 Aromatic porphyrin macrocycle 74, 75 was generated by broking a carbon-carbon bond in each of the spiro-linked acenaphthenone moieties 72, 73. Ring opening of respective porphodimethenes 72, 73 (syn and anti) was performed by refluxing in THF in the presence of 30% KOH.
Reactions of acenaphthenequinone 1 and ammonium acetate led to the ring opening of acenaphthenequinone (Scheme 21).26 Acenaphthenequinone, ammonium acetate, and acetic acid were heated at reflux for 2.5 h to produce 2H-isoimidazole 76. Rearrangement of intermediate 76 occurred to produce product 77.
4. MULTICOMPONENT REACTIONS OF ACENAPHTHENEQUINONE
4.1 TWO-COMPONENT REACTION OF ACENAPHTHENEQUINONE
A novel, one-pot, green method catalyzed by triethylamine was reported in aqueous media for the synthesis of functionalized spirooxindoles 79 by the aldol reaction of vinyl malononitriles 78 as vinylogous nucleophiles and dione (Scheme 22).27
The known dye biacenaphthylidenedione 83 was achieved by deoxygenation of acenaphthenequinone 1 with hexaethylphosphorous triamide. First, dipolar intermediate 80 attained via nucleophilic attack of P(NEt2)3 on the carbonyl carbon atom of acenaphthenequinone to transform into structure 81 possessing a P-O-C bond. Then elimination of hexaethylphosphoric triamide from compound 81 offered carbene species 82 which underwent dimerization to yield the known dye biacenaphthylidenedione 83 (Scheme 23).28 These compounds 83 were synthesized previously by treatment of acenaphthenequinone 1 with PCl5 in the presence of Na2S in boiling ethanol.29
4.2 THREE-COMPONENT REACTION OF ACENAPHTHENEQUINONE
A facile one-pot three-component reaction of acenaphthoquinone 1, an activated methylene reagent 85, and 1,3-dicarbonyl compounds 84 for the synthesis of a series of spirochromene derivatives 86 catalyzed by ammonium chloride in water was reported by Dabiri and co-workers (Scheme 24).30
Acenaphthequinone 1, malononitrile/ethyl cyanoacetate 85 and various reagents including cyclic CH-acids 87 react to form new spiroacenaphthylene derivatives 88 via a facile and efficient one-pot three component synthesis (Scheme 25).31 Also, the similar multicomponent cascade-reaction was performed by heating acenaphthenequinone 1, cyclic CH-acids 87, and malononitrile 85 at 80 ºC in water without any catalyst to form spiroacenaphthylene heterocycles in 90-95% yields. The former reaction was completed in 4-8 hour but the latter was performed in 15 min.32
Acenaphthoquinone 1, various benzyls 89 and ammonium acetate 90 reacted under solvent-free conditions by heating. Thus, 9,10-diaryl-7H-benzo[d,e]imidazo[2,1-a]isoquinolin-7-ones 91 was synthesized in good to excellent yields (Scheme 26).33
Three-component synthesis of spiro[indoline-3,4′-pyrazolo[3,4-b]quinoline]dione 94, 95 was reported by Chen and co-workers via a one-pot reaction of acenaphthenequinone 1, 5-amino-3-methylpyrazole 92, and 1,3-dicarbonyl compounds 84, 93 in aqueous medium using ceric ammonium nitrate (CAN) as catalyst (Scheme 27).34 Reaction mixture was stirred at 80 ºC for 6-12 h to produce desired product in good yield.
Multicomponent condensation reaction of 4-hydroxycoumarin 97 and malononitrile 85 with acenaphthenequinone 1 catalyzed by alum (KAl(SO4)2•12 H2O) afforded novel mono- and bis(spiro-2-amino-4H-pyrans) (Scheme 28).35
An efficient, simple, and catalyst-free three-component reaction of 1,3-indandione 101, amino uracils 102 and isatins or acenaphthylene-1,2-dione 1 is reported by Imani Shakibaei and co-workers in refluxing ethanol (Scheme 29). Spiroacenaphthylene-1,4′-indeno-1,5′-pyrido[2,3-d]pyrimidines 103 was prepared in good yields (78-89%).36
A multicomponent reactions involving sarcosine 35, isatin 104 and acenaphthenequinone 1 acquiescent novel spsiropyrrolidine derivatives 105, 106 are reported by Nair and co-workers (Scheme 30). A reaction of sarcosine 35 and excess acenaphthenequinone 1 in aqueous MeOH at 90 ºC proceeded to afford a mixture of two products 105 and 106. The mechanism of reaction was demonstrated in Scheme 31.37
4.3 FOUR-COMPONENT REACTION OF ACENAPHTHENEQUINONE
Spiro[acenaphthylene-diindenopyridine]triones 108 was obtained via a one-pot, pseudo four-component synthesis of 1,3-indandione 101, aromatic amines 107, acenaphthylene-1,2-dione 1 and p-TSA by using a ‘Grindstone Chemistry’ in 3–4 min (Scheme 32).38 Also, another procedure was reported to produce spiro[acenaphthylene-diindenopyridine]-triones with higher yields from 1,3-indandione 101, aromatic amines 107, acenaphthylene-1,2-dione 1 and p-TSA by Ghahremanzadeh and co-workers. This protocol offered higher yield of products by refluxing in acetonitrile for 1 h.39
The one-pot synthesis of spiro[indoline/acenaphthylene-3,4´-pyrazolo[3,4-b]pyridine derivatives 112 were achieved by the four-component domino reactions of phenylhydrazine 109, 3-aminocrotononitrile 110, acenaphthylene-1,2-dione 1, and cyclic 1,3-dicarbonyl compounds 111, including cyclohexane-1,3-diones, barbituric acid, and 2-thioxodihydropyrimidine-4,6(1H,5H)-dione, in the presence of (±)-camphor-sulfonic acid (CSA) in aqueous medium by heating under reflux at 100 ºC for 2-3 h (Scheme 33).40
Although dispiropyrrolidines 115 was synthesized by a tandem Knoevenagel–1,3-dipolar cycloaddition reaction sequence (Method A), a highly flexible, rapid, mild, but effective extension procedure for a catalyst-free, four-component, one-pot reaction sequence of isatin 117 or acenaphthylene-1,2-dione 1, sarcosine 35, 1,3-indanedione 101, and aldehyde 113, without the isolation of dipolarophiles was reported by Ming Li and co-workers (Method B). In this reaction 1,3-dipole azomethine ylide and dipolarophile was generated in situ (Scheme 34).41 Such a strategy would afford access to a fast, one-pot synthesis of dispiroheterocycles 115, which are otherwise accessible merely through multistep synthesis (Method A).
Functionalized 3-spiropyrrolidine oxindoles and 3-spiropyrrolizidine oxindoles particularly 3-spiropyrrolidine acenaphthenones 119 was afforded by a regioselective one-pot four-component [3+2] cycloaddition reaction of 3-(cyanoacetyl)indoles 118, aldehydes 113, isatin/acenaphthylene-1,2-dione 1 and amino acid 35. The reaction of azomethine ylides (produced by the reaction of 1 and 35) with a novel dipolarophiles produced in situ through the rapid and convenient Knoevenagel condensation between 3-(cyanoacetyl)indoles 118 and aldehydes 113 was performed in this protocol in MeOH at reflux for several hours (Scheme 35).42
5. SUBSTITUTION REACTIONS OF ACENAPHTHENEQUINONE
Synthesis, structure and anion binding of a new acenaphthenequinone-based calix[4]pyrrole 123 have been studied.43 A novel calix[4]pyrrole type anion receptor containing two anti-symmetrically meso-situated acenaphthenequinone moieties has been achieved by a one-pot facile four component synthesis (Scheme 36).
Harrison et al. reported coloured compounds 125 production from adding concentrated sulphuric acid dropwise during 8 h to a stirred mixture of thiophene 124, acenaphthene-1,2-dione 1 and acetic acid. In this report thiophene 124 and acenaphthenequinone 1 react in acidic solution to construct a red compound 125 (Scheme 37).44
As demonstrated in scheme 38 an efficient synthesis of symmetrical 2,2-bis(1H-indol-3-yl)-2H-acenaphthen-1-one 127 was achieved in high yields by electron-donating substitution of indole 126 and acenaphthenequinone 1 under grinding for 30-60 min at room temperature catalyzed by recyclable solid superacid SO42-/TiO2.45
The reaction of acenaphthenequinone 1 with phenacyl bromide 128 was found to be fast under ultrasonication condition. This reaction performed in the presence of sodium iodide and indium under ultrasonication condition to give α-hydroxy keto compounds 129 in very high yield (Scheme 39).46
The synthesis of 8- and 9-fluorobenzo[k]fluoranthenes 132, 133 have been organized by Wittig reaction of the 1,2-bis[(triphenylphosphonio)methyl] salt of the proper fluorobenzene 131 with acenaphthenequinone 1 under phase-transfer conditions. The phosphonium salts are active both as reagents and as phase-transfer catalysts for the two-phase reaction with lithium hydroxide. The yields for synthesis were low (2% for 132 and 15% for 133), but the reactions can be performed on large scale with only minimum byproduct formation. These compounds 132 and 133 are active as tumorigenic agents (Scheme 40).47
A novel solvent-assisted domino Michael-aldol reaction of acenaphthenequinone 1 with acetophenones 134 was reported in methanol in the presence of KOH by heating at about 60 ºC for 12 h to afford dispiro compounds 138 (Scheme 41). Acenaphthenone-2-ylidene-ketone 135 was achieved from the condensation between acenaphthenequinone 1 and acetophenone 134, which undergoes Michael-type addition with a molecule of methanol to give the intermediate 136. Michael addition of 136 to another molecule of 135 pursued by cyclisation formed 138. Thus, the mechanism involved catalysed aldol condensation, dehydration, solvent addition, and Michael addition reactions respectively.48
6. CONCLUSION
In conclusion, acenaphthenequinone could provide versatile examples of heterocycles prevalently found in a wide variety of compounds known to exhibit potential biological properties. Important advances in the synthesis from acenaphthenequinone are summarized in detail, with classification in to four types: (a) pericyclic, (b) ring opening, (c) multicomponent, (d) Substitutions of acenaphthenequinone. Also development of the acenaphthenequinone reactions is to be continued.
7. ACKNOWLEGEMENTS
We gratefully acknowledge for financial support of Alzahra university Research Council.
References
1. A. Dömling, E. Herdtweck, and S. Heck, Tetrahedron Lett., 2006, 47, 1745. CrossRef
2. J. L. Hyatt, R. M. Wadkins, L. Tsurkan, L. D. Hicks, M. J. Hatfield, C. C. Edwards, C. R. Ross II, S. A. Cantalupo, G. Crundwell, M. K. Danks, R. K. Guy, and P. M. Potter, J. Med. Chem., 2007, 50, 5727. CrossRef
3. M. C. Rodriguez-Argüelles, M. B. Ferrari, G. G. Fava, C. Pelizzi, G. Pelosi, R. Albertini, A. Bonati, P. P. Dallaglio, P. Lunghi, and S. Pinelli, J. Inorg. Biochem., 1997, 66, 7. CrossRef
4. Y. S. El-Alawi, B. J. M. Conkey, D. G. Dixon, and B. M. Greenberg, Ecotoxicol. Environ. Saf., 2002, 51, 12. CrossRef
5. E. S. H. E. Ashry, H. A. Hamid, A. A. Kassem, and M. Shoukry, Molecules, 2002, 7, 155. CrossRef
6. F. W. Lichtenthaler, T. Weimer, and S. Immel, Tetrahedron: Asymmetry, 2004, 15, 2703. CrossRef
7. D. Hojo, K. Noguchi, M. Hirano, and K. Tanaka, Angew. Chem. Int. Ed., 2008, 47, 5820. CrossRef
8. W. C. Dae, H. Y. Lee, W. O. Sun, H. C. Jung, J. P. Hea, P. S. Mariano, and C. Y. Ung, J. Org. Chem., 2008, 73, 4539. CrossRef
9. V. Nair, K. C. Sheela, D. Sethumadhavan, R. Dhanya, and N. P. Rath, Tetrahedron, 2002, 58, 4171. CrossRef
10. V. Nair, D. Sethumadhavan, S. M. Nair, S. Viji, and N. P. Rath, Tetrahedron, 2002, 58, 3003. CrossRef
11. E. A. El-Sawi, T. B. Mostafa, and H. A. Radwan, Chem. Heterocycl. Compd., 2009, 45, 981. CrossRef
12. V. Nair, A. U. Vinod, N. Abhilash, R. S. Menon, V. Santhi, R. L. Varma, S. Viji, S. Mathew, and R. Srinivas, Tetrahedron, 2003, 59, 10279. CrossRef
13. V. Nair, S. Devipriya, and E. Suresh, Tetrahedron, 2008, 64, 3567. CrossRef
14. J. Chevalier, S. Atifi, A. Eyraud, A. Mahamoud, J. Barbe, and J. M. Page`s, J. Med. Chem., 2001, 44, 4023. CrossRef
15. A. R. S. Babu and R. Raghunathan, Tetrahedron Lett., 2008, 49, 4618. CrossRef
16. R. Ranjith Kumar, S. Perumal, S. C. Manju, P. Bhatt, P. Yogeeswari, and D. Sriram, Bioorg. Med. Chem. Lett., 2009, 19, 3461. CrossRef
17. T. Augustine, C. C. Kanakam, S. M. Vithiya, and V. Ramkumar, Tetrahedron Lett., 2009, 50, 5906. CrossRef
18. A. R. S. Babu and R. Raghunathan, Tetrahedron Lett., 2007, 48, 305. CrossRef
19. A. R. S. Babu and R. Raghunathan, Tetrahedron, 2007, 63, 8010. CrossRef
20. H. Liu, G. Dou, and D. Shi, J. Comb. Chem., 2010, 12, 633. CrossRef
21. J. Jayashankaran, R. D. R. S. Manian, M. Sivaguru, and R. Raghunathan, Tetrahedron Lett., 2006, 47, 5535. CrossRef
22. A. R. Miller, J. Org. Chem., 1979, 44, 1931. CrossRef
23. M. M. Rahman, Y. Matano, and H. Suzuki, Synthesis, 1999, 395. CrossRef
24. C. K. Chang and M. P. Kondylis, J. Chem. Soc., Chem. Commun., 1986, 316. CrossRef
25. M. Harmjanz, H. S. Gill, and M. J. Scott, J. Org. Chem., 2001, 66, 5374. CrossRef
26. D. M. White, J. Org. Chem., 1970, 35, 2452. CrossRef
27. T. H. Babu, K. Karthik, and P. T. Perumal, Synlett, 2010, 1128. CrossRef
28. A. V. Bogdanov, V. F. Mironov, B. I. Buzykin, and A. I. Konovalov, Russ. J. Gen. Chem., 2005, 75, 825. CrossRef
29. G. Mehta, P. V. V. S. Sarma, R. Uma, S. Pogodin, S. Cohen, and I. Agranat, J. Chem. Soc., Perkin Trans. 1, 1999, 13, 1871. CrossRef
30. M. Dabiri, M. Bahramnejad, and M. Baghbanzadeh, Tetrahedron, 2009, 65, 9443. CrossRef
31. M. N. Elinson, A. I. Ilovaisky, V. M. Merkulova, P. A. Belyakov, F. Barba, and B. Batanero, Tetrahedron, 2012, 68, 5833. CrossRef
32. M. Saeedi, M. M. Heravi, Y. S. Beheshtiha, and H. A. Oskooie, Tetrahedron, 2010, 66, 5345. CrossRef
33. M. Adib, B. Mohammadi, S. Ansari, H. R. Bijanzadeh, and L. G. Zhu, Tetrahedron Lett., 2011, 52, 2299. CrossRef
34. H. Chen and D. Shi, J. Comb. Chem., 2010, 12, 571. CrossRef
35. A. R. Karimi and F. Sedaghatpour, Synthesis, 2010, 1731. CrossRef
36. G. Imani Shakibaei, A. Feiz, H. Reza Khavasi, A. Abolhasani Soorki, and A. Bazgir, ACS Comb. Sci., 2011, 13, 96.
37. V. Nair, K. C. Sheela, N. P. Rath, and G. K. Eigendorf, Tetrahedron Lett., 2000, 41, 6217. CrossRef
38. R. Ghahremanzadeh, S. Ahadi, G. I. Shakibaei, and A. Bazgir, Tetrahedron Lett., 2010, 51, 499. CrossRef
39. R. Ghahremanzadeh, G. I. Shakibaei, S. Ahadi, and A. Bazgir, J. Comb. Chem., 2010, 12, 191. CrossRef
40. K. Balamurugan, S. Perumal, and J. C. Menéndez, Tetrahedron, 2011, 67, 3201. CrossRef
41. M. Li, W. L. Yang, L. R. Wen, and F. Q. Li, Eur. J. Org. Chem., 2008, 2751. CrossRef
42. K. Zhao, S. L. Zhu, D. Q. Shi, X. P. Xu, and S. J. Ji, Synthesis, 2010, 1793. CrossRef
43. W. Yang, Z. Yin, Z. Li, J. He, and J. P. Cheng, J. Mol. Struct., 2008, 889, 279. CrossRef
44. W. T. A. Harrison, B. J. Morrison, and O. C. Musgrave, Tetrahedron, 2004, 60, 9255. CrossRef
45. G. L. Feng, Chin. Chem. Lett., 2010, 21, 1057. CrossRef
46. V. Nair, C. N. Jayan, and S. Ros, Tetrahedron, 2001, 57, 9453. CrossRef
47. J. E. Rice, A. Czech, N. Hussain, and E. J. LaVoie, J. Org. Chem., 1988, 53, 1775. CrossRef
48. J. J. Vadakkan, V. Raman, N. B. Fernandez, S. Prathapan, and B. Jose, New J. Chem., 2003, 27, 239. CrossRef